1. Introduction
Sustainable development (SD) aims to enhance society's quality of life by considering social, economic, and environmental pillars [1]. Internationally, numerous countries have endorsed the Paris Agreement (PA) and the 2030 Agenda, which encompasses 17 sustainable development goals (SDGs) to address climate change and promote SD. Among the significant contributors to climate change, two sectors are intrinsically connected to these pillars of SD: the energy and waste sectors [2,3]. These two sectors are among the main emitters of greenhouse gases (GHG), accounting for 73.2% and 3.2% of emissions, respectively [1]. Consequently, transforming them necessitates robust national-level public policies to guide their responsibilities in facilitate SD transition [4,5].
Adequate waste disposal continues to be one of the key challenges faced by developing countries in their journey towards achieving SD [6]. Despite efforts to address this issue and ensure adequate disposal in sanitary landfills, there are adverse effects to consider, such as methane generation through the anaerobic degradation of organic compounds, leading to GHG emission that impact the environmental and energy aspects [7]. Nevertheless, gases produced in landfills can be harnessed and converted into biogas, providing a renewable energy source [8]. This approach benefits both the waste and energy sectors, as it addresses their significant challenge of replacing fossil fuels in the global energy mix [3,9].
Biogas utilization from landfills (BUL) is a technique initially proposed in the 1970s and has since become a common practice in countries like the United States for around 30 years, and more recently in many European territories [7,10]. However, in Latin American and Caribbean countries, as well as other low- and middle-income economies, there are still misconceptions surrounding its implementation. These range from technical and technological ignorance to issues related to education, governance, and the lack of public policies that promote its development [10-12].
Despite the challenges faced by developing countries, numerous studies highlight the economic, energy, social, and environmental potential of BUL, emphasizing its essential role in achieving SD in these regions [7,10,13]. Some include: an environmental and economic analysis to identify the potential and innovations for using municipal solid waste (MSW) as an energy source in African urban areas [14] and in Bangladesh [15]; a large-scale study of biogas generation with multicriteria analysis at technical, economic, institutional, and organizational levels in Bolivia [16]; an evaluation of energy potential based on the population of each region and subsequent economic study in Brazil [8]; projections of methane emissions in 6 sanitary landfills operating between 2015 and 2020 in Malaysia [17]; an evaluation of energy potential from biogas for electricity production in Pakistan [18]; and an economic and environmental assessment of facilities using biogas for energy purposes in Thailand [19].
As evident from the mentioned studies, there is a focus on technological assessments, but there is a lack of comprehensive discussion on the energy potential of using biogas from landfills and the existing policies and mechanisms in each country that can promote its efficient development, considering the involvement of the two sectors.
Colombia, an emerging economy in South America, has a population of 45.5 million inhabitants and a 2022 GDP per capita of 6.63 thousand U.S. dollars [20,21]. In the 1990s, the country restructured public services, yielding notable advancements in energy and waste sectors compared to other countries in the region [22,23]. The National Interconnected System (SIN) provides electricity access to around 99% of users in the energy sector [24], while almost 97% of the generated waste is sent to landfills following national standards in the waste sector [25]. However, both sectors must undergo in-depth restructuring to address global challenges such as inadequate waste disposal, climate change, and limited energy access in rural and isolated areas. Integrating renewable energy sources and ensuring energy access can help the energy sector reduce emissions. Meanwhile, the waste sector must address the upcoming crisis related to the lifespan of most landfills in the country [26]. Currently, each sector has separate public policy strategies for transitioning towards SD and climate change mitigation [26,27].
Some studies on BUL have been conducted in Colombia. For instance, Borzychowski et al., (2012) performed a technical and financial pre-feasibility study for a waste-to-energy project in an existing landfill, aiming to generate electricity from collected biogas. Acosta and Pasqualino (2014) conducted a review of biogas production in Colombia, considering MSW at that time, and evaluated the potential for using this technology. Another study by Alzate Arias et al., (2018) estimated the electricity production potential of three Colombian municipalities (Pasto, Andes, and Guayatá), selected based on socioeconomic and demographic characteristics. However, these calculations did not consider other municipalities with higher population density, GDP, and different gravimetric characterizations.
Understanding the potential of biogas for electricity generation is crucial to develop suitable mechanisms and strategies for public policies that promote its widespread use in two key sectors: energy and waste [12]. Consequently, this article aims to firstly estimate the electric energy generation from biogas produced in landfills in Colombia's main cities using the method proposed by Yedla (2005) based on MSW characterization and quantity. Secondly, it discusses the contribution of this technology to current public policies on SD and climate change in the energy and waste sectors, using Colombia as a case study.
2. Methods
This study involves a mixed analysis [32] to integrate quantitative results electric power generation potential in Colombia's main cities with qualitative discussions on the contribution of BUL to meet current Colombian public policies. These public policies focus on SD and climate change mitigation in the energy and waste sectors. The procedure is summarized in Fig. 1.
Firstly, to quantify power generation potential, we focused on major cities based on their MSW generation (Cali, Medellín, Barranquilla, Cartagena, Bucaramanga, Santa Marta) using the 2018 annual report from the Superintendence of Domestic Public Services (SSPD) [33]. Bogotá, as the central city, was excluded from the analysis due to an existing biogas treatment station at the Doña Juana landfill since 2009, with a capacity of approximately 6,700 tons per day and a total installed generation capacity of 24.68 MW [34].
Zamorano et al., (2007) and Fei, Wen and De Clercq (2019) mentioned various techniques to estimate biogas and energy generation, each with varying assumptions and complexity. This study opted for an estimation technique that best fit the available data, considering regional waste characterization as a key variable, a factor not explored in previous Colombian studies. The calculation method proposed by Yedla (2005) was used to estimate the energy potential of biogas recovery in a landfill, based on total organic carbon, collection efficiency, and methane content of the collected gas [36].
Eq. 1:
Total assimilable carbon available in one year
Where,
Wa= annual waste generated (tons)
flf =fraction land filled
fa-doc=fraction of assimilable organic carbon in the degradable waste
Eq. 2:
Total gas generated from one year of waste
Where,
rlfg=methane generation rate (m3/t)
fmethane=fraction of methane in landfill gases
Total energy generated from one year of waste using landfill gas system with biogas recovery.
Eq. 3:
Where,
ψ= gas collection efficiency
( = calorific value of Methane (Kcal/m3)
The formula yields result in kcal/year, which were then converted to kWh/year further converted to the equivalent power generated in MW. To obtain the parameter r lfg , the calculation proposed by [36] was utilized to gather additional information. Initially, the gravimetric characterization of MSW in each city was investigated and adapted based on specific categories: organic waste, paper and cardboard, plastics, textiles, rubber and leather, wood and other biodegradables, glass, metals, and others. Each waste type was subsequently classified into one of the three classes shown in the first column of Table 1. The heat calculated from r lfg directly reflects the methane generation rate; obviating the need to employ f methane in the calculation.
Table 1 Waste characterization and methane generation potential
Waste characterization | Methane generation potential (m3 CH4/ton) |
---|---|
Relatively inert (RI) - plastics, glass, metals, others | 20 |
Moderately Decomposable (MD) - textiles, rubber, and leather | 120 |
Decomposable (D) - organic, paper and cardboard, wood, and other biodegradable | 160 |
Source: [37]
After estimating the energy potential in the country's main cities, a content analysis of key public policies related to SD and climate change mitigation in the energy and waste sectors was conducted. The content analysis helped process a large amount of text from these policies to address the research questions set forth in this study [38]. The formulated research questions were as follows:
RQ1: How would the efficient implementation of this technology contribute to the country's public policies on SD in these two sectors?
RQ2: What potentialities and weaknesses exist in the country's public policies regarding implementing this technology for SD and climate change mitigation?
Data collection involved two stages. The first stage encompassed a quantitative study, including literature reviews on "biogas use in Colombia" and “waste characterization”, as well as a documentary review, including including government reports, to gather information for the estimations in this study. The second stage involved a qualitative analysis. The identification of public policies on biogas, waste management and renewable energy in Colombia was carried out through scientific and gray literature reviews using databases from recognized universities in the country, as well as consultations on the websites of leading government institutions.
Quantitative analysis calculated inhabitants and percentages that could benefit from the technology's efficient implementation, based on regional per capita energy consumption. BUL's energy potential was compared to SIN's 2018 capacity. The qualitative analysis drew from the research of Rodić and Wilson (2017) and McCollum et al., (2018), which identified governance aspects related to the contribution of the waste and energy sectors in meeting the SDGs. Consequently, this article presents the specific contribution of BUL to achieving the SDGs and its connection to the bi-sectoral public policies in Colombia for SD advancement. The quantitative results allowed for a sector-specific discussion on the role of this technology in contributing to SD and assesing the adequacy of existing Colombian public policies in this content.
Results and discussion
3.1. Solid waste composition
Table 2 displays the MSW gravimetric composition in the selected cities for energy generation calculation. Note that the chosen landfills serve both the primary cities and nearby municipalities. According to Coskuner et al. (2020) and Marmolejo et al. (2010), any waste management strategy requires an accurate and standardized characterization of MSW [39,40]. However, it is evident that there is no standardized method for MSW characterization across the cities included in the study.
Table 2 Characterization of MSW by the main cities in the country

Source: Own creation
Notes: a. Organic; b. Paper and cardboard; c. plastics; d. textiles, rubber, and leather; e. Wood and others; f. glass; g. metals; h. others.
1Adapted from [40], 2Adapted from [41], 3Average of the gravimetric characterizations carried out on the MSW in the landfill. Source: [42], 4Adapted from [43], 5Adapted from [44], 6Adapted from [45]. 7 Adapted from [70]
Fig. 2 compares the gravimetric characterizations of each city presented in this article with the national average. Based on the data collected for this country, the waste composition difference is not directly influenced by the amount of waste generated (parameter Wa, Table 3), nor by the total population at the regional level (See Table 4).
3.2. Parameters and estimation of electric power generation
Table 3 presents the values of each parameter to calculate the energy recovery potential in each of the analyzed cities.
Table 3 Parameters adopted for calculations.

Source: Own creation
Notes: 1 This information corresponds to the year 2018 [33]. 2 The value one is used, as the information used in the equation is the total fraction destined for landfills in each city. 3 Values used by Yedla (2005) based on studies reported in the literature. 4 Value found in Pasto(2007)
Table 4 displays the methane gas generation, electrical energy (kWh/year), and power output in MW based on the parameters. It also compares energy supply to inhabitants and the percentage of the total population of each region that this represents. For instance, taking Cali as an example, the amount of waste generated and discarded annually, which is 756,304 tons, has the potential to generate enough energy to supply nearly 20% of its population. Similarly, in other regions, the energy generated could help supply approximately 13% of the inhabitants of Medellín (energy potential of 41.74 MW) and around 23.38% of the inhabitants of Barranquilla (energy potential of 37.32 MW). It is important to consider the annual per capita consumption, which according to the Planning Unit Energy Mining (UPME), is 1159 kWh/year [46], as it can provide valuable insights into the overall energy consumption patterns and requirements in each region.
3.3. Correlation between BUL and public policies in the waste and energy sector towards SD
BUL implementation in developing countries has been insufficient, resulting in the underestimation of its contributions toward SD in each region. Table 5 presents a bi-sectoral analysis of how BUL can promote the transition towards SD by considering the correlation between this technology, Colombian public policies, and their impact on achieving the SDGs, based on the research of Rodi and Wilson (2017) and McCollum et al. (2018).
Colombia, as one of the signatory countries of the 2030 Agenda and the PA, has ratified these agreements by incorporating them into national public policies for compliance.
Regarding the 2030 Agenda, the waste sector has not fully recognized its potential contribution to SD. Through CONPES 3918/2018, the national government defined the roadmap for implementing the 17 SDGs [47]. Within this policy, waste management is included in the promotion of SDG 8 and SDG12 through recycling and waste reuse, SDG 11 through appropriate waste disposal practices, and SDG 13 through waste management actions under the PA framework. Importantly, all the measures to comply both agreements are contained in CONPES 3874/2016, also known as the National Solid Waste Management Policy (PNGRS) [47].
BUL plays a significant role in achieving multiple SDGs, including SDG 3, SDG 11, SDG 12, and SDG 13, as it effectively reduces the environmental impacts caused by methane emissions from landfills. The results indicate that its implementation in the main cities of the country could reduce a total of 216.1 x106 m3 per year of methane production. This reduction is crucial in mitigating climate change, considering that methane is 21 times more potent than carbon dioxide, as highlighted by [48].
Despite advancements in modern technology for methane extraction and utilization, it is concerning that approximately 25% of methane produced in sanitary landfills still escapes into the atmosphere.
Looking ahead to 2030, the total projected emissions in Colombia are estimated at 332.73 Mton CO2eq, with a mitigation scenario targeting a reduction of 66.48 Mton CO2eq. In alignment with Law 1931/2018, each economic sector related to climate change must determine its Comprehensive Management Plans for Climate Change (PIGCCs) [49]. Specifically, the waste sector, responsible for 6% of the country's total emissions, has established that BUL aims to reduce at least 2.9 Mt of CO2 eq in GHG emissions yearly (27,49,50). On the other hand, the energy sector aims to reduce 11.2 Mton CO2eq, representing 16.8% of the NDC target. The energy sector's main strategy is transforming the electrical matrix to integrate more renewable energy sources [27].
To achieve this, the government enacted Law 1715/2014, which allows the integration of FNCERs into the National Energy System (SEN). This law permitted the use of energy from solid waste that couldn't be reused or recycled and had to be delivered to the landfill [51]. In 2018, the PIGCC of the mining-energy sector (PIGCC ME) (Resolution 40807/2018), laying the groundwork for long-term contracting auctions [52], aiming for 2,500 MW of installed solar and wind power capacity by 2022 [53].
Moreover, the government's support and promotion of BUL through financial and institutional incentives align with SGD 12. In Colombia, the Ministry of Housing, City, and Territory Development (MVCT) and the Commission for the Regulation of Potable Water and Basic Sanitation (CRA) have established public policies to encourage the development of waste sector projects. However, Resolution CRA 853/2018 established the final disposal fee without fully considering the potential of biogas treatment, hindering its use and its contributions to SD transition and climate change mitigation [54]. The PNGRS proposes inter-institutional cooperation with other sectors, such as energy and agriculture, to implement waste-to-energy technologies. Nonetheless, the lack of clear assignment of responsibilities in this area persists, causing delays in the development of projects [26].
Table 5 Linking BUL to Waste and Energy Sector Policies for Sustainable Development
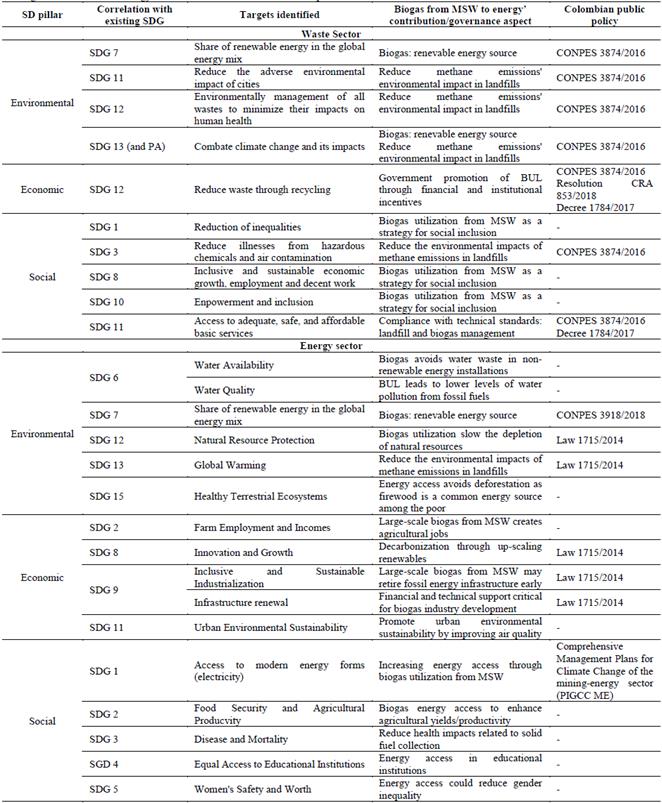
Source: Own creation. Notes: SDG 1: No poverty; SDG 2: Zero Hunger; SDG 3: Good health and well-being; SGD 4: Quality education; SDG 5: Gender equality; SDG 6: Clean water and sanitation; SDG 7. Affordable and clean energy; SDG 8: Decent work and economic growth; SDG 9: Industry, innovation and infrastructure; SDG 10: Reduced inequalities; SDG 11: Sustainable cities; SDG 12: Responsible consumption and production; SDG 13: Climate action; SDG 15: Life on land.
The waste sector's commitments to SDGs mainly focus on economic and environmental aspects, neglecting other essential factors. Notably, Colombia is home to a substantial number of informal waste pickers in the region. As reported by Aluna Consultores Limitada (2011) the number of waste pickers exceeded 50,000 by 2010 [55] and continues to grow due to the influx of migrants from neighboring countries, such as Venezuela [56], as well as the impact of the COVID-19 pandemic [57]. Promoting BUL as a strategy for social inclusion would enable the generation of employment opportunities and the implementation of positive actions for informal waste pickers, who currently lack sufficient government and public health support. This approach would facilitate the fulfilment of SDG 8 and SDG 10 within the waste sector, thereby promoting the social aspect of SD in Colombia.
Regarding the energy sector, CONPES 3918/2018 highlights the importance of increasing electricity coverage in isolated and rural areas and fostering international cooperation for clean energy research and technology to comply with SDG 7 [47]. McCollum et al. (2018), support the idea that energy sector compliance with SDG 7 is influence all other SDGs. Table 5 shows the energy sector's participation in BUL, contributing across SD pillars. Compared to other non-conventional renewable energy sources (FNCERs, initials in Spanish), the country needs sufficient incentives to integrate BUL into the energy mix. Intersectoral projects require strategic alliances and institutional arrangements to harness MSW-derived energy potential effectively.
Colombia presented its 2015 NDC within the PA framework, outlining mitigation and adaptation goals to address climate change. Initially, the goals sought to reduce emissions by 20% below the projected 2030 levels in a Business as Usual (BAU) scenario or by 30% with sufficient international support [58]. For COP26, Colombia raised its ambitions, targeting a 51% reduction below the projected 2030 levels in a BAU scenario, with a long-term goal of achieving emissions neutrality by 2050 [59]. To meet the NDC's goals, Colombia enacted Law 1931/2018, establishing the National Climate Change System (SISCLIMA). This system encompasses policies, regulations, processes and involvement of governmental and private entities, along with resources, plans, strategies, instruments, mechanisms, and information to manage mitigation and adaptation to GHG in response to climatic changes [60]. The Intersectoral Commission on Climate Change (CICC) coordinates and guides the implementation of climate change strategies [61]. The NDC's mitigation target is equitably distributed across key sectors of the economy [62].
Despite notable progress in integrating renewable energies into the Colombia's electricity matrix, the expected participation of biomass, including MSW, is projected to increase modesty from 22 MW to only 56 MW in the expansion plan from 2020 to 2034. Among the additional 6,008 MW proposed for this period, wind and solar make up 84%, while minors, biomass and cogeneration account for 10%, and major hydro constitutes the remaining 6%. No thermal expansion is planned. These projections depend on the formulation of an attractive fiscal framework with efficient incentives to promote the entry of FNCERs [63].
According to UPME (2020), the electricity generation installed capacity in the SIN comprised 68.1% hydraulic energy, 30.8% thermal (including 21.1% Gas, 9.2% Coal, and 0.5% liquid fuels), and a mere 1.1% from non-conventional renewable sources (comprising 0.1% biomass, 0.7% cogeneration, 0.1% wind, and 0.2% solar) [63]. The collective energy potential of the seven analyzed cities is estimated to be 206.42 MW. When compared to the total capacity of the SNI in 2018 (17,660 MW), this represents approximately 1.3% of the total. Despite the modest percentage, it signifies a noteworthy advancement in transitioning towards a more renewable-based electrical mix, especially considering the positive impact that BUL offers at the energy level and across all three pillars of SD [63]. The prospect of fully harnessing biogas, along with other organic waste sources, demonstrates the capability to meet up to 20% of the demand for natural gas and positioning itself as a globally significant third source of energy [64-66].
The cities selected in this study align with the findings of Alzate, Restrepo-Cuestas and Jaramillo-Duque (2019), who suggest that implementing waste-to-energy plants using BUL is feasible in economically developed urban centers [67]. Among the advantages highlighted by these authors are the viability for municipalities with more than 100,000 inhabitants, reduced land use, and low investment.
The study by Triana and Velasquez (2019) emphasizes the importance of considering the specific characteristics of each region, such as calorific value for waste generation potential. In this article, data for waste characterizations were obtained from government portals, detailed information on chemical characteristics was limited and assumed based on the methodology used. It is essential to stress that up-to-date waste characterization is necessary for the successful implementation of BUL and other waste management technologies in municipalities.
Furthermore, Alzate-Arias et al., (2018) found that BUL involves relatively low investment and operating costs compared to other waste-to-energy technologies, even without the application of incentives set by Law 1715/2014. Despite producing less electricity, these projects remain viable. This aspect allows for the diversification of the country's electricity matrix and addresses the challenges associated with the end of the lifespan of many landfills across the nation.
4. Conclusions
In this article, we focus on estimating the electricity generation potential in Colombia's main cities and assessing the role of BUL in achieving public policy objectives related to SD and climate change mitigation. We also examine the potential alignment of BUL benefits with the SDGs and Colombian public policies focused on SD, with a specific emphasis on waste management and energy sectors.
The implementation of BUL requires a preliminary assessment of the electricity generation potential, which can be accomplished using the methodology described in this article or established approaches from the existing literature, while considering available data.
The integration of BUL into public policies, supported by incentives and efficient institutional arrangements, holds the potential to address at least 14 out of the 17 SDGs. However, our research highlights the underestimation of BUL's potential in shaping effective public policies that align with SD and climate change mitigation goals in the country.
The waste sector has not fully recognized BUL potential, and regulatory barriers hinder its implementation. To fully harness the benefits of BUL, there is a pressing need for this sector to focus not only on economic and environmental aspects but also on social factors. By promoting BUL as a strategy for social inclusion, the waste sector can generate employment opportunities and improve the lives of informal waste pickers, thereby advancing social development-related SDGs.
The transition of the energy sector towards non-conventional renewable energy sources, including BUL, is critical for achieving SD and further advancing the country's energy transition. Strategic alliances and institutional arrangements are necessary to effectively harness the energy potential of BUL. By doing so, significant strides can be made towards SD and mitigating the impacts of climate change.