Tropical forests store about 55% of the total carbon (C) present in terrestrial ecosystems (Pan et al. 2011); thus, they significantly contribute to the dynamics of the C cycle and to the mitigation of global climate change (IPCC 2014; Morrison-Vila et al. 2021). This C is stored mainly in the biomass (above and below ground) of trees and in the soil (Pan et al. 2011). For this reason, interest has increased in determining forest C stocks (Pugh et al. 2019) and revealing the influence of environmental and anthropogenic factors that determine its dynamics (Poorter et al. 2016), in the context of the problem of global warming.
Belowground biomass (fine and coarse roots) represents more than 25% of the total biomass of tropical rain forests (Cairns et al. 1997; Saugier et al. 2001), so they are fundamental in the functioning of the ecosystem (Chapin III et al. 2002). In particular, fine roots (<2.0 mm in diameter) are important for the acquisition, transport, and accumulation of water and nutrients from the soil (Hendricks et al. 1993; Brearley 2011); furthermore, they can store between 3 and 120 t ha-1 in tropical forests (Vogt et al. 1986). Therefore, they can be considered as a significant sink and reservoir of atmospheric C. However, it has been shown that the biomass of fine roots (FRB) is determined by factors such as the type of ecosystem (Cairns et al. 1997), the basal area (Finér et al. 2011), climatic conditions (Roy and Singh 1995), soil fertility (Powers et al. 2004; Powers and Peréz-Aviles 2013), the dynamics of disturbances and forest clearings (Ostertag 1998), the type of predominant tree species (Valverde-Barrantes et al. 2007), the availability of water and nutrients (Cavelier et al. 1999; Espeleta and Clark 2007; Brearley 2011), rates of mineralization, nitrification, ammonification, and total availability of nitrogen (N) (Singha et al. 2020) and the time (age) of succession (Hertel et al. 2006; Quinto et al. 2013; Singha et al. 2020), which indicates that the variation in these factors affects the C reserves of the ecosystem, and its function in the face of climate change.
Controversially, some studies evaluating the influence of soil fertility and successional age on FRB show contrasting trends. Indeed, in some cases a negative correlation has been shown between soil nutrients (especially N) and FRB (Brearley 2011; Powers and Peréz-Aviles 2013; Singha et al. 2020), while other studies do not show such correlations (Quinto et al. 2016a), and cases have even been reported in which fine roots proliferate within nutrient-rich patches (Roy and Singh 1995). On one hand, in the studies that evaluate successional processes, two contrasting tendencies have been evidenced. On the other hand, Deans et al. (1996); Hertel et al. (2006); Quinto et al. (2013) observed significant increases in FRB with increasing successional age, while the analyzes of Power and Peréz-Aviles (2013) and Brearley (2011) show that the FRB is poorly determined by age or time of succession in tropical forests, apparently because FRB recovers early in succession or very quickly after disturbances (Brearley 2011; Power and Peréz-Aviles 2013; Quinto et al. 2013). Recently Singha et al. (2020) observed that the FRB was positively associated with the time (age) of succession; but, with the total N content of the soil, the relationship was negative, which also increased with the age of the ecosystem. Thus, the lack of consensus and current debate on the influence of nutrients on FRB in tropical successional ecosystems is evident.
Assessing these patterns is fundamental because, although FRB represents a considerable portion of total C of the forest (Jackson et al. 1997), it plays a crucial role in plant nutrition, absorption, and recycling of minerals, soil enrichment with organic matter, and ooverall ecosystem productivity (Singha et al. 2020). Particularly tropical forests that have suffered in recent years from strong effects due to human activities such as deforestation, degradation, Selective logging, forest fires, open-pit mining, among others (FAO and PNUMA 2020), it is essential to understand the changes of the FRB in the different successional stages; because with the passage of time, soil conditions change (Guariguata and Ostertag 2001); and with this, it is likely that the influence of edaphic nutrient content on the stocks and recovery of belowground biomass will also vary; therefore, it is necessary to evaluate the relationship that soil physicochemical parameters have on FRB in different successional stages, with the aim of contributing to the ecological and functional restoration of degraded tropical ecosystems.
In particular, open-pit mining of metals such as gold has become one of the main drivers of deforestation and degradation of tropical forests (Primack and Vidal 2019; Kalamandeen et al. 2020). It is estimated that between 2001 to 2013, around 1,680 km2 of tropical forests in South America were lost (Primack and Vidal 2019); of which 41% corresponds to mining carried out in Guyana, 28% in the Southwest of the Amazon, 11% in the Tapajos-Xingú forests in Brazil, and 9% in the Magdalena basin in Colombia (Álvarez-Berrios and Aide 2015). Between 2010 to 2017, it is estimated that between 57,000 and 60,000 ha of natural forests were lost to gold mining in Guyana and Perú, respectively (Kalamandeen et al. 2020). A particular case has been recorded in Colombia, in the state of Chocó (Colombian Pacific), where in 2015 licenses were granted for the mining exploitation of 302,381 ha of natural forest, representing 6.49% of the territory (Tierra Digna 2016); with which, not only the biodiversity of the region is threatened, but it also affects the carbon content stored and the capacity of ecosystems to mitigate global climate change (Quinto et al. 2013).
Chocó biogeography is one of the rainiest regions in the world, with places that have rainfall levels higher than 10,000 mm per year (Poveda et al. 2004). In this region, open pit gold mining generates the deforestation and degradation of more than 360 ha of forest annually (IIAP 2001; Ramírez and Ledezma 2007). Due to this, these ecosystems offer us an opportunity to evaluate the aforementioned hypotheses about the influence of soil fertility and successional age on the FRB in areas degraded by open pit mining. In this sense, in this investigation the following questions were raised: How does the FRB vary as a function of the time of succession, in areas degraded by mining in these high rainfall tropical ecosystems? How much do the edaphic conditions, especially the contents of nutrients, determine the FRB of areas degraded by mining with different successional stages? In areas degraded by mining, which factor has a greater influence on the variation of the FRB, soil fertility or successional age? Even more so if one considers that, according to Austin and Vitousek (1998), with high precipitation the nutrient content tends to decrease due to runoff and leaching. For this reason, the objective of evaluating the biomass of fine roots and its relationship with the soil in areas degraded by mining in the biogeographical Chocó (Colombia) was established.
MATERIALS AND METHODS
Study area
This study was carried out in forested areas previously degraded by open-pit gold mining, in the locality of Jigualito (5°06'01" N - 76°32'44" W), municipality of Condoto, in the Colombian Pacific (Figure 1).
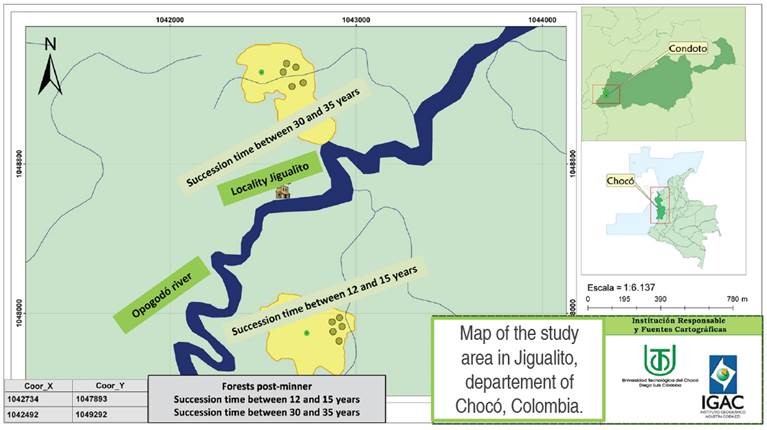
Figure 1 Map of the study area in the town of Jigualito (municipality of Condoto), department of Chocó, Colombia. Modified from Quinto et al. (2022).
This has an average rainfall of 8,000 mm per year, an altitude of 70 masl and flat topography. This locality is part of the north central Chocó biogeographical subregion, which includes the upper basins of the Atrato and San Juan rivers, in Piedemonte and Colinas low landscape units with humid terraced soils and with a type of transitional sedimentary rock (Poveda et al. 2004). The forests are mostly secondary, and with different ages of recovery due to the fact that mining has been carried out in the area at different times (Figure 2).
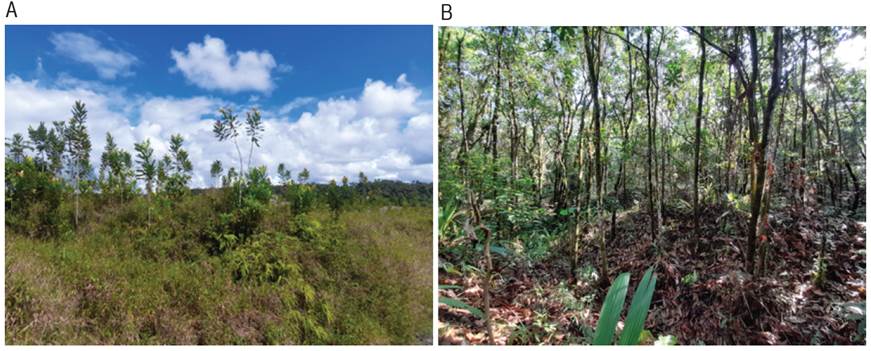
Figure 2 Forested areas previously degraded by open-pit gold mining, in the locality of Jigualito, municipality of Condoto, in the Colombian Pacific. Where A. are areas with a succession time of between 12 to 15 years. B. Are areas with a succession time of between 30 to 35 years.
The soils are ultisols, but due to mining they were characterized by a lot of rocky material and sand. In addition, they are acidic and have high contents of OM, total N, available P, Al and clay; while the concentrations of Ca, K, Mg, CICE and silt are very low in areas of recent mining activity, but their content is higher in areas with more recovery time (Ramírez et al. 2019). On the other hand, the soils of the forested areas surrounding the mines present extreme acidity, clayey with high contents of Al, OM and total N, and low amounts of P, Mg and Ca, likewise, the K contents are intermediate and the CICE is low (Quinto and Moreno 2016).
In the mature forest, the most abundant tree species were Wettinia quinaria, Mabea occidentalis, Eschweilera sclerophylla, Vismia sp., Inga sp., Pourouma chocoana, Vismia macrophylla, Matisia sp., Protium apiculatum, Couepia platycalyx, Miconia sp., Sloanea grandifora, Sloanea fragrans, Anaxagorea crassipetala, Humiriastrum melanocarpum, Faramea jefensis, Cespedesia spathulata, Chrysochlamys clusiifolia, and Brosimum utile (Ramirez et al. 2019). While, in post-mining forests, the most dominant tree species were Cecropia peltata, Vismia baccifera, Cosmibuena macrocarpa, Ochroma pyramidalis, Welfia regia, Pityrogramma calomelanos, Cespedesia spathulata, Perebea xanthochyma, Inga chocoensis, Pourouma bicolor and Ocotea cernua (Ramírez and Rangel 2019).
Experimental design
A design stratified by age of succession was used, with three strata for sampling. Stratum 1, initial age (EI) included areas recently degraded by mining, with a succession time between 12 to 15 years. This stratum presented small shrubby and woody vegetation, with a smaller average diameter and richness of tree species. This ecosystem is dominated by plant species such as Cespedesia spathulata (Ruiz & Pav.) Planch, Cyathea sp., Ficus sp., Vismia sp., Cosmibuena macrocarpa, Hampea romeroi Cuatrec., Xylopia macrantha Planch. & Triana, Piptocoma discolor (Kunth) Pruski, Abarema barbouriana (Standl.) Barneby & J.W.Grimes, Isertia pittieri Standl, Lunania parviflora Spruce ex Benth, Bellucia pentamera Naudin, Cecropia peltata L., Croton sp., Faramea multiflora A. Rich., Tococa guianensis Aubl., and Inga acrocephala Steud. The second stratum, stratum 2, called recovery age (ER) corresponded to areas that had a recovery time between 30 to 35 years; in which, an arboreal vegetation with greater diameter and specific richness was found. This ecosystem is dominated by plant species such as Cespedesia spathulata (Ruiz & Pav.) Planch., Cosmibuena macrocarpa, Clidemia septuplinervia Cogn., Cecropia peltata L., Inga lopadadenia Harms., Crotonsp., Vismia sp., Socratea exorrhiza (Mart.) H.Wendl., Ficus sp., and Licania mambranaceae Sagot ex Laness. The time elapsed since the mining activity ended was determined with interviews with the miners in the area, with the reports of the mining permits issued with the Mayor's Office, and with the information provided by the community council "Consejo comunitario" of the locality. Finally, the last stratum 3, corresponded to primary forests present in the region, and was taken as a reference scenario.
Establishment of plots
In stratum 1 (EI), mines with a recovery time between 12 to 15 years were selected. Five plots of 25x25 m were established in these mines. Similarly, in stratum 2 (ER), which corresponded to another forest area previously affected by mining and with a regeneration time between 30 to 35 years, five permanent plots of 10x50 m were installed. In stratum 3, three plots of 1 ha (100x100 m) located in forests of the localities of Opogodó, in the Colombian Pacific, were used, which were divided into 25 quadrants of 20x20 m. The latter were used as reference units. The sampling units were installed randomly within the mines, avoiding the edge effect. The plots were installed with different sizes in the succession ages, due to differential characteristics in the structure of the vegetation of the post-mining areas; however, this situation did not influence the analyzes and results obtained, because the samples in which the fine roots were collected were the same in all the sites.
Estimation of the biomass of fine roots (FRB)
For the measurement of the FRB, ingrowth cores growth cylinders were used (Moreno-Hurtado 2004; Hendricks et al. 2006). In this sense, by means of a soil hole (8 cm in diameter and 20 cm deep), pits (samples) of a known volume of soil from each of the quadrants of the plots were extracted from the forest, and the fine roots present were removed, first in the field with the help of different caliber sieves and then in the laboratory. The soil extracted and free of roots was placed in the holes left by the hole, without any kind of packing, in such a way that the new soil is completely in contact with the surrounding environment and thus eliminate the obstacles that could limit the growth of the plants. To line the filling space and facilitate the subsequent collection of the sample, thick metal wires were used on the walls of the hole at three different points.
The fine root samples were taken in the center of the quadrants of the plots at a depth of 20 cm, which were separated according to their diameter and those with diameters ≤2 mm were determined as fine roots. Sand and clay were removed from the fine roots sampled every six months using sieves with different sieves (0.5 and 1 mm wide) and subjecting the samples to different water pressures. The collected fine roots were dried at 70 °C for 48 h in the Acequilab Ltda® drying oven and weighed dry with a precision analytical balance (0.0001 g). These procedures were carried out in the Laboratory of Botany and Ecology of Universidad Tecnológica del Chocó "D.L.C". The dry weight values in grams of the fine roots were expressed in terms of t ha-1.
Measurement of edaphic characteristics
In each of the sampling units, five composite soil samples were taken at a depth of 20 cm, in the corners and the center of each of the quadrants of 5x5 m, 10x10 m and 20x20m. In total, 300 composite soil samples were taken, 125 in the EI and ER successional strata of the areas degraded by mining and 175 in primary natural forests. The soil samples collected were sent to the Biogeochemistry Laboratory of Universidad Nacional de Colombia - Medellin Headquarters. In said laboratory, texture (percentage of sand, silt, and clay), pH, OM content, Al, effective cation exchange capacity (ECEC), and nutrient content (N, P, K, Ca and Mg) were analyzed, using the techniques referenced below: the texture with the Bouyoucos technique, the pH with the potentiometer of soils: water 1:2 ratio, the OM with the Walkley and Black technique and with volumetrics, the P available with L-ascorbic acid, UV-VIS spectrophotometer, total N with the Micro-Kjeldahl analytical technique, Al with 1 M KCl/volumetry, NTC 5 263, nutrients (Ca, Mg, K) with the 1 N ammonium acetate method, neutral, atomic absorption (Osorio 2014). The results of the physicochemical characteristics (nutrients, acidity, and texture) evaluated in the two post-mining forests and in the reference primary forest are described in greater detail in a recent study carried out in the study area (Quinto et al. 2022).
Statistical analysis
The Kruscal-Wallis (KW) non-parametric test was used to evaluate the variation of the FRB as a function of the recovery time, given that the assumptions of normality and homogeneity of variances of the data and their residuals were evaluated with the Bartlett, Hartley and Kurtosis statistics (between +2.0 and -2.0). Then, to determine the linear correlations and associations between edaphic variables (pH, MO, Al, total N, P, K, Ca, Mg, CICE, sand, silt, and clay) of each of the successional stages (strata 1 and 2) and the FRB, multiple regressions were used with the method of selection of significant variables backward; for which, the FRB and soil data were transformed with the natural logarithm, due to their lack of statistical normality. In addition, a principal component analysis (PCA) was used to evaluate the co-linearity and relationship between the edaphic variables, with the PCA data, the principal component with the highest significance (eigenvalue>1.0) was selected as an integrated soil fertility variable, similar to what was done by Powers and Peréz-Aviles (2013). The values of the principal component (Component 1) were related to those of the FRB using simple exponential regression models. Finally, General Linear Models (GLM) were used to evaluate the influence of successional age, the main component (Component 1) as an integrated measure of edaphic fertility, and the physicochemical variables of the soil on the FRB together. The analyzes were performed in the R programming environment (R Development Core Team 2012).
RESULTS AND DISCUSSION
The FRB presented statistically significant differences between the different successional stages in the areas degraded by open pit mining (KW=151.8; P<0.00001). However, these differences did not show a trend of increasing or decreasing FRB over time (Figure 3). Specifically, in successional stages between 12 and 15 years, with sandy textured soils, the FRB was on average (±standard error) 5.73±0.87 t ha-1; while, in intermediate stages of recovery between 30 to 35 years, with sandy textured soils, the FRB was lower, with average values of 2.56±0.51 t ha-1. Finally, in reference forests, with clay-textured soils, the FRB was 5.91±0.85 t ha-1 (Figure 3).
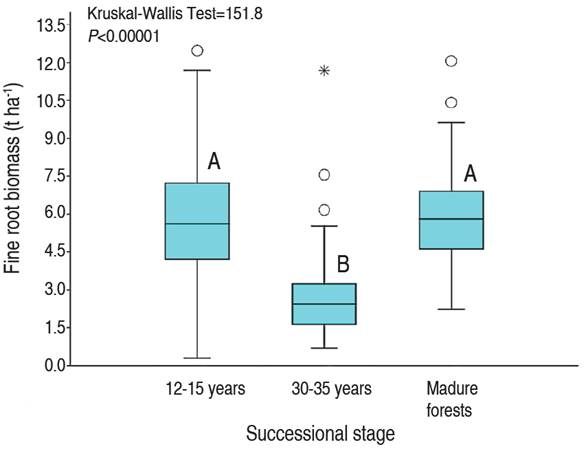
Figure 3 Fine root biomass as a function of successional stage in abandoned mines in Jigualito, Colombian Pacific. The letters "A" and "B" indicate significant differences.
The FRB recorded in areas degraded by mining in the Chocó biogeography presented values between 2.56 and 5.73 t ha-1; which is a value that is within the range between 3 and 120 t ha-1, reported for tropical forests (Vogt et al. 1986). Likewise, these values are similar to the belowground biomass recorded in areas degraded by open-pit mining in the biogeographical Chocó, with a recovery period between 1.5 to 6 years, with averages of 4.88 t ha-1, and a range between 0.45 and 12.81 t ha-1 (Quinto et al. 2013). These quantifications show that, in areas affected by open pit mining, the FRB recovers quickly; and in less than 12 years, FRB values were similar to those recorded in primary reference forests of 5.91 t ha-1 reported in this study, and also to those quantified in rain forests of Opogodó (5.91 t ha-1) and Pacurita (6.28 t ha-1), in the biogeographic Chocó (Quinto et al. 2016a). This similarity in the FRB of mature forests and areas degraded by mining denotes that with little time for plant succession, high amounts of belowground biomass can be reached, since 10 years of ecosystem recovery was sufficient time for the recovery of the FRB in soils.
The fact of not observing increases in FRB with the passage of succession time in areas degraded by mining is similar to that recorded by Brearley (2011) and Powers and Peréz-Aviles (2013), who also noted little relationship or increases in the FRB with successional age. Among the possible reasons that explain why there was no evidence of increases in FRB with succession time, the following can be mentioned: first, the recovery of FRB is very fast in disturbed and/or deforested areas (Brearley 2011; Powers and Peréz-Aviles 2013), even in areas affected by mining where the soil structure is affected (Quinto et al. 2013); surely due to the need to colonize new edaphic spaces left by the disturbance, and due to the need to quickly acquire the nutrients that are being released in this recently affected ecosystem. Second, due to the changes that occur in the distribution of aboveground and belowground biomass with the passage of time of succession (Feldpausch et al. 2004; Quinto et al. 2013); since, in the initial stages, the acquisition of nutrients from the soil is required for the development of the plants, which generates a greater FRB; subsequently, when a high proportion of nutrients (N, P, K, Ca, and Mg) has already been stored in the aboveground biomass as the time of succession progresses (Feldpausch et al. 2004), it becomes necessary to carry out more photosynthesis and growth in diameter and height, for which greater aerial biomass is produced, while the belowground biomass remains constant (Quinto et al. 2013), which is related to what was stated by Bloom et al. (1985), who propose that plants respond homeostatically to the imbalance of resources in the environment, for example, the deficit of nutrients and/or sunlight, through the allocation of the resources for the acquisition of the elements that are more limiting to growth (Bloom et al. 1985). Therefore, it is denoted that in the initial stages, more fine roots are produced due to the limitation by soil nutrients, and in the later successional stages, more aboveground biomass and litter production are generated due to the competition for solar radiation for photosynthesis, as evidenced in areas altered by mining (Quinto et al. 2013) and in this investigation.
The third reason is related to changes in the composition of plant species that occur with succession in areas affected by mining (Valois-Cuesta and Martínez-Ruiz 2017; Ramírez and Rangel 2019); since, in the initial stages, a greater number of herbaceous and small woody species that have a greater number of fine roots are recorded; while, in late successional stages, there is a greater presence of trees and shrubs, which tend to have a higher biomass of thick roots. Therefore, these changes in species composition would be partially explaining the variation in FRB in these areas affected by mining. This assertion is related to what was reported by Valverde-Barrantes et al. (2007), who observed variations in the FRB content according to the type of dominant tree species in the ecosystem, which is partially similar to what was reported in this study; since, with the changes in the floristic composition, there were also changes in the FRB. In summary, it is concluded that the little increase in FRB with succession is due to factors such as the rapid colonization of fine roots in degraded areas due to the need to acquire nutrients and occupy previously affected soil spaces, variations in the distribution aboveground and belowground biomass with succession, and changes in floristic composition over time. Likewise, the little increase of the FRB with the succession denotes the need to restore the forest ecosystem; therefore, there is evidence of a retrogression with the time of succession that needs to be corrected.
Spearman's correlation analysis showed a negative relationship between fine root biomass and O.M., total N, P, Ca, Mg, and CICE contents, in post-mining areas in general (S2-Table 2, S3-Figure 1, Figure 4B). Using PCA, it was denoted that the first two principal components (component 1 eigenvalue=4.41 (36.7% variance); component 2 eigenvalue=3.03 (25.28% variance); explained the 61.98% of the total variation of the physicochemical variables of the soil of the mines together (Figure 3A). Likewise, an association was observed between edaphic variables such as OM, total N, Ca, CICE, Mg, Al, and K (Figure 4A). For its part, the ACP including the FRB denoted that biomass was positively associated with edaphic variables such as clay, silt and pH; while, with OM, total N, Ca, CICE, Mg, Al, and K denoted an inverse relationship (Figure 4B). Through the GLM, it was determined that, in the areas degraded by mining, in general, the FRB was significantly determined by the successional age, the Principal Component 1, OM, and soil clay (Table 1). However, in degraded areas between 12 and 15 years of recovery, FRB was positively associated with OM and silt; and negatively with clay and sand (Table 2). While, in the degraded areas between 30 and 35 years old, the FRB was positively related to Al, N, Ca and the percentage of clay; but, with K, OM and Mg it was negative (Table 3).
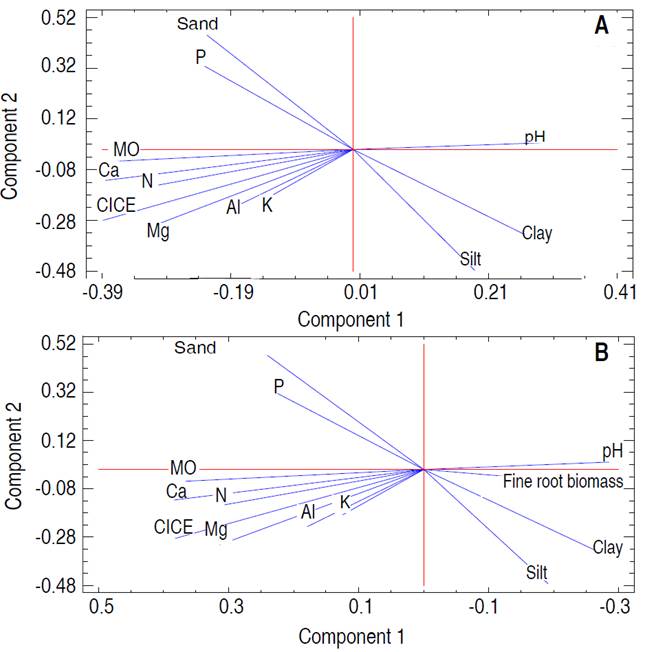
Figure 4 Principal component analysis (PCA) for fine root biomass variables and soil parameters in abandoned mines in Jigualito, Colombian Pacific. Where the component 1 eigenvalue=4.41 (36.7% variance); component 2 eigenvalue=3.03 (25.28% variance) of the total variation.
Table 1 General Linear Models of fine root biomass as a function of edaphic variables in abandoned mines in Jigualito, Colombian Pacific. Where R2=32.09%, R2 (adjusted)=24.7%.
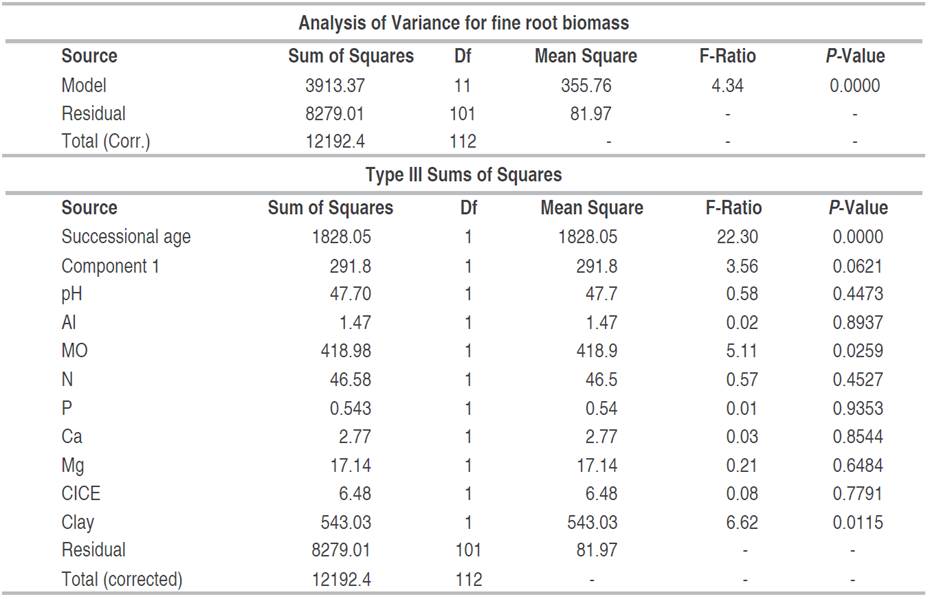
Table 2 Multiple regression of fine root biomass as a function of edaphic variables in abandoned mines in Jigualito, Colombian Pacific. Where R2=19.81%, R2 (adjusted)=9.78%. Backward variable selection method. (12-15 year).
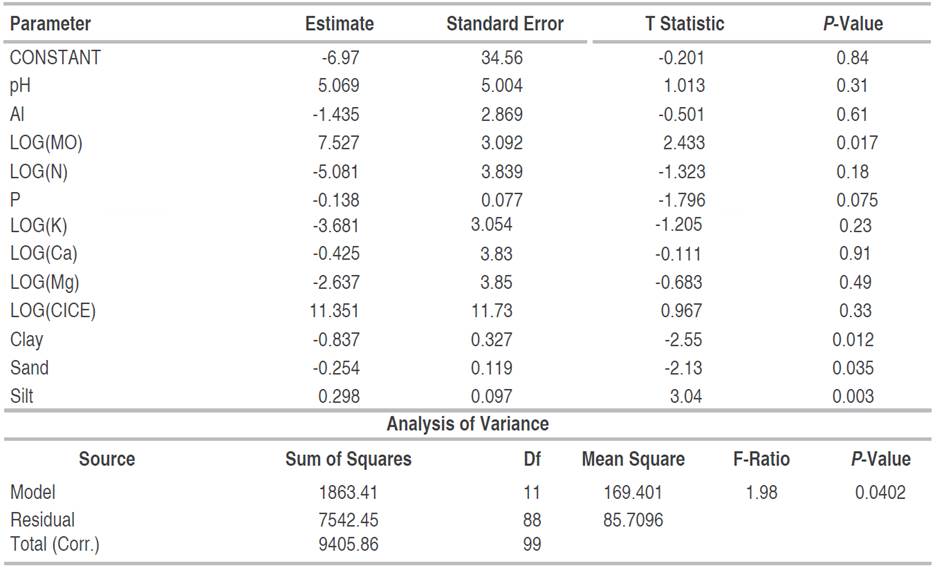
Table 3 Multiple regression of fine root biomass as a function of edaphic variables in abandoned mines in Jigualito, Colombian Pacific. Where R2=95.64%, R2 (adjusted)=89.53%. Backward variable selection method. (30-35 year).
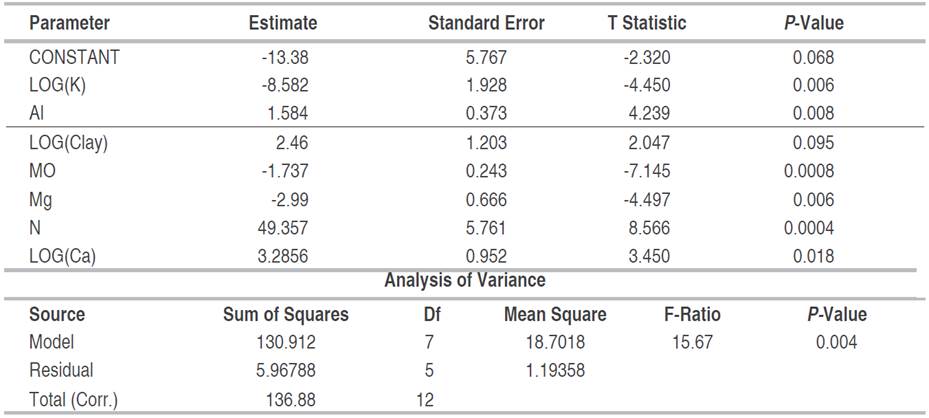
Relationship of the FRB with the physicochemical variables of the soil in areas affected by mining
FRB in areas degraded by mining was associated with edaphic variables such as texture (percentage of clay and silt), pH, and soil fertility (nutrient content, OM, and Principal Component 1). Specifically, a negative relationship between FRB and variables such as MO, total N, Ca, Mg, and K was denoted; which is similar to that previously reported in various studies carried out in tropical forests, in which it is evident that the mass (biomass and/or necromass) of fine roots is negatively related to the edaphic content of N (Maycock and Congdon 2000; Powers et al. 2004; Brearley 2011, Powers and Peréz-Aviles 2013; Singha et al. 2020), P available (Gower 1987; Powers et al. 2005; Powers and Peréz-Aviles 2013; Quinto et al. 2016a), Mg (Powers and Peréz-Aviles 2013), and Ca (Gower 1987; Powers and Peréz-Aviles 2013). These negative correlations between FRB and soil nutrients denote nutritional limitations for the growth of trees in the ecosystem (Brearley 2011), which is evidenced in the present study, since the edaphic content of Ca, K, Mg and CICE was very low. In addition, the contents of OM, total N, and P available, although very high, their absorption may be limited due to the high contents of Al that are recorded in the area (Ramírez et al. 2019). This is due to the fact that the high availability of Al produces a decrease in root elongation, reduces stem growth, alters metabolic and physiological processes, and decreases the productivity of the ecosystem (Shetty et al. 2021). Likewise, soil Al reduces nutrient absorption, produces nutritional stress, chlorosis and necrosis, decreases leaf size, and affects photosynthesis (Chandra and Keshavkant 2021), with which, the acquisition of nutrients is affected, and nutritional limitation is generated.
With open pit mining, the texture of the soil is generally altered, which presents considerable relevance, since the FRB was positively related to the percentage of silt and clay. This positive association is explained by the fact that in soils with high clay and silt contents some cations are retained (P, Ca, Mg, K) (Osorio 2014), against which, the FRB presents increases to be able to absorb them. This increase in FRB is commonly referred to as proliferation; and in the present study, it was observed in small patches of clay, silt, and OM, in soils that are predominantly sandy, as a strategy to acquire nutrients fixed there, similar to what was observed by Roy and Singh (1995), who recorded higher FRB in the soils when there was greater availability of N and C, as well as, they are related to the results of Metcalfe et al. (2008); Jiménez et al. (2009); Kochsiek et al. (2013) and Quinto et al. (2016b), who observed higher rates of productivity and turnover of fine roots in small patches of sandy and/or nutrient-rich soils, as a strategy to acquire nutrients in tropical oligotrophic soils. This greater biomass and dynamics of fine roots in sandy soils is possibly due to the fact that the high sand content favors aeration (oxygen content) and macroporosity (Osorio 2014), and with this the growth and biomass of fine roots the soils is promoted.
The relationship between the soils and the FRB was different in the successional stage; in early stages (12-15 years), where soils are sandy, acidic and poor in nutrients (Ca, Mg, K), with high contents of available P and OM, FRB was positively associated with OM and silt content, similar to what occurs in poor and infertile soils, where fine roots proliferate in small areas and soil patches with higher nutrient content (Kochsiek et al. 2013; Quinto et al. 2016b). This is similar to that reported by Roy and Singh (1995), who observed increases in FRB related to seasonal increases in edaphic N and C content in acid and sandy soils present in tropical dry forests in India. This evidence a strategy of rapid production of FRB in environments that are very poor in nutrients, as is surely happening in areas degraded by open-pit mining, but in the initial stages of plant succession.
While, in areas affected by mining with a longer time (30-35 years) of succession, the FRB was negatively related to the content of K, MO, and Mg; This is similar to the pattern observed in low altitude tropical forests, where the mass of fine roots is negatively related to edaphic fertility in response to the nutritional limitation of the ecosystem (Gower 1987; Maycock and Congdon 2000; Powers et al. 2004; Brearley 2011; Powers and Peréz-Aviles 2013; Quinto et al. 2016a; Singha et al. 2020). However, in these sites, a positive relationship between FRB and soil Al content was also denoted, which may possibly be related to the presence of tree species tolerant to high Al contents recorded in these successional stages (Ramírez et al. 2019). Since, with the toxicity generated by the availability of Al, some tree species may be benefiting more than others (Watanabe and Osaki 2002); and probably, they would be responsible for the positive relations between the FRB and the edaphic Al. In particular, trees from botanical families such as Melastomataceae, Rubiaceae, Euforbiaceae, and Lauraceae, recorded in these areas affected by mining (Valois-Cuesta and Martínez-Ruiz 2017; Ramírez and Rangel 2019) have been previously described as Al tolerant and bioaccumulative (Jansen et al. 2002). Therefore, they would surely be responsible for the relationships denoted between the FRB and the edaphic Al. This phenomenon is similar to that recorded with the species Acacia mangium, considered to be tolerant to Al toxicity (Watanabe and Osaki 2002), and which has been successfully used in restoration processes of areas degraded by mining in some regions of Colombia (León and Osorio 2014), including the Colombian Pacific.
CONCLUSIONS
In the areas degraded by mining in Colombian Pacific, the relationship between the FRB and the physicochemical conditions of the soil changes as succession progresses. In addition, the FRB trends show nutrient limitations of the ecosystem. These limitations are determined by the lack or scarcity of nutrients generated by mining, sandy, and rocky textures with little mineral retention, the influence of high edaphic Al contents, and changes in the composition of tree species, especially, the N fixers and those tolerant to Al toxicity, which would be the main responsible for the recovery of nutrients and the FRB of the ecosystem. In synthesis, after the degradation of the ecosystem, as occurs with mining, the adaptations that the forest develops are denoted, such as the development of abundant biomass of fine roots, to acquire mineral nutrients from the soil, which evidences the strong affectation that is caused by mining. In addition, it is shown that it is necessary the restoration and that with a recovery of soil fertility in areas affected by anthropic activities, the underground carbon storage of the ecosystem is improved in the long term, and with it, the mitigation of global climate change.