INTRODUCTION
Howler monkeys show high plasticity to adapt to changes in the structure and composition of the vegetation (Chaves and Bicca-Marques, 2013). This has been attributed to their anatomy and digestive physiology, which is based on cecal and colonic fermentation of fibers (Milton, 1987). Although their diet is based on leaves, they have also been recorded eating fruits, flowers, lianas, and pods (Aristizabal, 2013; Chaves and Bicca-Marques, 2013; Aristizabal et al., 2017).
Herbivores lack enzymes to digest plant fibers, though they have maintained a symbiosis with intestinal microbiota that can cleave the fiber through digestive fermentation (Stevens and Hume, 1998). The activity of the intestinal microbiota makes many nutrients available, and in turn, degrades harmful compounds. Nonetheless, the composition interactions and dynamics of the microbial community influence the nutritional and health status of the host (Sanz et al., 2004; Yildirim et al., 2010; Lozupone et al., 2012; Amato et al., 2015).
Gastrointestinal bacteria interact and depend on each other, being metabolically structured as groups of different bacteria whose relative abundance is regulated by environmental factors and the structure and function of the intestinal epithelium (Apajalahti, 2005). Bacterial communities with high species diversity are less susceptible to invasions by bacteria pathogens, having smaller populations that use the resources more efficiently (Lozupone et al., 2012). On the contrary, less diverse microbial communities have large population sizes, since they spend fewer resources due to the lower competition (Alcock et al., 2014).
Diet changes can alter the composition of the intestinal microbiota in the long term; for example, in lowland gorillas, the abundance of Firmicutes is lower during the low fruit season, whereas Bacteroidetes tend to increase (Gomez et al., 2016). In humans, Bacteroides is associated with high animal protein and fat diets, while Prevotella is associated with diets rich in carbohydrates and simple sugars, like fruits (Wu et al., 2011). Over short periods, changes are influenced by the balance in the content of carbohydrates, fat, and dietary fiber, which also alters the structure of the bacterial community (Lozupone et al., 2012). In nonhuman primates, the adaptation of a host to a specific diet results in the adaptation and evolution of microbial species in their gastrointestinal system, and conversely, the microbiome influences the host evolution and the diversity of their diet (Yildirim et al., 2010; Gomez et al., 2016; Stumpf et al., 2016).
Both captivity and habitat degradation have been linked to decreases in microbial richness and diversity in Alouatta pigra, with captive individuals showing lower species richness (less than six times) than individuals living in the conserved habitat (Amato et al., 2013). In this study, we evaluate the diversity of the rectal microbiota of A. pigra inhabiting two neighboring areas with different physiography and different plant composition within a highly fragmented landscape. We also compare the rectal microbiota diversity found in both wild and captive monkeys. We hypothesize that the small-scale changes in plant composition between vegetation fragments alter monkeys' food availability, which makes their gut microbiota adapt to local conditions. Furthermore, the adaptability of captive individuals to a different diet produces changes in bacterial diversity or gastrointestinal dysbiosis caused by different nutritional regimens.
MATERIAL AND METHODS
Study area
The study area included flooded and non-flooded areas from a highly fragmented landscape in the municipality of Balancán, Tabasco, Mexico («17°48' N, 91°32' W, 30 m. a. s. l.; INEGI, 2008) (Fig. 1). During the 1970s through the 1990s the original flood, plain forests underwent a severe degradation, losing approximately 80 % of their original cover, resulting in a vegetation patchwork of crops and cattle ranches (Pozo-Montuy and Serio-Silva, 2007). The vegetation of the two areas had different structures and compositions. The flooded area is dominated by Sabal mexicana, but there also are Pithecellobium lanceolatum, Enterolobium cyclocarpum, Maclura tinctoria, Guazuma ulmifolia, Tabebuia rosea, and Coccoloba barbadensis. The non-flooded area is dominated by Guazuma ulmifolia, and there also are S. mexicana, Haematoxilum campechianum, Lonchocarpus guatemalensis, and E. cyclocarpum. The representative vegetation was 80 % of the vegetation composition of each area (Pozo-Montuy and Serio-Silva, 2007).
We also obtained samples from captive howler monkeys which were fed with Leaf Eater Biscuit (Mazuri Primate Leaf Eater Diet, PMI Nutrition International), baby cereals (wheat, oats, soy, barley) (Nestle Company), yogurt, and leafy greens such as lettuce, spinach, celery, alfalfa, and some fruits, such as banana, melon, papaya, and orange.
Study subjects and capture technique
Between November 2008 and June 2009, we captured 15 individuals: five from a flooded area, and 10 from a non-flooded area (Fig. 1). To cover a larger area, we selected one individual of each troop due to the semi-closed social structure of howler monkeys (Van Belle et al., 2006). A certified veterinarian team captured apparently healthy individuals by chemical restraint using ketamine hydrochloride IM (Anesket, Pisa, Mexico; 10-25 mg/kg; Hall et al., 2001) or with tiletamine-zolazepam IM (Zoletil 50, Virbac, France; 15 mg/kg; Ancrenaz et al., 2003) to promote muscular relaxation when the howlers were in tallest trees. The anesthetics were injected intramuscularly by darting (3 ml plastic darts with needle 1.2 x 38 mm) with a CO2 rifle. Once each monkey was immobilized, the samples were collected. After sampling, each monkey was introduced to a wire mesh cage until its recovery, which took between 1 to 2 hours. Subsequently, the individuals were released near their groups.
We also sampled five captive howler monkeys from the Chapultepec Zoo in Mexico City. The sampling technique was performed during the annual health check exam, under light sedation with ketamine hydrochloride IM. The sampling method and sample handling were the same as described above. All restraint and management procedures will be following the Guidelines for use of wild mammal species in research of the American Society of Mammalogists (Sikes et al., 2016). The study was approved with the permit issued by the Mexican government agency (SEMARNAT; SGPA/ DGVS/05938/08), and by the Animal Care Committee of INECOL.
Collection and handling of samples
Once the animals were immobilized, everyone was subjected to a complete physical exam. Later four rectal swabs were collected from everyone after cleaning the perineal area with benzalkonium chloride; two swabs were immediately transferred to Cary-Blair transport medium (COPAN Diagnostics Inc., Murrieta, CA, USA) and maintained at 4 °C until further processing in the laboratory; the other two swabs were transported in anaerobic jars.
After transportation and the arrival of the samples to the laboratory, bacterial isolation and identification were performed at the Conservation Medicine Laboratory of the Superior Medicine School, National Polytechnic Institute (Instituto Politécnico Nacional, IPN, at Mexico City). Bacteria were isolated by the culture of swabs on rich media (Blood agar; Buxton, 2005) and serial dilution under aerobic and anaerobic conditions. Each bacterial isolate was presumptively identified based on morphophenotypic characteristics such as Gram staining, microscopic morphology, growth in selective and differential media (e.g. tetrathionate broth, MacConkey agar, brilliant green agar and Salmonella-Shigella agar, alkaline peptone water, TCBS or thiosulfate-citrate-bile salts-sucrose agar); the bacterial species were assigned by mean of the percentage of similitude (PS) of each isolate based in the database of Bergey's Manual of Systematic Bacteriology (Holt et al., 1994).
Data analysis
A presence-absence data matrix was built for everyone, with information on the presence and absence of each bacterial morphospecies. Alpha diversity was calculated per habitat condition by morphospecies richness (S), Simpson Dominance index (DS), and Margalef diversity index (DMg) (Magurran, 2004). Margalef index allows obtaining information about biodiversity based on the number of morphospecies present but not in their abundance. Simpson index (D) was calculated to estimate alpha diversity considering the species richness and evenness, where D increases with richness assuming that all morphospecies are distributed between all individuals. Also, the Shannon-Wiener index (H) was calculated supposing that all isolated bacterial morphospecies are represented and all individuals (identified bacteria) were distributed randomly (Magurran, 2004); Shannon-Wiener indexes were compared by a paired t-test. The diversity estimators are provided with lower and upper values. We also performed a dominance/diversity plot (Whittaker plots) to compare the shapes (evenness) of the curves, and the sequence of each morphospecies that compose the bacterial community in each condition (Magurran, 2004). Finally, we compared the microbial community diversity (beta diversity) with principal coordinates analysis (PCoA) (Lozupone et al., 2011) to evaluate the distance metric within them, considering the individuals as a parcel within the habitat condition. In the same way, we performed an analysis of similarity (ANOSIM) with Bray-Curtis indexes and 9999 permutations to evaluate the similarity among three communities (McGarigal et al., 2000) . All analyses and diversity estimators were performed by PAST version 3.06 statistical software (Hammer et al., 2001) using a significance level of 0.05.
RESULTS
The culture and isolation of rectal microbiota under the specified growth conditions showed growth of 62 different morphotype bacteria (in 32 to 35 genera, 20 Families, six Classes, and four Phyla), most of them with species identity similitude values above 70 %. Proteobacteria was the most prevalent phylum in howler monkeys from the non-flooded area (47.54 %), followed by Firmicutes (22.95 %), Actinobacteria (19.67 %), and Bacteroidetes (9.84 %). Although most Firmicutes bacteria cannot grow under aerobic conditions, we were able to isolate some bacterial species. Howlers in the flooded environment showed that Firmicutes and Proteobacteria phyla had the same prevalence (42.11 % each one), followed by Bacteroidetes (10.53 %), and Actinobacteria (5.26 %). Instead, captive howlers had a similar prevalence as the howlers in the flooded area regarding Firmicutes and Proteobacteria phyla, but prevalence values opposites in Bacteroidetes (5.26 %) and Actinobacteria (10.53 %). The Firmicutes/ Bacteroidetes ratio in captive howlers was higher than in wild howlers in flooded and non-flooded areas (8, 4, and 2.33, respectively).
Despite the difference between the number of samples per habitat, the howler monkeys from non-flooded habitat had a higher richness of bacterial types (S = 45), higher Margalef's diversity value (DMg = 10.66), Simpson's diversity index (D = 0.968), and lower dominance (DS = 0.032) (Table 1) . This dominance is marked by Myroides, Chryseobacterium indologenes, followed by Micrococcus sp. and Escherichia coli (Fig. 2) . Gut microbiota of captive monkeys had low bacteria diversity (S = 18), but no significant differences were found regarding non-flooded habitat monkeys. Its alfa diversity consisted of a lower Margalef's diversity (DMg = 5.675) and Simpson's diversity index (D = 0.940), and higher dominance (DS = 0.060)(Table 1) of Streptococcus vestibularis and E. coli (Fig. 2). Monkeys from flooded area maintained by middle dominance (DS = 0.053), Margalef's diversity (DMg = 6.113) and Simpson's diversity index (D = 0.947); the equitability were complete (J = 1) (Table 1; Fig. 2).
Comparison of the Shannon diversities between the two samples, using a t-test showed highly significant differences between the microbiota of animals from non-flooded and flooded conditions (p = 0.0006), and non-flooded and captive conditions (p = 0.0002) (Table 1). Some bacterial morphospecies were specific to habitat conditions. The abundance of bacterial morphospecies in the microbiota community of howlers inhabiting non-flooded habitats had less equitability than other conditions (Fig. 2). In these habitat conditions the relative abundance (RA) of Myroides/Chryseobacterium indologenes was higher (RA = 0.50; n = 5) followed by Escherichia coli and Micrococcus sp. relative abundances (RA = 0.40; n = 2, for each one). Nonetheless, the microbiota community of captive howlers monkeys was constituted of19 bacterial morphospecies, being dominated by Streptococcus vestibularis and E. coli (RA = 0.40; n = 2, for each one). In flooded conditions, there was no dominance for any bacterial morphospecies as the microbiota was constituted of18 morphospecies with an evenness value and each one with an equitability value of 1(Table 1).
Table 1 Number of bacterial morphospecies and diversity indexes in samples of animals from three habitats; comparison between Shannon-Wiener indexes and analysis of similarity (ANOSIM) with Bray-Curtis indexes.
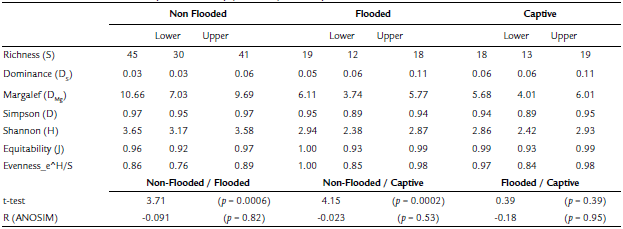
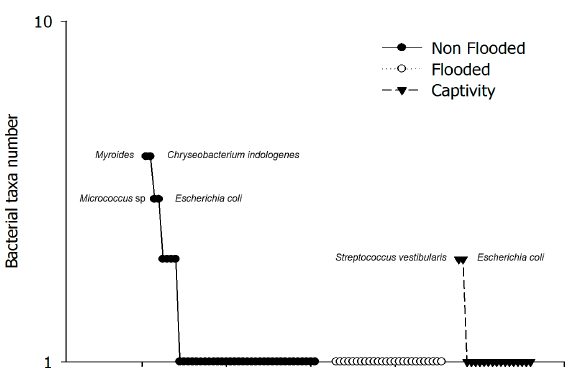
Figure 2 Dominance/diversity plot of gut bacterial morphospecies of wild howler monkeys from three habitats.
The comparison between microbial community diversity (beta diversity) with PCoA (Fig. 3) and ANOSIM (R = -0.07, p = 0.81) did not allow the differentiation of the three groups of the habitat conditions. According to the Shannon index t-test, the same outcome was obtained with ANOSIM pairwise comparison, which showed that the intestinal microbial community of the flooded habitat had more similitude with captive howler monkeys than those inhabiting non-flooded habitats (Table 1).
DISCUSSION
The rectal microbiota genus diversity in wild black howler monkeys from this study was higher than in other reports with the same species of primate (Amato et al., 2013). However, in our study, the isolation of bacterial morphospecies may not reflect the real diversity of the gut microbiota community (Frank and Pace, 2008). It is not ideal to compare the results of several studies since methods are very different: DNA sequencing has fewer biases compared to culturing since many gut microbes will not survive on common agar or in aerobic conditions. In our case, the growth of bacteria occurred according to the sampling conditions (as aseptic as possible and maintaining anaerobic conditions in the field), the transport medium, and isolation techniques. The lack of specific isolation requirements results in a difficult culture and taxonomic identification (Frank and Pace, 2008). Nevertheless, the identification of bacteria is more successful by the traditional method of diagnosis (isolation, biochemical proofs, and other phenotypic criteria) (Mühulhauser and Rivas, 2014) than molecular techniques. Molecular diagnosis needs a prior library of specific sequences for bacterial identification, which is not available for many bacterial species, whereby some studies identify only to the level of Family or genus (see Amato et al., 2013). However, the specific bacterial community may be underestimated due to the sampling and bacterial isolation methods (Budding et al., 2014), for the sampling of a single individual of each social group as a representative of the bacterial community of all individuals, as well as the quality of the food intake (Lozupone et al., 2012; Aristizabal, 2013; Budding et al., 2014). The change in the intake or differential intake could structure the intestinal microbial community of the host as an evolutionary adaptation to the quality and type of diet structure (Mariat et al., 2009; Yildirim et al., 2010; Wu et al., 2011; Lozupone et al., 2012). These changes in food intake can be explained by temporal availability (seasonal changes) or natural or induced changes in its composition (Yildirim et al., 2010; Amato et al., 2013, 2015). This is the case of changes related to land use, where fragmentation and habitat loss influence the composition of microbial communities of primate species, including the genus Alouatta (Amato et al., 2013, 2015).
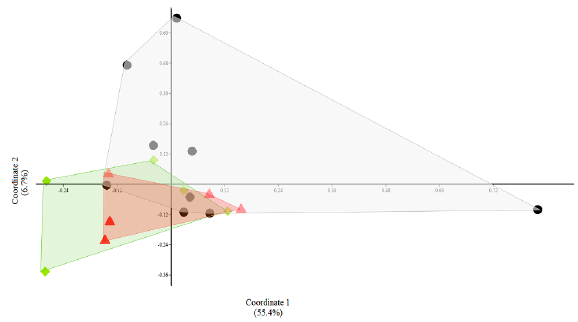
Figure 3 Principal coordinates analysis (PCoA) between three communities of gut bacterial morphospecies from howler monkeys from three habitats. The markers and polygons indicate the origin of the condition: circles: non-flooded area; triangles: flooded area; diamonds: captivity.
Firmicutes/Bacteroidetes Ratio
Firmicutes and Bacteroidetes Phyla are important components of the intestinal microbiota community (Durbán et al., 2011). However, most Firmicutes cannot grow under aerobic conditions. In our study, some Firmicutes were isolated exclusively from the howler monkeys captured in the non-flooded habitat and Bacteroidetes in the howlers of the flooded area. Nonetheless, though there are some biases in the Firmicutes/Bacteroidetes ratio, this reflects differences in the composition of the bacterial community between sites could be influenced by the food composition and availability of the monkeys (Amato et al., 2013, 2015). Firmicutes are prevalent in fermenter mammals, humans, and nonhuman primates (Frey et al., 2006). However, Bacteroidetes are present to a lesser extent in human vegetarians and gorillas (Hayachi et al., 2002; Frey et al., 2006). The ratio of these two Phyla is an indicator of how the bacterial community changes according to food composition and availability (Mariat et al., 2009). For the surveyed howler monkeys, the Firmicutes/ Bacteroidetes ratio was higher in captive animals, possibly reflecting that the diet is low in fiber and high in protein and fat (Gómez et al., 2016), which increases the production of metabolites derived from amino acids associated with inflammation of the colon (Mariat et al., 2009; Gómez et al., 2016). These intestinal inflammatory processes are the most common non-infectious diseases in captive howler monkeys (Pastor-Nieto, 2015). On the contrary, in wild howler monkeys, a decrease in Firmicutes/Bacteroidetes ratio is observed. Firmicutes decrement is observed when weight loss occurs in the host or diet is high in fiber and polyphenolic compounds (Gómez et al., 2016). In the flooded area, the ratio is higher likely due to the consumption of plants with high protein concentration (e. g. Pithecellobium sp., Enterolobium sp., Maclura sp., Guazuma sp., Tabebuia sp.) (Aristizabal, 2013; Aristizabal et al., 2017). However, in the wild, the howler monkeys feed on high concentrations of fiber and mostly on average concentrations of condensed tannins (Aristizabal, 2013) which could promote an increase of Bacteroidetes (Mariat et al., 2009; Gómez et al., 2016). This is observed in the non-flooded area in which the presence and consumption of Haematoxilum campechianum, a plant species with a high concentration of tannins that Alouatta consumes up to 25.7 % of the diet in the dry season (Pozo-Montuy and Serio-Silva, 2006). The consumption of tannins affects the composition of the microbiota (dysbiosis) due to the creation of a protein-tannin complex (Dawson et al., 1999), and this may be reflected in the weight loss of individuals (Mariat et al., 2009).
Captive Howler monkeys
The captivity conditions are considered an artificial environment, where a balanced diet is provided to animals to meet their nutritional requirements (Pastor-Nieto, 2015). Nevertheless, diets supplied to howler monkeys in captivity are not rich in natural fiber and mineral components compared to food consumed in the wild. The monkeys of the Chapultepec Zoo were fed with low fiber and sugar and a gluten-rich diet consisting of Leaf Eater Biscuit (Mazuri Primate Leaf Eater Diet, PMI Nutrition International), commercial cereals (wheat, oats, soy, barley) (Nestle Company), yogurt, fresh leafy greens, and some fruits so that this captive environment also produces changes in the composition of the intestinal microbiota (Nakamura et al., 2011).
Agreeing with our hypothesis, results reflect a loss of intestinal microbial diversity in the captive black howler monkeys, meeting up less than half of bacterial morphospecies than those found in wild howler monkeys. In captivity, the bacterial community was dominated by Streptococcus vestibularis and E. coli. The first one is an inhabitant of the human oral mucosa, and mucosa of the mammary glands of cattle (Pombert et al., 2009). On the other hand, E. coli inhabits the gastrointestinal tract of humans and animals; for this reason, many bacterial species can be shared between different animal species, which suggests that the spatial overlap between vector species affects the dynamics of the intestinal microbiome and bacterial transmission (Rwego et al., 2008).
Some bacterial morphospecies isolated from rectal swabs is commensal and natural inhabitants of the oral cavity of many mammals including humans and nonhuman primates. These bacteria may come from contamination of the fecal sample of the captive monkeys, contamination of the food, or poor hygiene of keepers of the captive animals. From the phylum Firmicutes, we found Lactococcus sp., which has a probiotic function in the intestinal microbiota of many species, and it is a facultative anaerobe related naturally to the animal gastrointestinal tract (Bolotin et al., 2001). The presence of these species of bacteria in captive howlers could be associated with the inclusion of fermented dairy products in their diets, such as yogurt, which are offered as a preventive or treatment of gastrointestinal imbalances, gastric dilatation, and wasting syndrome (Pastor-Nieto, 2015). These diseases are common in the genus Alouatta when offered diets that are rich in sugars, immunogenic cereal proteins such as gluten, poor in natural fiber sources, and excessive amounts of fruit (Pastor-Nieto, 2015).
Wild howler monkeys from non-flooded area
The highest microbial diversity was found in the howler monkeys from non-flooded areas, probably due to the vegetal composition and structure. In this area, the dominant plant is Guazuma ulmifolia, from which howler monkeys eat leaves and fruits up to 6.4 % of the time, being the fourth plant species most consumed by howlers in the area (Aristizabal, 2013). Of other plant species reported in the same area, flowers, and fruits of Sabal mexicana are consumed 1.1 % of the time when they are available, like the leaves of Enterolobium cyclocarpum (Aristizabal, 2013; Aristizabal et al., 2017). These plants possibly were not sufficient to cover the food and energy requirements of howler monkeys; it has been reported that howlers eat other plants from the arboreal and non-arboreal layers as supplementary feeding. The consumption of plants from the non-arboreal layer may influence the intestinal microbiota of howler monkeys, as these plants are easily digested due to lower contents of fiber (Aristizabal, 2013).
Bacterial dominance in the intestinal microbiota of howler monkeys includes three morphospecies mainly Myrioides sp./Chryseobacterium indologenes, Micrococcus sp., and Escherichia coli. The presence of facultative bacteria from soil, water, and plants in samples of howler monkeys could suggest that these primates are using the non-arboreal layer, whether for food, access to water sources or to move between areas promoting spatial overlap with other animal species and bacterial transmission (Goldberg et al., 2007; Rwego et al., 2008).
In our study, Firmicutes, such as Lactobacillus spp. were found exclusively in howler monkeys captured in the non-flooded area, suggesting that the plants available for monkeys (dominance Pithecellobiun, and elements of Sabal mexicana, Haematoxilum campechianum, Lonchocarpus guatemalensis, and E. cyclocarpum) are promoting growth and maintenance of probiotic bacteria due to fiber fermenting activity for lactic acid production (Frey et al., 2006).
Other Firmicutes were also exclusive in animals from the non-flooded area, namely Streptococcus inae and Salmonella cholerasuis. Streptococcus inae is an emerging pathogen associated with the intake of fresh fish (Baiano and Barnes, 2009). Probably this bacteria has been transmitted to Alouatta via contact when they reach the ground contaminated by carcasses of armored catfish species (Pterygoplichthys or Hypostomus spp.: Loricariidae). These armored catfish are invasive species that when the Usumacinta River is low-level, the fish are trapped in small ponds. When the ponds are dried, the fish die, leaving the carcasses in putrefaction. The fact that Balancán zone is backyard livestock may explain the presence Salmonella spp. This zoonotic bacteria of global distribution is transmitted by contaminated food or water, and some serotypes have been isolated from the gastrointestinal tract of small livestock and cattle. Nonhuman primates and other wild species could also be reservoirs playing an important role in its transmission and disease persistence (Oludairo et al., 2013).
Wild howler monkeys from flooded area
The vegetation of the flooded area is dominated by Sabal mexicana, but there also are other plant species with high protein content (e. g. Pithecellobium lanceolatum and Enterolobium cyclocarpum). Besides, the black howler monkeys also consumed high quantities of Maclura tinctoria, Guazuma ulmifolia, Tabebuia rosea (Aristizabal, 2013; Aristizabal et al., 2017).
Many bacteria identified in the microbiota of skin and mucous membranes (e.g., Streptococcus spp., Staphylococcus spp., and Bacillus spp.) are represented as singletons when considering the fecal bacterial diversity of monkeys from the flooded area. Although disinfection procedures were applied before the collection of the samples, these bacteria could contaminate the rectal swabs by contact with the anus or perineum skin. When howler monkeys go down to a ground contaminated by rodent and pork feces, they could also become contaminated. Likewise, howlers can become contaminated in forest fragments surrounded by fields of sorghum (Sorghum spp.), which remains contaminated when the flood has passed. This same contamination process could be responsible for the presence of Bacillus, Salmonella ssp., Serratia sp., and Tatumella sp., including the contact with contaminated soil and water by the human and domestic animal presence (Grimont and Grimont, 2006; Oludairo et al., 2013).
Some bacterial morphospecies were found in howler monkeys from at least two different habitats, including Staphylococcus cohnii, which is a commensal bacterium of skin and mucous membranes with a high potential to adhere to epithelial cells (Álvarez-Posadilla et al., 2006). This finding could be due to bacterial contamination during sample handling. However, other bacterial morphospecies could reflect some behaviors of howler monkeys, such as Photorhabdus (Xenorhabdus) luminescens, which is a pathogenic bacterium from insects and a symbiont of soil nematodes (Peel et al., 1999). The monkeys eat plants on non-arboreal strata (Aristizabal, 2013) and they also accidentally ingest soil nematodes, or they can be accidentally eating insects infected by P. luminescens.
Finally, Vibrio species found (V. cholerae and V. mimicus) have medical importance because they can be contaminants of water and food, respectively (Igbinosa and Okoh, 2008). Humans are the only host for V. cholerae and reservoirs are not known in nature (Igbinosa and Okoh, 2008). Many species of Vibrio are considered emerging, and the overlap of habitats can increase the transmission and interchange of these microorganisms, some of which can develop as opportunistic pathogens especially when interspecific interactions by hosts are frequent (Goldberg et al., 2007; Rwego et al., 2008).
Bacterial communities as entities are energetically efficient and metabolically flexible to environmental changes (Apajalahti, 2005). The intestinal microbiota not only has a role in the digestion of dietary components but also influences the metabolism and feeding behavior of the host (Alcock et al., 2014). Although the intestinal microbiota is stable (Mariat et al., 2009), it is also easily influenced, either by-products pro and prebiotics as well as changes in diet (Alcock et al., 2014). The intestinal microbiota may be susceptible to microbial invasion of opportunistic bacteria when changes or disturbances happen. These invasive microorganisms can dominate and produce dysbiosis due to loss of diversity and, consequently consequences to the health, as we found in captive howler monkeys. It has been reported that this loss of bacterial diversity could be reduced by sixfold to the high richness observed in wild monkeys, as reported by Amato et al. (2013). A microbial community with high species richness is less susceptible to invasions, and its resilience is higher, especially when disturbances are small and short-term (Lozupone et al., 2012), as we detected in howler monkeys from non-flooded habitats. Changes in the availability and distribution of food resources are some factors influencing the host's composition of the host's intestinal bacterial community (Hayashi et al., 2002; Yildirim et al., 2010; Lozupone et al., 2012; Amato et al., 2015). In addition, probiotic bacteria intake and some plant polyphenolic compounds favor the development and composition of intestinal microbiota (Sanz et al., 2004).
The studies in gut microbial diversity not only should evaluate the richness of microbial species but each host individual must be considered a habitat where a specific bacterial community develops and is influenced by many evolutionary and ecological factors (Ley et al., 2008; Mariat et al., 2009; Lozupone et al., 2012; Alcock et al., 2014). Although we did not find any differences between bacterial communities of the animals from the three habitats by ANOSIM and PCoA, the Shannon index-test showed similarities among monkeys from flooded habitats and captive howler monkeys, suggesting that bacterial diversity loss is due to the poor food quality and diversity loss, which supports our hypothesis.
Depending on the host species to be evaluated, there are difficulties and advantages in the study of intestinal bacterial communities. The sampling method, storage, and processing of samples sometimes have some limitations; however, rectal swabs have been a convenient method for a simpler collection and storage of samples without perturbation of the microbiota (Budding et al., 2014). However, some bacterial morphospecies found in this study may have been obtained by contamination (Durbán et al., 2011). The existence of some bacteria could be due to the type of feeding of Alouatta since it has been observed that non-human primates have complex microbial communities when their feeding is based on leaves. The leaf-eating primates can present structuring intermediate communities including bacterial types from omnivorous and herbivorous species (Ley et al., 2008), which increases the possibility of finding high species diversity and differences between host individuals and small-scale landscapes.
CONCLUSION
Our findings show that changes in the plant composition and the structure ofthe highly fragmented landscape in small scales alter the diversity of the rectal microbiota of A. pigra inhabiting two areas neighboring for loss of the availability and diversity of food. According to our hypotheses, the structure of the intestinal bacterial community can be an indicator of howler monkeys' health, habitat quality, and recognition of local adaptations of microbiota and their hosts. Likewise, captivity promotes the loss of diversity of the intestinal microbiota of howler monkeys. In addition, we suggest that the intestinal microbial diversity of animals in captivity should be monitored to promote health and animal welfare and, use specific probiotics if necessary.