INTRODUCTION
Stevia rebaudiana (Bertoni) Bertoni is a small perennial growing up to 65-80 cm tall, with sessile, oppositely arranged leaves. Only one stevia species contains several sweetening compounds, with rebaudioside A being the sweetest, and it can be up to 300 times sweeter than common sugar (Basharat et al., 2021). The number of people suffering from cardiovascular diseases, diabetes, hypercholesterolemia, and obesity has increased globally. Consequently, the demand for non-caloric sweeteners has increased while sugar consumption has decreased (Putnik et al., 2020).
At first glance, S. rebaudiana can be successfully grown under different conditions regarding climate and soils, because it is adapted to poor soils, with low nutrient requirements (Ramesh et al., 2006; Hussin et al., 2017). However, irrigation should be carefully used to avoid an increase in soil salinization. It has been estimated that soil and water salinity is pervasive throughout the globe, reaching 20% of the total irrigated land area in the world (Huang et al., 2019). Although the biochemical, physiological, and agronomical aspects of S. rebaudiana have been extensively investigated under optimal environmental conditions, its response to environmental constraints such as salinity and/or drought has been unclearly reported. Some scholars (Ramesh et al., 2006; Zeng et al., 2013; Reis et al., 2015; Cantabella et al., 2017; Hussin et al., 2017; Debnath et al., 2019; Shahverdi et al., 2019; Jarma-Orozco et al., 2020) have described the pattern S. rebaudiana plants in face of water and salt stress. These authors reported that S. rebaudiana plants under salt stress show events that influence the photosynthetic process due to stomatal closure as this limits CO2 fixation. S. rebaudiana seemed to be tolerant to the imposed NaCl stress, given that no negative effects of salinity on plant growth were detected (Ramesh et al., 2006; Cantabella et al., 2017; Hussin et al., 2017). However, other studies (Zeng et al., 2013; Reis et al., 2015) have described that the total dry weight decreased by 40% at 0.12 M NaCl but remained the same at 0.06 and 0.09 M NaCl; indicating that hypohaline soil can be utilized in the cultivation of S. rebaudiana and may be profitable for optimizing the steviol glycoside composition. However, Cantabella et al. (2017) describes that NaCl induces reactive oxygen species (ROS) accumulation in S. rebaudiana leaves. Anyway the salt-sensitivity or tolerance seems to be a cultivar-dependent as previously reported by Debnath et al. (2019). Thus, irrigation with saline water can be used to grow S. rebaudiana plants for steviol glycoside production (Cantabella et al., 2017). If the main goal of cultivation of S. rebaudiana still is stevioside production, Shahverdi et al. (2019) describes that S. rebaudiana should be irrigated with non-saline water, because 0.15 M NaCl promoted the reduction of plant height (42%), leaf dry weight (46%), chlorophyll a (40%), chlorophyll b (47%), total sugars (48%), with an accompanying increase of the MDA content (7.3-fold). These authors described that the highest percentage of stevioside and rebaudioside A were detected at lowest NaCl concentration (e.g., 30 mM), denoting that S. rebaudiana should be compared to a salt-sensitive plant.
Understanding how S. rebaudiana plants can face salt stress under greenhouse conditions can be fundamental in improving the management and production of this agronomically and economically important plant, especially since the climate-change scenarios foresee an increase in aridity in many areas where this species is cultivated (Cantabella et al., 2017; Hussin et al., 2017; Jarma-Orozco et al., 2020). Moreover, there is less information concerning the effects of salt stress on photosynthesis and chlorophyll fluorescence in S. rebaudiana or their correlations with electron transport sink (Hussin et al., 2017; Acosta-Motos et al., 2019). The main goal of this study was to analyze the gas exchange, electron transport rate, photosystem (PS)II efficiency during the exposure to sunlight (ΦPSII), electron transport rate (ETR) and growth parameters in two morphological distinct genotypes, subjected to high salt concentration in the irrigation water. This way, the effect of salt stress on S. rebaudiana and the threshold salinity level of the species will be revealed to cope with salinity in Caribbean salted-soils.
MATERIALS AND METHODS
Plant material and environmental conditions
The study was carried out in the same conditions described in Hernández-Fernandéz et al. (2021) containing two Stevia rebaudiana genotypes obtained by natural pollination in a controlled greenhouse. Cuttings of genotype 04 (G04), and genotype 19 (G19) of S. rebaudiana were obtained (Fig. 1A). After 4-months growth in a shaded greenhouse in 3-L pots filled with a mix of sand, organic matter and coconut fiber (1:1:1 v:v; Fig. 1B-C) the plants were transfer to 10-L pots filled with Monteria soil (Colombia), plus river sand (3:1 v:v; Fig. 1D). The experimental set up was performed under completed randomized design, containing two genotypes (04 and 19), six salt concentrations (0, 0.28, 0.82, 1.65, 2.74, and 4.10 M) administered in the first day of salt stress. The salt concentrations ere designed to test the threshold salinity level in S. rebaudiana plants based on confusing previous results with this species. After salt administration, all plants were daily irrigated with 200 mL of tap water (without salt addition) to replace water lost by the atmosphere during the whole study period (60-d). All pots were designed avoid the escape of any irrigation water to escape through holes and, therefore, the leaching of previously salinized soil. All experiments were under the greenhouse as previously reported to this species on Caribbean conditions (Jarma-Orozco et al., 2020).
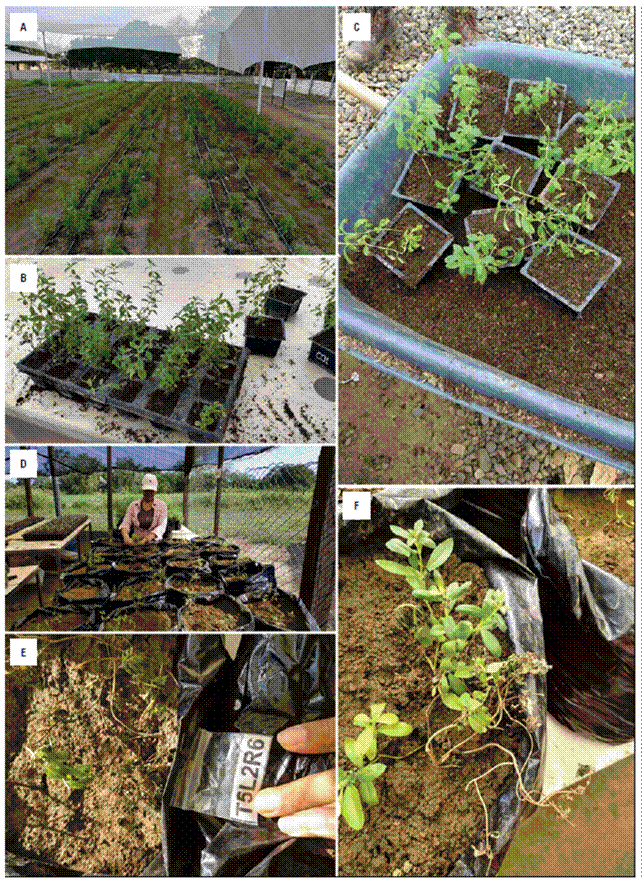
Figure 1. Timeline of Stevia rebaudiana plants from vegetative propagation to salt stress symptoms. A. Vegetative propagation in biospace nursery (Jarma-Orozco et al., 2020). B. Individually vegetative propagation plants. C. Growth plants in shaded greenhouse in 3-L pots. D. Growth plants in definitive 10-L pots. E. 30-days plants under 0.82 M NaCl, showing some burning of leaf edges or leaf dropping. F. 30-d plants under 4.1 M, showing leaf dropping, and brown necrosis.
Leaf gas exchange parameters and chlorophyll a fluorescence
Leaf gas exchange and chlorophyll a fluorescence were determined exactly as described in Dos Santos et al. (2019). All analysis were started on 6-month-old S. rebaudiana plants, under saturated leaf irradiance as previously tested by light curves versus net photosynthesis - P N (Jarma-Orozco et al., 2020).
Growth parameters
After 2-month-old salt (i.e., 6-months plants) the leaf dry weight (LDW), shoot dry weight (SDW), root dry weight (RDW) and total DW (leaves + shoot + root) were measured. The harvest index (HI) was calculated as (1)
Based on the data of root and shoot dry weights, root to shoot dry weight ratios (RSR) were calculated, after oven-drying at 60°C until constant weight. The value at which yield is reduced by 50% (C50) was calculated as described by González et al. (2015).
Principal component analysis
All measured physiological and morphological parameters were used to perform a principal component analysis (PCA) exactly described in Hernández-Fernandéz et al. (2021).
Statistical data analysis
A Shapiro-Wilk test (Shapiro and Wilk, 1965) and Brown-Forsythe test (Brown and Forsythe, 1974) were used to test for normality and variances. All data are presented as means plus standard errors (SE; n = 10). All results were analyzed with a two-way ANOVA. Means were compared using a Student test Newman-Keuls (SNK) with the statistical software package as well as the parametric correlations (Statistica v 8.0 StatSoft, Tulsa, OK).
RESULTS
Gas exchange and chlorophyll a fluorescence
After 30-d salt stress, gas exchange and chlorophyll fluorescence parameters were measured. However, the second evaluation at 60-d could not be done, because salinity dramatically reduced the leaf area, producing a few brown leaf tips, leaf dropping (Fig. 1E), and in the highest NaCl concentration leaf dropping, and brown necrosis, negatively affecting the analysis in this timeline (Fig. 1F).
The net photosynthesis (P N) was significantly reduced with increasing salt concentration, G04 being more affected by salinity than G19 (Fig. 2A). The P N of G04 dropped sharply from 9.89 μmol CO2 m-2 s-1 on non-saline plants to 4.92 μmol CO2 m-2 s-1 in the 0.82M NaCl; an abrupt drop of 50.3%. On the other hand, P N of G19, was slightly non-significant reduced from 9.32 μmol CO2 m-2 s-1 on non-saline plants to 7.07 μmol CO2 m-2 s-1 in the 0.82 M NaCl (reduction of 24%), but sharply and unexpectedly raised to 12.20 μmol CO2 m-2 s-1 in the 4.1 M, an increase of 72 and 31% when to 2.74 M and non-saline plants were used to compare basis.
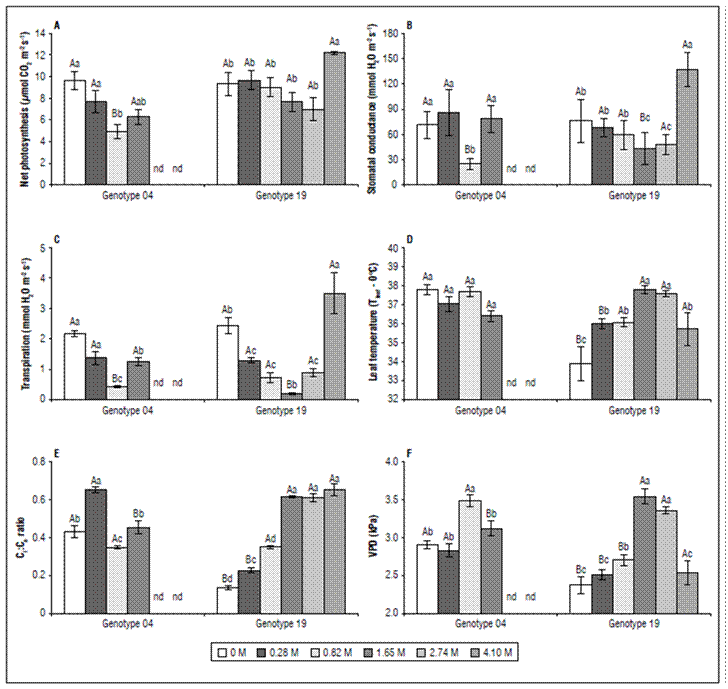
Figure 2. Gas exchange parameters measured in 30-d salt-stressed Stevia rebaudiana plants at G04 (left panels) and G19 (right panels). A. Net photosynthesis, B. Stomatal conductance, C. Leaf transpiration, D. Leaf temperature, E. internal-to-ambient CO 2 concentration (C i / C a ratio), and F. Vapor pressure deficit. All data are expressed as means ± SE, n = 10. Different lowercase letters denote significance within salt concentration for each the genotype, and different capital letters denote significance within genotype for the same salt concentration.
Except for 0.82 M NaCl, the stomatal conductance (g s) of G04 was not significantly affected by NaCl. At0.82 M NaCl the g s was dropped by 67% (a reduction of 1.6-fold). On the other hand, in the G19 the g s was slightly reduced (44%) from non-saline plants (76.1 mmol H2O m-2 s-1) to 2.74 M NaCl (45.5 mmol H2O m-2 s-1), and then sharply raised to 136.8 mmol H2O m-2 s-1 in the 4.1 M; an increase of 1.8-3.0-fold when compared to non-saline and 2.74 M NaCl plants (Fig. 2B).
The transpiration (T) pattern was like those shown in g s. The T of G04 under 0.82 M NaCl showed an abrupt fall of 2.4-fold, but with a strong recovery in the 1.65 M treatment. The T on 0.82 M NaCl, and 1.65 M NaCl were 19%, and 59% of those values measured on non-saline plants (Fig. 2C). In G19 the drop in T was higher than those shown in G04. Comparing non-saline and 1.65 M NaCl, we showed a fall of 92%; however, these values were slightly and strongly increased by of 4.5-17.4-fold when compared to those on 4.1 M NaCl (Fig. 2C).
The leaf temperature (Tleaf) was genotype-dependent because there was no significant modulation on G04, but an abrupt increase of 11.4% on G19 under 2.7 M NaCl, comparing to non-saline plants (Fig. 2D).
The internal to atmospheric CO2 ratio (Ci:Ca ratio), did not show any interesting modulation in G04, but a strong increase by about 1.4-, 2.2-, and 2.2-fold, in 0.82 M NaCl, 1.65 M NaCl, and 2.74 M NaCl, respectively. In 4.1 M NaCl plants, the Ci:Ca ratio calculated in G19 was 381% higher than that of non-saline plants (Fig. 1E). Similarly to Ci:Ca ratio, the vapor pressure deficit (VPD) did not show a biological interesting pattern, but an unexpected modulation increase of 48% on 1.65 M NaCl when compared to non-saline plants.
Taking all gas exchange data together, we argue that G04 does not seem to have its gas exchanges coupled with atmospheric variations to the same extend as G19. While in G04, there is a 20% increase in VPD with an increase in salinity between treatments 0.82 M (3.48 kPa) and non-saline (2.90 kPa), there was a significant drop (10.5%) between the treatment of 0.82 and 1.65 M. On the other hand, G19, seems to be more integrated with the atmosphere, since the DPV was increased by 1.5-fold between the 1.65 M and non-saline treatments, even though this DPV decreased at 2.7 M (4.6%) and 4.1 M (28.4%) treatments.
The electron transport rate (ETR) was similarly reduced in both salt-stressed genotypes (Fig. 3A). In G04 submitted to 0.82 M NaCl, the ETR was 80% smaller than in non-saline plants, even so 1.65 M NaCl seems to be recovering its ETR by 3-fold. ETR measured on G19 submitted to 1.65 M NaCl was strongly reduced by 86% when compared to non-saline plants, even so, 4.1 M NaCl unexpectedly increased its ETR by 2-18-fold when compared to non-saline and 1.65 M NaCl plants (Fig. 3A). Between all of the gas exchange and chlorophyll a fluorescence parameters, the ΦPSII was the most negative modulated. In G04, the reduction in ΦPSII was about 0.3-, 0.9-, and 1.2-fold in 0.28, 0.82, and 1.65 M NaCl, respectively. In G19, ΦPSII was also strongest negatively modulated by about 0.5-, 1.3-, 0.6-, 2.1-, and 1.7-fold in 0.28, 0.82, 1.65, 2.74, and 4.10 M NaCl, respectively. The relationship between ETR xP N was reduced by 2.8-, and 1.4-fold in 1.65 M NaCl, and 2.74 M NaCl, but showed an increase of 4-fold in 4.1 M NaCl, and this only in G19.
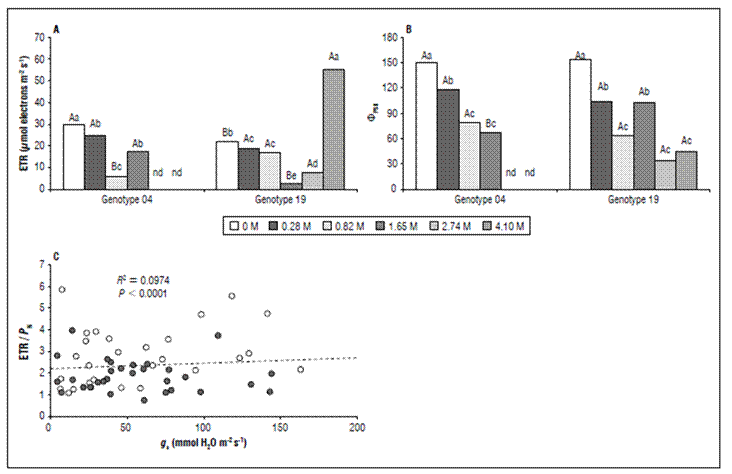
Figure 3. Electron transport rate (ETR; A), actual PSII efficiency during the exposure to sunlight (ΦPSII; B) measured for genotype 4 (left panels) and genotype 19 (right panels). Relationship between the ratio of electron transport rate (ETR) and net photosynthesis (P N) versus stomatal conductance (g s; C) measured in 60-d salt-stressed Stevia rebaudiana plants at genotype 4 (open symbols) and genotype 19 (filled symbols) subjected to six concentration of NaCl (0, 0.28, 0.82, 1.65, 2.74, and 4.1 M). The regression coefficient and P-value are shown. All data are expressed as means ± SE. n = 10. In A and B, different lowercase letters denote significance within salt concentration for each the genotype, and different capital letters denote significance within genotype for the same salt concentration.
Growth parameters
Variations between different salt treatments were evident two to three weeks after the start of salt treatments. From the concentration of 2.74 M NaCl it was possible to observe the symptoms of stress. At this salinity level, nearly completely expanded and juvenile leaves were characterized by leaf chlorophyll chlorosis, burning of leaf edges or leaf dropping (Fig. 1E), while the 4.1 M NaCl plants showed brown necrosis at the leaf margins starting with tip burning, which expanded towards the leaf center with ageing (Fig. 1F).
The overall aerial dry weight was differentially affected in response increasing NaCl concentrations, but in G04 the influence was more evident (Fig. 4). The salt stress decreased substantially (98.0, 97.7, and 93.8%) LDW, SDW, and total DW in 4.1 M and 93.8, 92.2, and 87.8% in the G19 (Fig. 4A, 4B, 4D). Whereas aerial DW was decrease by salt stress, the RDW were increased in about 43.3 and 38.2% in G04, and G19, respectively (Fig. 4C); leading consequently to increase in RSR. In 4.1 M-salt stressed plants of G04 and G19 there is an unexpectedincrease of 56-fold and 20-fold in RSR (1.90, and 0.83, in G04, and G19, respectively), when compared to non-stressed plants (0.03, and 0.04, in G04, and G19, respectively) (Fig. 4E). Regression analyzes of influence of salt stress on plant growth expressed as dry weight of different organs of S. rebaudiana showed that the value at which yield is reduced by 50 percent (C50) was 0.263 M, and 0.458 M, in G04, and G19, respectively (Infographic).
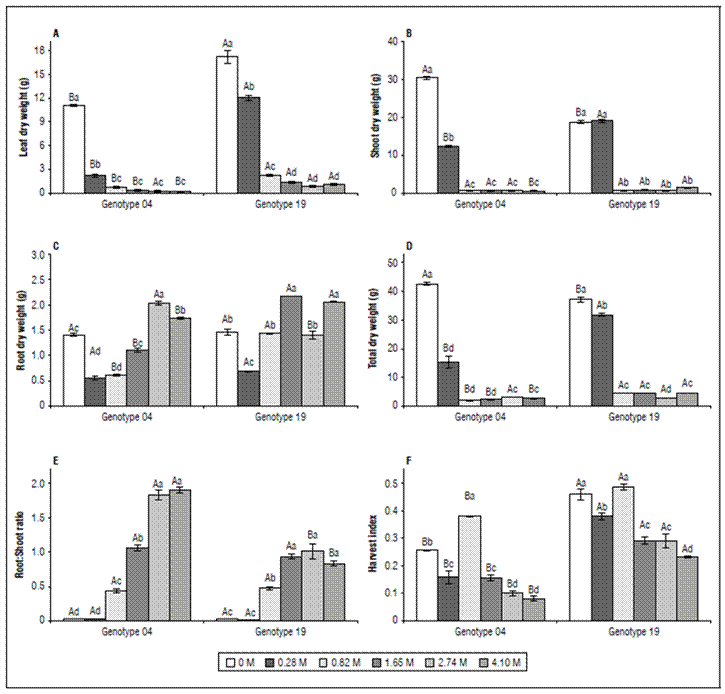
Figure 4. Plant biomass parameters measured in 60-d salt-stressed Stevia rebaudiana plants at genotype 4 (left panels) and genotype 19 (right panels). A. Leaf dry weight, B. Shoot dry weight, C. Root dry weight, D. Total dry weight, E. Root:shoot ratio, and F. Harvest index. All data are expressed as means ± SE, n = 10. Different lowercase letters denote significance within salt concentration for each genotype, and different capital letters denote significance within the genotype for the same salt concentration.
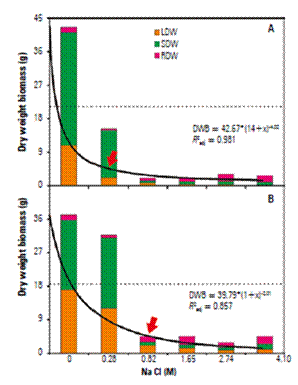
Infographic. Influenceof NaCl salinity on plant dry weight of different organs G04 (A) and G19 (B) genotypes of S. rebaudiana plants. Arrow indicates the salinity resistance threshold (i.e. inflection curve point), while the dotted line marks the C50 values. LDW: Leaf dry weight. SDW: shoot dry weight. RDW: root dry weight.
The harvest index (HI) was similarly influenced by salt stress in both genotypes. However, the drop shown in G04 was stronger (68%) than in G19 (49%). In both genotypes, an increase of 48% (in G04), and 5.6% (in G19) was seen at 0.82 M NaCl when compared to non-saline plants. The increase of HI at 0.82 M was significant in G04, but not in G19 (Fig. 4F).
PCA and dendrogram analyzes
The PCA analysis shows that each salinity level and genotype have a salt concentration-dependent pattern, nor a genotype-dependent pattern. Figure 5A clearly demonstrated that regardless of genotype the basal NaCl levels form a unique and homogeneous cluster. Similarly, the highest NaCl levels form another uniform cluster; even so the pattern shown in 4.1 M NaCl of the G19 form a third cluster. These groupings were confirmed by genetic distance at a line court of 38%. When we analyzed the contribution of each physiological and morphological parameters, and its direction and length in the loading plot (Fig. 5C, Tab. 1), we showed that the length in PC1, and PC2 ranged between -0.451 (PC2; ETR/P N) to 0.326 (PC1; P N). Since as, at first glance, P N seems to be more plastic than other parameters, the spatial distribution of all physiological and morphological parameters show us that this is very speculative, because other parameters modulated equally or more to P N. Thus, based on the direction and length contribution of each physiological and morphological parameter we can classify these parameters in accord of their descending length in three groups, namely group 1: P N, RSR, Tleaf, ΦPSII, g s, and WUEi; group 2: T, ETR, VPD, SDW, ETR/P N, and total DW; group 3: LDW, HI, Ci:Ca, and RDW.
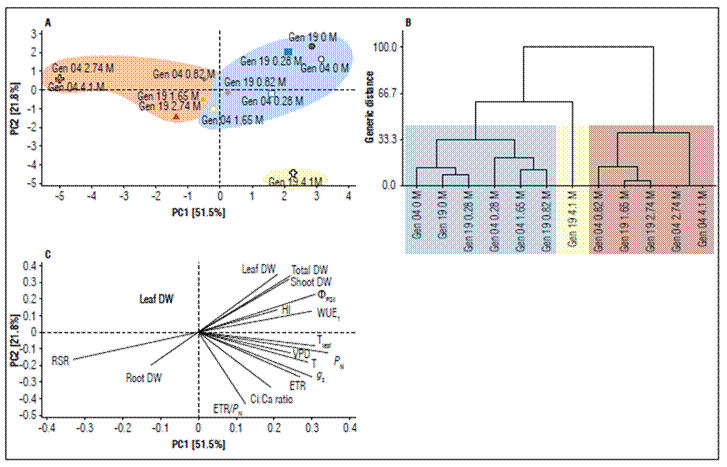
Figure 5. A. Principal component analysis to Stevia rebaudiana at G04 (circles) and G19 (squares) showing the spatial distribution of each physiological and morphological features. All plants were submitted to 0 M (orange), 0.28 M (cyan), 0.82 M (green), 1.65 M (brown), 2.74 M (red), and 4.1 M (black) of NaCl. B. Dendrogram indicating the phylogenetic grouping of treatment, regardless of the genotype. The formation of clusters was done at a line court of 38%. C. Loading plot graph. The direction and length of the lines are directly proportional to variables’ importance in separating groups. PC1, principal component 1; PC2, principal component 2. Abbreviations see inTab. 1.
Table 1. The contribution of each physiological and morphological parameters in each principal component of two genotypes (4, and 19) of Stevia rebaudiana evaluated at 30- and 60-days (physiological and morphological parameters, respectively) after the start of salt stress at 0 M, 0.28 M, 0.82 M, 1.65 M, 2.74 M, and 4.1 M NaCl.
Variable | PC1 | PC2 | PC3 | PC4 | PC5 | PC6 | PC7 | PC8 | PC9 | PC10 |
---|---|---|---|---|---|---|---|---|---|---|
P N | 0.326 | -0.149 | -0.002 | 0.147 | 0.010 | -0.136 | -0.236 | -0.384 | -0.312 | -0.221 |
g s | 0.273 | -0.296 | 0.136 | -0.092 | -0.077 | -0.374 | 0.256 | -0.252 | -0.232 | -0.239 |
T | 0.262 | -0.218 | 0.315 | -0.005 | -0.092 | 0.147 | 0.397 | -0.022 | 0.536 | 0.288 |
WUEi | 0.298 | 0.128 | -0.189 | 0.294 | 0.056 | 0.512 | 0.005 | 0.120 | -0.125 | -0.210 |
Tleaf | 0.300 | -0.084 | -0.312 | -0.011 | 0.050 | 0.053 | 0.158 | -0.119 | 0.052 | -0.164 |
Ci:Ca | 0.178 | -0.334 | -0.290 | -0.211 | 0.398 | -0.046 | -0.266 | -0.151 | -0.009 | 0.588 |
VPD | 0.251 | -0.085 | -0.423 | -0.007 | 0.170 | 0.240 | 0.290 | 0.039 | 0.116 | -0.085 |
ETR | 0.241 | -0.305 | 0.272 | -0.108 | -0.157 | -0.047 | -0.169 | 0.089 | 0.322 | -0.300 |
FPSII | 0.297 | 0.208 | -0.012 | -0.095 | 0.280 | -0.394 | 0.196 | 0.636 | -0.080 | -0.097 |
ETR/ P N | 0.101 | -0.451 | 0.252 | 0.118 | -0.204 | 0.349 | -0.153 | 0.432 | -0.426 | 0.173 |
Leaf DW | 0.225 | 0.321 | 0.275 | 0.172 | -0.023 | -0.024 | 0.369 | -0.187 | -0.341 | 0.405 |
Shoot DW | 0.228 | 0.285 | 0.282 | -0.226 | 0.233 | 0.242 | -0.357 | -0.117 | 0.164 | -0.148 |
Root DW | -0.125 | -0.194 | 0.238 | 0.608 | 0.612 | -0.166 | -0.072 | 0.056 | 0.135 | -0.063 |
Total biomass | 0.233 | 0.305 | 0.301 | -0.065 | 0.169 | 0.148 | -0.104 | -0.149 | -0.023 | 0.049 |
RSR | -0.322 | -0.143 | 0.070 | 0.132 | 0.166 | 0.252 | 0.349 | -0.249 | -0.036 | -0.191 |
HI | 0.212 | 0.155 | -0.204 | 0.577 | -0.403 | -0.207 | -0.223 | -0.035 | 0.273 | 0.168 |
Abbreviations: P N: net photosynthesis. g s: stomatal conductance. T: transpiration. WUEi: intrinsic water use efficiency. Tleaf: leaf temperature. Ci:Ca ratio: internal-to-ambient CO2 concentration. VPD: vapor pressure deficit. ETR: electron transport rate. FPSII: actual PSII efficiency during the exposure to sunlight. ETR/P N xg s: Relationship between the ratio of ETR and P N versusg s. Leaf DW: dry weight of leaves. Shoot DW: dry weight of shoot. Root DW: dry weight of roots. Total biomass: biomass total of shoot plus root. RSR: root : shoot ratio. HI: Harvest index.
DISCUSSION
Gas exchange and chlorophyll a fluorescence parameters
Salt stress usually decreases photosynthesis, but these effects vary with dose and species. However, conflicts in the debate on the relative importance of diffusive and metabolic factors to the overall control of photosynthesis even under mild environmental stress still arise (Hussin et al., 2017; Mendes et al., 2017; Pinho-Pessoa et al., 2018; Debnath et al., 2019; Dos Santos et al., 2019; Silva-Santos et al., 2019; Pan et al., 2021). The plant responses to salt stress include adaptive and/or deleterious changes, where these stress has a negative effect on carbon assimilation through reduction in P N, which results in plant growth reduction (Dos Santos et al., 2019). The ETR/P Nreflects the energy transfer to the photosynthesis. Under optimum conditions, P N is the main sink; however, under stress P N is more sensitive and decreases faster than ETR, promoting the ratio increase and indicating an energy impairment that can be associated to ROS production (Fonseca-Pereira et al., 2019). This is an indication that alternative electron sinks were increased due to the stressful conditions (Cerqueira et al., 2019). This combined with high irradiance can lead to the loss of coordination between light reaction and Calvin cycle due to insufficient supply of the electron and proton acceptor (CO2). Inevitably, this would result in a higher ratio between the electrons generated in the photosynthetic electron transport chain and the ones consumed by photosynthesis and respiration (ETR/P N) (Fonseca-Pereira et al., 2019).
In this study, we showed that, P N was not significantly modulated by salinity (r = -0.0981). Even a slight reduction occurred concomitantly after an increase of [NaCl]. However, P N was strongest and positively correlated to WUEi (r = 0.999), and g s (r = 0.758), moderately and positively correlated to ETR (r = 0.514), and T (r = 0.479), and weekly and positively correlated to total DW (r = 0.350), SDW (r = 0.331), and LDW (r = 0.284). In another way, P N was moderately and negatively correlated to VPD (r = -0.533) (Annex). Similar results were reported by Hussin et al. (2017) who noticed that low NaCl concentrations (up to 0.025 M) promoted the growth of S. rebaudiana seedlings, while higher water salinity (0.05 M) significantly suppressed their growth.
Variation in Tleaf is often linked to leaf water potential under stress conditions (Pompelli et al., 2010; Hsie et al., 2015; Mendes et al., 2017; Pinho-Pessoa et al., 2018; Debnath et al., 2019). In comparison with the non-saline plants, the stressed ones showed marked increase in Tleaf, which was more pronounced in the G19 than G04, indicating possible photosynthesis damage in G19. It is worth noting that Tleaf is strongly correlated to VPD (r = 0.600), and the increase of VPD, driving to salt stress (r = 0.392), lead to the strongest fall in all gas exchange parameters, like as P N (r = -0.533), g s (r = -0.434), T (r = -0.538), and WUEi (r = -0.533).
To date, most research is concentrated around stomatal limitation to photosynthesis (Flexas and Medrano, 2002; Chaves et al., 2008; Batista et al., 2012; Pan et al., 2021), with nonstomatal limitations receiving much less attention. If g s and Ci decrease as P N decrease and cause a decrease in stomatal limitation value, the stomatal limitation is dominant. Notwithstanding, if Ci increase as g s and P N decrease, the nonstomatal limitation should be the dominant one, as previously reported in this species. Hussin et al. (2017) described that the reduction of P N is largely regulated by g s in plentiful discordance to our results that clearly indicate a nonstomatal limitation of P N. A parallel decline in P N and g s (r = 0.758) indicate that limitation of photosynthesis under hyperosmotic conditions in S. rebaudiana plants might be, to some extent, due to a stomatal reason, restricted by stomatal closure and subsequently substrate (CO2) deficiency. Notwithstanding, the reduction of P N cannot be related to the decrease in the acquisition of CO2 by the stomatal closure since the stomata can remain open at moments of lower VPD (Hsie et al., 2015). Rather, this idea is supported by the fact that the Ci:Ca ratio was strongly increased with the salt concentration in G19 (r = 0.910; P<0.0001) and was more abrupt at the lower salt concentrations (which is expected to largely overcome limitations to CO2 diffusion throughout the mesophyll).
The fact that under salt stress P N has been reduced to a greater extent than ETR is an indication that alternative electron sinks were increased due to the stressful conditions caused by salinity (Hussin et al., 2017; Cerqueira et al., 2019; Fonseca-Pereira et al., 2019). This pattern led us to speculate that the reduction of P N should be attributed rather to nonstomatal limitations than to stomatic ones. However, Hussin et al. (2017) describe that in S. rebaudianaP N was substantially reduced in response to salinity without concomitant changes in ETRmax, a fact that is in plentiful discordance with our data, where the ETRmax showed in this study was 30 μmol electrons m-2 s-1, less than 50% of smallest ETRmax described by Hussin et al. (2017). Lastly, high Ci:Ca ratio could indicate that the photosynthetic decline was nevertheless due to salt-induced reduction in the mesophyll conductance, rather than to stomatal effects. These findings confirm our hypothesis that stomatal closure was not the main reason for decreased photosynthetic rates in response to substrate salinity and was in accordance with P N/Ci curves (Centritto et al., 2003).
The an efficient mechanism for dissipating excess light energy and thus minimizing ROS generation seems plausible, especially in the light of the studies by Acosta-Motos et al. (2019) and Cantabella et al. (2017). This is particularly meaningful in Colombian Caribbean region, where active radiation can go up to 2,200 μmol photons m-2 s-1 (Jarma-Orozco et al., 2020). To further elucidate the physiological performance of the genotypes to the salt stress, here we compared the relationship between the ETR and P N versus g s. We showed that in 30-d S. rebaudiana stressed plants a strong reduction was seen of 50%, and 24% in P N while strongest ETR decrease by 80%, and 86% in G04, and G19, respectively (Fig. 1A, and 2A). With the steeper reduction in ETR than P N, the ETR/P N decreased too, promoted by an increase of salinity (r = 0.387). So, the relationship between ETR/P N vs.g s was slightly and significantly increased, indicating that alternative electron sinks were increased due to the salt stress (Fig. 3). However, this pattern was genotype-dependent, because in the G04 these relationships were increased (r = 0.281; P< 0.0001), while in G19 these relationships were decreased (r = -0.160; P< 0.0001) (data not showed). Thus, the largest ETR/P N vs.g s relationships values were observed in the G04, and the lowest were in the G19 (Fig. 3). This strongly suggests that there must be an alternative pathway for sustaining electrons coming from PSII in non-assimilatory electron flow (e.g., photorespiration and the Mahler-peroxidase) in S. rebaudiana plants exposed to salinity (Osmond et al., 1997).
In vivo studies of the effects of NaCl on Chl fluorescence in Synechococcus cells showed that incubation of cells in the presence of NaCl did not damage QA, pheophytin, and P680 but blocked the transport of electrons from water to P680 probably due to the increase of reducing power in the system and low reoxidation of NADP+ (Yu et al., 2018). This inhibition leads to the accumulation of NADPH that can induce excitation pressure and hence the generation of ROS in PSI. Even though strong light inhibited the repair of the photodamaged PSII, implying that a combined effect of salt and high light intensity on photosynthetic machinery has a synergistic action on PSII activity (Pan et al., 2021), we argued that this has not occurred in the plants of S. rebaudiana, since they were grown under greenhouse when measuring gas exchange parameters, no more than 90% of the theoretical saturating light was applied (Jarma-Orozco et al., 2020).
Growth parameters under salinity
In this statement, we showed that the effects of salinity on biomass production were much stronger between the 0.28 and the 4.1 M (reduction of 82.7% for G04, and 85.7% for G19) than during the non-saline and 0.28 M NaCl (reduction of 63.9% for G04, and 14.6% for G19) (Fig. 4, and Infographic). However, our data were in plentiful discordance to Reis et al. (2015) that which classified S. rebaudiana as moderately sensitive to moderately tolerant, or as not highly tolerant to salinity. These authors argued that this hypothesis is based on the yield that was relatively tolerant to the irrigation water salinity at salinity threshold of 2 dS m-1, equivalent to 0.03 M, obtained by us with a linear regression (r = 0.9947; P = 0.0005). So, our data may classify the S. rebaudiana plants as a tolerant to highly tolerant plant, because the main P N or biomass production was slightly reduced until 0.28 M NaCl, equivalent to 13 dS m-1 or 6- to 7-fold more saline than threshold salinity level considered to Reis et al. (2015). For the sake of clarity, the C50 (González et al., 2015) calculated from G04 and G19 was 0.263 M (11.6 dS m-1), and 0.458 M (19.8 dS m-1) for G04 and G19, respectively (Fig. 1).
In the early growth stage, there is an obvious reduction in aerial and root dry matter formation mainly from leaves in G04 in comparison to G19. Cantabella et al. (2017) described that at the end of the salinity period, no effect of NaCl stress on plant biomass was observed in S. rebaudiana plants, and, in fact, a significant increase in LDW was even detected. Hussin et al. (2017) described that more biomass was allocated to the shoots as compared to roots as shown by the increase in the shoot : root fresh weight ratio in response to low NaCl salinity levels. Although this issue, at first glance, is in contrast to our data, data presented by Hussin et al. (2017) cannot be compared with our data, since the ratio calculated by those authors was based on fresh weight, because salt stress implies in a lower water potential. Thus, comparing the effect of salinity using fresh weight is a wrong interpretation that does not consider the plant physiology and the forces that govern water flow between environments of different osmotic potentials (Holbrook, 2015).
Cantabella et al. (2017) suggested that S. rebaudiana plants can deal with short- and long-term NaCl stress, displaying a series of mechanisms to acclimate to salinity, including ion homeostasis, modified growth parameters, photosynthesis protection, proline accumulation, and antioxidative metabolism related changes. It highlights that in their study Cantabella et al. (2017), applied 25-d of irrigation water plus salt at 5 g L-1 or 0.08 M, the [NaCl] is at least 4-fold lower than our lowest salt concentration (0.28 M NaCl) and 51-fold lower than our highest salt concentration (4.1 M NaCl).
Debnath et al. (2019) studying the effect of 100 mM NaCl on 4-weeks salt-stressed Shoutian-2 cultivar of S. rebaudiana the plant height, total biomass, SDW, LDW, stem DW, and RDW were reduced by 63, 73, 76, 75, 84, and 61%, respectively. Elsewhere, in the Fengtian cultivars the reductions were 25, 63, 65, 67, 46, and 49%, showing that the effect of salt stress is cultivar-dependent. However, the data showed in figure 4, demonstrated that both genotypes were negatively influenced in a similar manner to salt stress, and that G19 shows a more acute reduction in its harvest index than G04. Moreover, the loading plot graph shows that RDW and RSR did not have any effect to distinct the G04 than G19, while SDW and ΦPSII seem to be more favorable to separate G04 than G19, while all physiological parameters seem to be more favorable to separate G19 than G04.
Some scholars have described that S. rebaudiana plants had a robust salt stress defense system, that includes a decrease in growth and biomass production, chlorophyll bleaching, evolution of the chlorophyll fluorescence parameters, and a large increase in antioxidant enzyme activities (Zeng et al., 2013; Cantabella et al., 2017; Acosta-Motos et al., 2019; Debnath et al., 2019). Taken together, these physiological responses combined with an increase of 17-42-fold in proline content and an increase of 2-6-fold in ETR in salt-stressed S. rebaudiana plants (Hussin et al., 2017; Acosta-Motos et al., 2019), led us to speculate that S. rebaudiana seems to be a tolerant plant instead of a moderately or sensitive plant as reported previously. The data presented in this study also allow us to argue that even in extreme salt conditions, the reduction of physiological and morphological parameters due to salinity must be strictly linked to nonstomatal and metabolic changes than to the limitations to the CO2 flux by stomatal closure.