1. Introduction
Sequential batch reactors (SBR) consist of one or more reactors used for sequential equalization, aeration, and clarification. The operating cycle of a typical SBR system consists of five steps, (1) fill: the reactor is loaded with wastewater; (2) react: air is supplied to the reactor for the growth of microorganisms and the biological oxidation of organic compounds; (3) settle: solids settle, allowing clarification of the liquid; (4) draw: clarified liquid and sediment or sludge are drained from the reactor as separate streams; (5) idle: this step is used when there are two or more reactors in the system and one remains empty while the others are full[1]. SBR constitute a versatile technology in the treatment of wastewater that continues gaining importance in the elimination of pollutants [2]. Due to their simple configuration, they have been used efficiently to treat domestic wastewater (DW), especially where they have proven to be efficient in eliminating organic compounds and solids. Besides, some studies indicate that the SBR concept is a viable alternative and economically attractive for the conventional continuous-flow activated sludge process in eliminating biological oxygen demand, suspended solids, and nitrogen and in the chemical precipitation phosphorus [3].
The conventional activated sludge process is the most widely used biological process for treating municipal and industrial wastewater. This process consists of a reactor called the aeration tank, a settling tank, solids recycled from the settler to the aeration tank, and a sludge wasting line [4]. In contrast, the SBR system performs all those functions in only one tank distinguishing by the enforcement of controlled short-term unsteady state conditions leading in the long run to a steady state for composition and metabolic properties of the microbial population growing in the reactor by controlling the distribution and physiological state of the microorganisms [5].
The effectiveness of an activated sludge process depends on aerobic metabolism, which often requires intensive mechanical aeration [6]. To promote the aerobic biochemical reaction, the rate of oxygen supply to the microorganisms must be rapid, then the oxygen supplier must be highly efficient. Microbubbles are bubbles of 10 to 60 µm in diameter with a big gas-liquid interface area that, with a long residence time in the liquid phase, improve the dissolution of oxygen gas from the air in water. Venturi tube is a kind of hydrodynamic cavitation microbubble generator; In this device, milli-bubbles are injected into the liquid stream that flows from the inlet of the Venturi tube, and when the two-phase flow accelerates through the throat of the tube, the dynamic pressure changes rapidly, forming microbubbles by reducing milli-bubbles and/or cavitations [7]. Venturi nozzle-type generators do not require air injection equipment since they suck air through the neck of the nozzle due to the pressure drop produced by the passage of the working fluid through the Venturi [8].
The recirculation parameter strongly influences the overall performance of the process in a biological system due to affecting the microbial ecology, hydraulic regime, and operating characteristics of the system by directly controlling the biochemical system. For instance, recirculation of the treated wastewater to mix with the influent wastewater provides a method to balance the hydraulic head with the carbon load [9]. Moreover, well-mixed reactors provide uniform conditions for microbial growth by increasing the active area in the reactor and absorbing sudden organic overloads, reducing the effects of acidogenic bacteria.
An efficient way of mixing a fluid without the use of mechanical devices is to use the jet generated in the inlet pipe to the tank; for this, it is preferable a turbulent flow (Reynolds number greater than 3000), in order not to waste its energy and not dissipate it when hits the walls or deflectors [10].
This work describes the design, construction, and evaluation of a laboratory-scale prototype of a sequential discontinuous reactor with recirculation and aeration through a Herschel-type Venturi tube coupled to a centrifugal pump. The objective was to use the Venturi as an aeration device and recirculation as a mixing and homogenization system, that uses the flow energy to ensure uniform mixing in the tank and an adequate supply of oxygen to the system. In the evaluation of the prototype, the effect of the Venturi position with respect to the reactor wall and its influence on the performance of the reactor to eliminate the organic matter were studied.
2. Materials And Methods
2.1 Analytical procedures
The measurements of biological oxygen demand (BOD5) were performed by the azide modification iodometric method [11] and those of chemical oxygen demand (COD) by the closed reflux method and titration [12], using the 0.10 N dichromate allowing the performance of reactors to be monitored. These are the methods used by the laboratory of the Instituto de Hidrología, Meteorología y Estudios Ambientales (IDEAM), homologous to the 5210 B and 5220 C procedures of the Standard Methods [13]. The total suspended solids were determined as the difference between total solids and total dissolved solids according to procedures 2540 B and 2540 C of the Standard Methods [13], respectively. The reagents used in the implementation of these methods are analytical grade. Hydrogen potential (pH), dissolved oxygen, and temperature measurements were done with a multiparameter probe Hach HQ40D.
Data treatment
The Q rejection test with a 99% confidence level [14] was applied over three replicates of samples for the BOD5 and COD of each reactor. The mean and standard deviation are calculated with the non-rejected data by the test. In each reactor, the Venturi was at a characteristic distance from the reactor wall. Therefore, each reactor and its evaluation are an experimental treatment part of a simple comparative experiment of treatment pairs with inferences about equality in means and variances of the response variable, reduction efficiency of BOD5 using an α of 0.05.
Experimental apparatus
Figure 1 presents the schematic of the sequential discontinuous reactor prototype and the section with the dimensions and construction details of the Herschel Venturi tube [15]. The dimension d 1 indicates the distance between the Venturi discharge point and the reactor wall that represents the experimental variable, taking values of 0.156 m (Reactor 1, R 1 ), 0.117 m (Reactor 2, R 2 ), 0.078 m (Reactor 3, R 3 ), and 0.039 m (Reactor 4, R 4 ). The reactor walls and the Venturi material are high-density polyethylene (HDPE); the piping and accessories are polyvinyl chloride (PVC); the gaskets are synthetic rubber. The type of the pump is centrifugal (Bayite BYT-7A015 DC 12V).
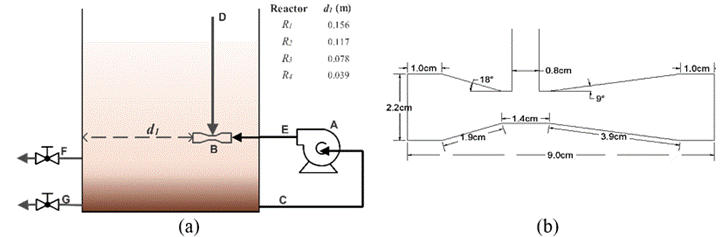
Figure 1 (a) Schematic of the discontinuous sequential reactor prototype, A: centrifugal pump; B: Venturi tube; C: pump suction; D: air suction; E: pump discharge; F: clarified liquid drainage; G: sludge purge; d 1: distance from the Venturi to the reactor wall. (b) Cut with the dimensions and construction details of the Herschel Venturi tube
The reactor is a cylindrical tank 0.27 m in diameter and 0.35 m high, in which the suction of the pump (C) is located one centimeter from the bottom of the reactor, and the discharge (E) is 5.9 cm from it and 5.0 cm from the liquid level. The design specifies the oxygen supply in 1.25×107 mg/L at a pressure and temperature (average) of 1 atm and 21 °C, respectively, during the operation period reactor, maintaining a discharge rate of the recirculated liquid through the Venturi of 0.417 m/s. A pre-programmed timer EXCELITE 1650w controlled the operation sequence of the SBR.
2.2 Substrate
Table 1 presents the composition of the substrate or synthetic wastewater used in the evaluation of the prototype [16]; this simulates domestic wastewater with a COD of 130 mg/L and guarantees the appropriate nutritional requirements for the growth of the microorganisms.
2.3 Inoculum
The inoculum was obtained from domestic wastewater discharge with pH 7.07 and COD of 110.8 mg/L that proportioned a source of local microorganisms.
2.4 Experimental procedure
The experiment simultaneously operated four models of the designed SBR prototype, each with the Venturi located at one of the previously indicated distances d 1 . At the operation beginning, each reactor was loaded with 3.2 L of the substrate (synthetic wastewater) and inoculated for a single time with 4.8 L of inoculum. The operation cycle has a duration of 24 h, during which the reaction time (2.5 h) alternates with the settlement period (1.5 h) controlled by a digital program timer. After the last settlement period ended, 3.2 L of the clarified liquid was removed (0.05 h). When restarting the operation cycle, the volume of each reactor (8 L) was completed by adding 3.2 L of the fresh substrate (0.005 h), which mixed with the sedimented sludge at the end of the previous operation. This activity was repeated over a month; for each reactor, the temperature, pH, and dissolved oxygen were registered, and every eight days, a sample of clarified liquid was taken to determine BOD5, COD, and solids suspended total (SST). Although each stage of the SBR operates in a transitory state, at the operating cycle end, some of their properties reach stable values. The steady state condition was defined when the performance parameters of the reactors have a variation below the 10% [17].
3. Results and Discussion
The total suspended solids reflect the biomass growth, which in the settlement stage are concentrated and deposited in the bottom of the tank and remain in the reactor, while drained the clarified liquid; these solids are retained and accumulated throughout the month of operation, this time corresponding with the solids retention time (SRT). Visual inspections of the operation showed that the recirculation system effectively distributed the concentrated solids after settlement periods in all reactors. Additionally, patterns of deposition of solids in the bottom of the tank allow inferring that in reactors 1 and 2, the solids reach a more homogeneous distribution. In these two reactors, the position of Venturi with respect to the wall presents a great distance.
During the operation of the reactors, the temperature in each one registered as an indicator of the environmental conditions, Figure 2 shows the temperature control chart, it indicates an average of 21.1 °C with a standard deviation of 1.1 °C, Furthermore, during the operating time the oscillation did not exceed the three standard deviations. In 80% of the time, the variations remained within the two standard deviations. The average operating temperature of the reactors is outside the optimal range reported for the development of bacterial activity, which is 25 to 30 °C [18]. However, this was not a limiting factor for satisfactory biomass growth, probably because the inoculum comes from a local environment of 22 °C.
Figure 3 shows the control chart for pH and dissolved oxygen. The pH had a mean value of 6.7 ± 0.5, and during all the experiments, its variation did not exceed two standard deviations, and 85% of the time was less than one standard deviation. The graph also shows that the pH value tends to be below the average after day twelve. Considering that most bacteria tolerate pH values lower than 9.5 and higher than 4.0 [18], the values obtained during the experiment are adequate.
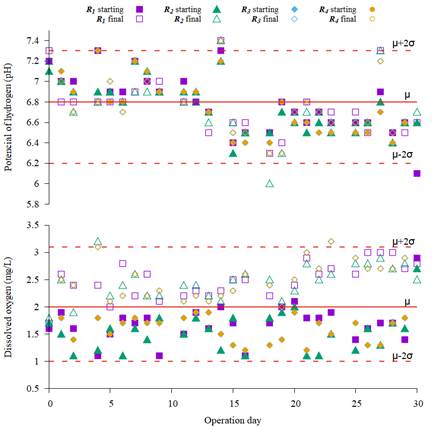
Figure 3 Control graph of dissolved oxygen and hydrogen potential during the operation of the four reactors
Table 2 Efficiency in the reduction of oxygen demands in the clarified liquid
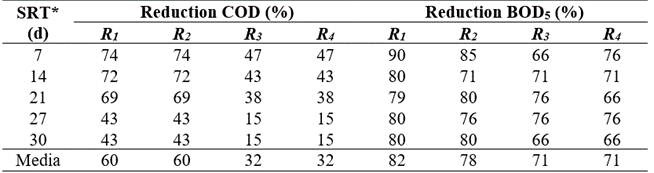
* SRT: Solids retention time.
The mean value of the dissolved oxygen concentration in the reactors was 2.0 mg/L, with a standard deviation of 0.5 mg/L, and all the data registered are within the two standard deviations. Standard deviation, given the magnitude of the mean value, is significant. However, the behavior in oxygen dissolved control chat shows that data taken at the beginning of the operation have an average concentration of 1.6 ± 0.3 mg/L, consistent with the stop in recirculation and therefore in aeration. While data of the end of the operation cycle, with an average concentration of 2.5 ± 0.4 mg/L reflects the effect of aeration through the Venturi and which is greater than the 2.0 mg/L value recommended for aerated biological systems [19].
Table 2 presents the efficiency in oxygen demands reduction in the clarified liquid. In reactors 1 and 2, the reduction in COD is higher; also, this reduction is more significant at solids retention times less than 21 d in all cases. The reduction in BOD5 of reactors 1 and 2 stabilizes around 80% when SRT is greater than 21 d; in reactors 3 and 4, it stabilizes around 66%.
Table 3 presents the results of hypothesis tests for the reduction of BOD5 in the evaluation of the four models of the SBR prototype. The application of the t-test implies that the samples are from independent populations described with a normal distribution and with equal standard deviations [20]. Figure 4 shows the cumulative probability graph to efficiency in BOD5 reduction; in the chart, a straight line adjusted the data of all reactors, which allows corroborating that these belong to a normal distribution and validates the application of the t-test and the use of the F distribution for the test of variances.
Hypothesis tests indicate no significant difference in the BOD5 reduction efficiency data variance, which allows us to affirm that the dispersion effect is the same in all reactors or that their sources produce the same effect. Furthermore, a comparison of the means shows that they do not significantly differ between rectors 1 and 2 or between reactors 3 and 4. Thus, for each of these two pairs of reactors, the position of the Venturi with respect to the wall of the reactor has the same influence on the performance of the reactor to remove organic matter.
Table 3 Results of hypothesis tests for the reduction of BOD5 in the evaluation of the four models of the SBR prototype
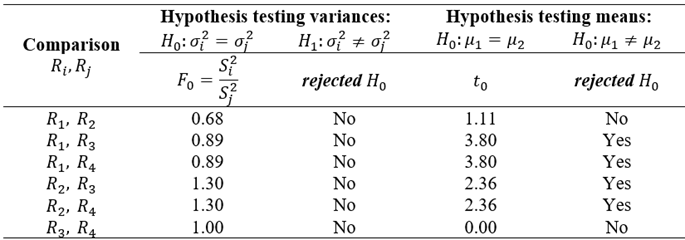
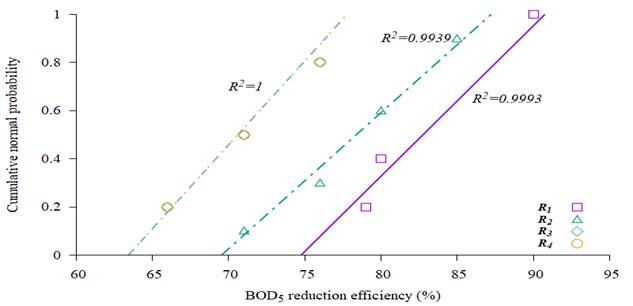
Figure 4 Cumulative normal probability plot for the BOD5 reductionn efficiency in evaluating the SBR prototype models
Figure 5 presents the results of the operation of the reactors as a function of the solids retention time (SRT). Chart a shows two trends in the COD of the clarified liquid, one followed by reactors 1 and 2, and another by reactors 3 and 4; in both cases, the increment of COD is attributable to the soluble microbial products since the BOD5 values throughout evaluation period were significantly lower than the initial BOD5 value (chart b). The final COD indicates that the final BOD5 is not the most significant contributor to the COD, as evidenced by the biodegradability factor
that goes from 0.72 in the original substrate to 0.25 in the clarified liquid of reactors 1 and 2, and 0.29 in reactors 3 and 4 (chart c). Furthermore, total suspended solids present a decreasing behavior in all reactors (chart d), which shows that they are not the cause of the increase in COD. Besides, this is evidence of the increment of biomass settleability.
The decrease in BOD5 during the SBR operating cycle reflects the consumption of the substrate. Substrate utilization rate presents an average value for reactors 1 and 2 of 49.2 ± 3.2 mg/L·d and in reactors 3 and 4 of 43.3 ± 2.8 mg/L·d, the average variation of this parameter in the evaluation period of the reactors was 6.5%. Therefore, the consumption of the substrate was done in a stable state. Figure 6a presents the substrate utilization rate (r ut ), calculated according to Equation (1) for the different consecutive SRT (17, 14, 21, 27, and 30 d), and shows its correspondence with the BOD5 obtained at the end of the operating cycle.
where, S 0 and 𝑆 are the BOD5 at the cycle starting and end, respectively. θ is the duration of the operating cycle (1 d).
The substrate consumption in the reactors had modeled according to Equation (2). Figure 6b presents the evolution of the substrate concentration expressed as BOD5 in the evaluated reactors, each curve corresponding with Equation (3), which is the result of integrating Equation (2) with the data from each reactor.
where, S1 is the BOD5 after a time of operation ɵ (in this case,
).
4. Conclusions
The Herschel-type Venturi tubes used as aeration devices allowed supplying 1.25×107 mg/L of oxygen (calculated, 1 atm and 21 °C) during the operation reactors, sufficient to maintain an average dissolved oxygen concentration of 2.0 mg/L.
The observations during the evaluation of the prototype allowed inferring that reactors 1 and 2 present a more homogeneous distribution of solids in the recirculation period, and the data show that these reactors have higher removal of organic matter. Therefore, considering that the level of oxygenation in all reactors was adequate for the aerobic process, it must be explored the existence of an optimal limit distance for the location of the Venturi.
The BOD5 reduction efficiency was higher than 80%, while the COD reduction efficiency was around 60%. Therefore, it was possible to establish that the main contribution to COD is due to soluble microbial products. Hence, the decrease in the biodegradability factor is the result of the action of microorganisms. Besides, the increase of solids retention time evidenced the increase in biomass settleability, which positively contributed to SBR operation.
These results support the implementation of the mixing and aeration by recirculation through a Venturi in applying the sequential batch reactor as a technology for wastewater treatment, avoiding moving parts inside the reactor.