INTRODUCTION
The agronomic performance of crops depends on edaphoclimatic factors, which are balanced under optimal conditions. Crops are subject to various types of biotic stresses (pests and diseases) and abiotic stress, isolated or combined. But in recent years, high contents of salts in water and in soil have become a significant problem in agriculture, especially in arid and semi-arid regions (Syvertsena and Garcia-Sanchez, 2014).
In arid and semi-arid regions, low rainfall and irregularity in rainfall mean that supplementary irrigation is almost mandatory, which, along with the need to expand cultivated areas, make low-quality water an alternative for irrigation (Lima et al., 2014).
Plants behave differently when saline water is used, and sensitivity to salinity depends on various factors, such as variety and plant exposure time (Pedrotti et al., 2015). When subjected to salt stress, plants experience change at the cellular level, including destruction of root plasma membrane and ionic stress on stem cells (Reis et al., 2016); therefore, a major symptom is stomatal closure, with a consequent lower photosynthetic activity, causing decreases in growth and productivity (Fraire-Velázquez and Balderas Hernández, 2013; Reis et al., 2016).
In recent years, one of the strategies to reduce the harmful effects of salt stress is using fertilization with silicon (Sahebi et al., 2016). Studies indicate that silicon can increase plant tolerance to salt stress since absorption of K and Ca ions contributes to the maintenance of K and Na contents in plants; however, mechanisms that contribute to increased tolerance to salt stress have not been discovered (Dias and Blanco, 2010; Shi et al., 2013; Castellano et al, 2016)
There are a few studies on some of the effects of Si in plants. These studies have shown that this element could mitigate the deleterious effects of salts in plants, but, for beets, there is little research that elucidates attenuator effect on salt stress. Thus, this study aimed to evaluate the effect of saline water and silicon applications on growth, chloroplastid pigments, fluorescence chlorophyll a and beet production.
MATERIAL AND METHODS
This experiment was conducted from January to March, 2018 in a greenhouse located in the Fruit sector belonging to the Federal University of Paraíba in Areia-PB, Brazil, located at 6°51'47" and 7°02'04" South latitude and West longitude, 35°34'13" and 35°48'28" Greenwich meridian.
The experiment design used randomized blocks and a 5 X 5 factorial arrangement, combined according to the Central Composite of Box experiment matrix (Mateus et al., 2011) for the electrical conductivity of the irrigation water (ECw) and silicon doses (Si), with minimum values (-a) and maximum values (a) of 0.5 to 6.0 dS m-1 and 0.00 to 18.16 mL L-1, totaling nine treatments, with four replicates and three plants per plot (Tab. 1).
Beet cv. Wonder seedlings were grown in trays and planted in pots with a 22 cm top diameter, 16 cm bottom diameter, 18 cm height, 8 dm3 volumetric capacity, and 1 cm circular holes in the bottom to allow better root aeration and percolation of excess water.
The containers were filled with horizon A soil, collected at a depth of 0-20 cm, classified as Planossolo Háplico Eutrófico êndico/Alfisol (Embrapa 2014). The chemical and physical characteristics (Tab. 2) were analyzed according to the methodology of Embrapa (2014). The soil was air dried, homogenized, and placed in containers accommodated previously with screen (tulle fabric) and 200 g of crushed rock, while preventing the soil from coming out of the holes in the bottom.
Table 2 Chemical and physical characteristics of the soil.
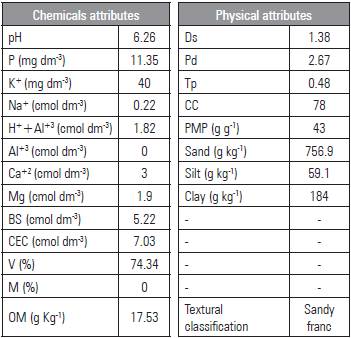
Base sum (BS) = (Na++K++Ca2++Mg2+); CEC = cation exchange capacity; EC = BS + (H++Al3+); V = (100 x BS/CEC); OM = organic matter. Ds = density of the soil; Pd = particle density; Tp = total porosity; (1- (Ds/Dp) * 100) Ucc = volumetric humidity level of field capacity - 0.033 MPa; Upmp = humidity level of the permanent wilting point - 1.5 MPa.
The plants were irrigated daily, bringing the soil moisture to about 80% of field capacity (CC). The different ECw were obtained by with the salts NaCl, CaCl2 2H2O and MgCl2 6H2O, at a ratio of 7:2:1 according to the characteristics (Tab. 3). Irrigation with water with different salinities was initiated 10 d after emergence. On the first day after emergence (DAE), the blade was calculated with the equation proposed by Mantovani et al. (2009); the total required irrigation (TRI) was calculated with the equation by Bernardo et al. (2008), considering 100% of irrigation application efficiency.
Table 3 Chemical characteristics of the water.
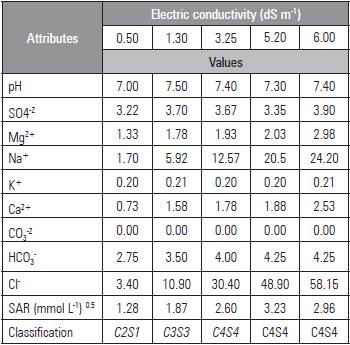
EC= electrical conductivity at 25 °C; SAR = sodium adsorption ratio [Na+/(Ca2++Mg2+/2)1/2]; CO3 2- = Absent. Water classification according to Richards (1954).
The silicon was applied in the liquid potassium silicate form (K2SiO3) with 12% Si and 15% K2O. The Si doses were applied via the soil. However, there was compensation of K2O via soil, the application used in the beet aiming to provide the same amount of potassium to all plants. The application was done weekly, totaling 7 applications during the development and production of beets. The doses (Si) were diluted in 1.2 L of distilled water, and 50 mL of this solution were applied to each plant.
During the experiment, weather data (Fig. 1) were recorded daily with a digital thermo hygrometer, HT-600 Instruthermr®, installed in the experimental area, at the height of the plants. The average air temperature was near the ideal range (25°C) during the crop cycle, according to Filgueiras (2008).
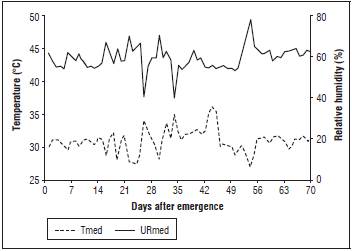
Figure 1 Graphical representation of relative humidity and temperature in the experiment. Mean Air temperature (Tmed) and mean relative humidity (URmed).
The fertilizer at sowing and covering was done with 40, 180 and 90 kg ha-1 of NPK, with urea, superphosphate and potassium chloride, according to the chemical analysis of the soil and fertilizer recommendations for State of Pernambuco, Brazil (IPA, 2008). During the conduction of the experiment phytosanitary controls of pests and weeds were made.
The growth of the beet plants was evaluated by measuring plant height (measured with a ruler graduated in cm), leaf area ((by measuring the length (C) and width (L), and by applying in the AF formula = C * L * f, with AF = leaf area in m2; C = length of the leaf in m; L = leaf width in m; and f = correction factor for beets (0.692), according to Simões et al. (2016)), number of leaves, and leaf length and width (using digital caliper) at 60 d after emergence.
At the end of the experiment, measurements were taken: longitudinal and transversal diameter of the bulb (using digital caliper); bulb fresh mass, leaf fresh mass, leaf dry mass, root dry mass, fresh mass bulb (through weighing on an analytical balance), leaf chlorophyll indices a, b, total and a/b ratio with a Clorofilog® chlorophyll meter (Falker). The readings were taken with intermediate leaves of the four central plants in the experiment area, performing four readings per plant.
The initial fluorescence (F0), maximal fluorescence (Fm), variable fluorescence (Fv) and quantum efficiency of photosystem II (Fv/Fm) were also evaluated using a portable fluorometer (PEA - Plant Efficiency Analyzer, Hansatech).
The data were subjected to analysis of variance, and, when significant, the data were subjected to polynomial regression analysis (P≤0.05). For the data that were not significant, standard deviations of the mean were carried out. The SAS University (Cody, 2015) software was used for these analyses.
RESULTS AND DISCUSSION
Plant height was adjusted to a quadratic model as a function of salinity in the irrigation water. There was a reduction in plant height with the increasing salt concentrations, reaching the point of maximum efficiency when the plants were irrigated with 0.40 dS m-1, corresponding to a height of 27.50 cm; the plant height was reduced when irrigation was done with high salinity water (Fig. 2A).
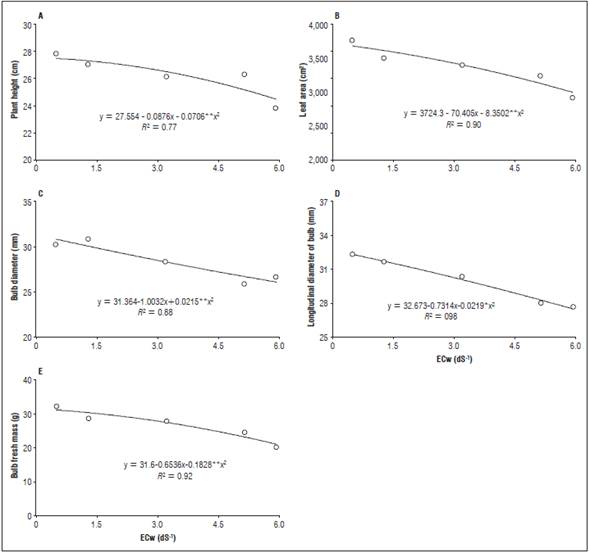
Figure 2 Plant height (A), leaf area (B), bulb diameter (C), bulb longitudinal diameter (D) and fresh bulb mass (E) of beet plants irrigated with water with different electrical conductivities.
A reduction in plant height was also observed by Silva et al. (2015), who found that the greatest height of beet cv. Itaapuã plants was 43.6 cm with 6 dS m-1 irrigation water, with a reduction with increasing salinity. Santos et al. (2016) observed that the maximum height was obtained with 3.11 dS m-1, 12.8 cm, confirming the results obtained in this study, i.e. the increased salinity in the irrigation water significantly reduced the height of the plants.
The same behavior was observed for the leaf area, which decreased as salinity increased. The largest leaf area was 3720.75 cm2, in the plants irrigated with 0.5 dS m-1 water (Fig. 2B). This reduction may have been caused by morphological and physiological changes, one of the first symptoms being a reduction in leaf area, which is a way to increase tolerance to salinity.
Santos et al. (2016) observed that the maximum leaf area was obtained with a salinity of 2.85 dS m-1, 21 4 cm2. This result is lower than that obtained in the present study. In studies on radish crops, Oliveira et al. (2012) found that the greatest leaf area value was observed in plants irrigated with lower salinity water (2 dS m-1), yielding 497.20 cm2, while the highest ECw (10 dS m-1) resulted in a smaller leaf area, 220 cm2, a reduction of 55.75%, corroborating the data obtained in this study.
The bulb diameter and the bulb longitudinal diameter decreased when the beet plants were irrigated with saline water, decreases with increasing salt. Larger diameters were obtained with plants irrigated with water with a lower salinity, yielding 31.26 mm with 0.10 dS m-1 irrigation water (Fig. 2C) and 32.56 mm with 0.15 dS m-1 water (Fig. 2D).
The bulb fresh mass also decreased with the increased salts in the irrigation water; the largest bulb fresh mass was 31.49 g when the beet plants were irrigated with 0.14 dS m-1 (Fig. 2E).
The results obtained in this present study agree with those of Silva et al. (2015) who studied the management of fertigation and soil salinity in terms of growth in a beet culture, observing that increasing the salinity of the water reduced the beet plant bulb diameter.
The salinity in the irrigation water did not significantly affect the analyzed variables (Tab. 4). However, the highest values were observed in the beet plants irrigated with low salinity water (0.50 dS m-1), and, although no significant effect was found, the lowest values were observed with higher salinity irrigation water (6.00 dS m-1), proving that the salt stress dramatically reduced the growth and development of the plants, as was observed by Silva et al. (2015) and Santos et al. (2016) in beets, and by Shi et al. (2013), Lima et al. (2014) and Reis et al. (2016) in rice, roses and castor, respectively.
Table 4 Mean ± standard deviation of the variables leaf width (Wl), leaf length (Ll), leaf area (La), number of leaves (Nl), chlorophyll content A (Cl a), chlorophyll B (Cl b), total chlorophyll (Total Cl), chlorophyll a/b ratio (Cl a/b), leaf fresh mass (Lmf), leaf dry mass (Ldm), root dry mass (Rdm), cross bulb diameter (Cbd), initial fluorescence (F0), maximal fluorescence (Fm), variable fluorescence (Fv) and quantum efficiency of photosystem II (Fv/Fm) of beet plants as a function of irrigation with saline water (ECw).
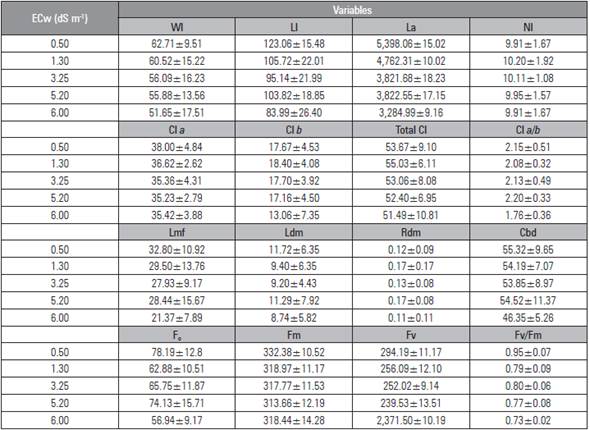
By irrigating the beet plants with 6.00 dS m-1 water, smaller values were obtained for leaf fresh mass, leaf dry mass and root dry mass, which may have been caused by a decrease in osmotic potential, the soil solution, and the excessive accumulation of salts, inhibiting the absorption of water from the beet plants and, as a result, requiring greater energy for the absorption of water and nutrients and reducing growth and biomass accumulation (Sá et al., 2015; Bertazzini et al., 2018).
The smallest values for the chlorophyll contents a, b and total chlorophyll a and fluorescence photochemical efficiency of photosystem II when the plants were irrigated with water with higher salinity (6.00 dS m-1) were due to the reduction in the activity of photosynthetic enzymes, limiting the electron transport in the chloroplasts. The accumulation of Na+ and Cl-1 in chloroplasts changes the photosynthetic activity of plants, which reduces the photochemical efficiency of photosystem II (Huang et al., 2012).
The same behavior was observed by Silva et al. (2013), who found a decrease in the growth and physiological parameters of beet cv. Early Wonder with increased salinity. Silva et al. (2015) also found that the salinity in irrigation water reduced the growth of beet plants.
It was observed that the leaf area decreased with the increase of the silicon doses, up to 9.08 mL L-1, with a subsequent increase up to 18.16 mL L-1, reaching a maximum leaf area of 3591.15 cm2 with 0.26 mL L-1 (Fig. 3A). The longitudinal diameter of the bulbs presented the same behavior, with the greatest longitudinal diameter, 32.45 mm, observed in the plants treated with 0.24 mL L-1 of silicon (Fig. 3B).
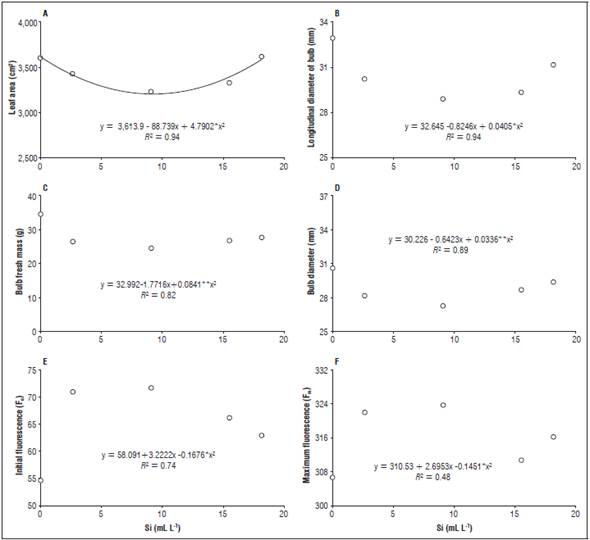
Figure 3 Longitudinal diameter of the bulbs (B), bulb fresh mass (C), bulb diameter (D), initial fluorescence (E) and maximum fluorescence (F) in beet plants for the different doses of leaf silicon.
The fresh mass of the bulb and the bulb diameter decreased with increased doses of silicon, up to 9.08 mL L-1; however, higher doses of Si increased these variables. The highest values for bulb fresh mass (Fig. 3C) and bulb diameter (Fig. 3D) were obtained when the plants received 0.23 mL L-1 and 0.26 ml L-1, yielding 32.60 g and 30.06 mm.
The initial fluorescence and maximum fluorescence increased with doses up to 9.08 mL L-1, with reductions over this dose. The highest values were observed when applying 9.08 mL L-1 with values of 71.63 (Fig. 3E) and 323.75 (Fig. 3F), respectively.
The silicon doses did not influence the analyzed variables (Tab. 5). However, the dose of 9.08 mL L-1 promoted higher contents of chlorophyll a, b, and total, and a higher dose (18.16 mL L-1) provided higher chlorophyll fluorescence (Fv) and quantum efficiency of photosystem II (Fv/Fm), indicating that Si may mitigate the effect of salt stress because of the increase of photosynthetic pigments (Rezende et al., 2018).
Table 5 Mean ± standard deviation of the variables plant height (Ph), leaf width (Wl), leaf length (Ll), number of leaves (Nl), chlorophyll content a (Cl a), chlorophyll b (Cl b), total chlorophyll (Total Cl), chlorophyll a/b ratio (Cl a/b), fluorescence (Fv), quantum efficiency photosystem (Fv/Fm), leaf fresh mass (Lfm), leaf dry mass (Ldm), root dry mass (Rdm) and transverse bulb diameter (Tdm) of beet plants under silicon applications via the soil.
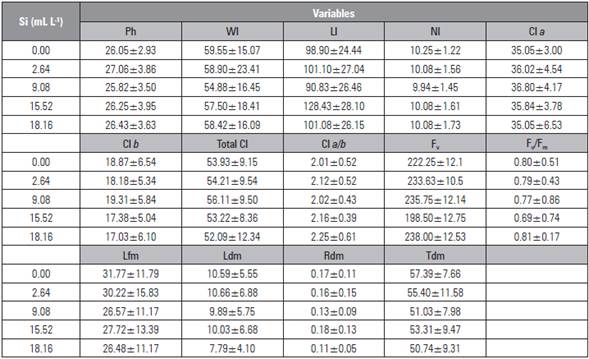
Although there was not a significant effect on the analyzed variables, greater values of chlorophyll a, b and total, fluorescence (Fv) and quantum efficiency of photosystem II (Fv/Fm) confirmed that Si has a significant influence on the photosynthesis and biochemistry of plants. Several studies have demonstrated the positive effect of Si, as reported by Bae et al. (2012), Tahir et al. (2012) and Yin et al. (2013), who found that an increased availability of Si increases growth, photosynthetic and biochemical aspects of plants.
As noted in this study, several studies have shown that Si promotes growth and photosynthetic activity (Bae et al, 2012; Tahir et al, 2012; Yin et al, 2013); leaves become more erect, allowing a greater absorption of CO2 and higher photosynthetic efficiency and chlorophyll contents. Si accumulation on the leaf surface may have promoted a physical barrier in the leaves of the beet plants, playing an important role in osmotic adjustment (Heckman, 2013; Cantuário et al., 2014).
CONCLUSION
The increase of electrical conductivity in the irrigation water reduced the growth and production of the beets, but the chlorophyll contents, biomass production and fluorescence were not influenced by irrigation with saline water.
The application of silicon in the soil promoted increases in growth and chlorophyll fluorescence a; however, it did not reduce the harmful effect of salt stress.
Electrical conductivity in the irrigation water above 0.50 dS m-1 adversely affected the beet cultivation, and the silicon dose of 9.08 mL L-1 is recommended for applications.