1. Introduction
The problems of Supply Chain Management (SCM) have diversified by including contemporary environmental and social challenges, accommodating sustainability approaches in the supply chain (Bendul, Rosca and Pivovarova, 2017). Climate change, the depletion of natural resources, the pursuit of energy efficiency, ensuring the rule of law and combating discrimination, insecurity and corruption begin to be part of the problems to be solved in the field of the SCM (Rajeev, Pati, Padhi and Govindan, 2017). Other peripheral topics, which affect the business world and the SCM are extraordinary migration flows, changes in political paradigms, the fracture of commercial blocks and even the viralization of news on social media that also begin to pique the interest of companies and their Supply Chain (SC) (KPMG, 2018).
Correcting CS operations to optimize the use of resources and mitigate their negative impacts is the dominant approach in SCM to respond to contextual issues (Baumgartner, 2011); however, the efficient use of resources is not enough to contribute to sustainable development from the SCM (Schaltegger and Burritt, 2014). According to Gómez-Maturano (2018), the objectives of sustainable development have permeated in SCM mainly with a weak sustainability approach, limiting the inclusion of sustainability to levels of tactical-operational performance, rather than allowing a strategic vision towards sustainability. The SCM is being amended to help solve contemporary environmental and social problems (Seuring, 2013), which is favorable even if the prevailing approach is weak sustainability.
One of the contemporary problems in which SCM can contribute to sustainable development is the generation of huge amounts of Urban Solid Waste [MSW] in large cities. In 2015, around 680 million tons of waste was produced in cities per year and it was estimated that by the next 10 years the number would reach 2.2 billion tons per year (BM, 2016). Particularly in Mexico, the generation of MSW has more than doubled, from 60 thousand tons per day in 1990, to 145 thousand in 2015 (SEMARNAT, 2013, 2016).
MSW generation is associated with the traditional structure of the SC: goods are produced from virgin raw materials, sold, used and finally disposed of as waste (Ellen MacArthur Foundation, 2016). Currently, the rate of consumption of natural resources is 1.5 times faster than what nature takes to renew them (UNEP, 2015). In Europe, waste-based energy recycling and energy recovery are estimated to capture only 5% of the original value of raw materials, while 31% of food is discarded throughout the SC (Ellen MacArthur Foundation, 2016).
Therefore, it is encouraging to notice the growth in closed-cycle supply chain research (Govindan, Soleimani and Kannan, 2015) that seek to keep the amount of matter and energy entering an SC constant. In addition, research in Reverse Supply Chains (RSC) for waste recovery has been increased, contributing to the strengthening of the circular economy (Agrawal, Singh and Murtaza, 2015).
In order to restructure the production models and their SC traditional, it should be borne in mind that the correction of a system, as is the SC, has a limit, which is its optimal operation with which the system was designed (Blanchard and Blyler, 2016). An SC designed under the traditional objectives of the SCM has a reduced capacity for improvement in sustainable terms, since the design is focused on economic performance.
The objective of this work is to propose a conceptual design procedure based on Systems Engineering for the design of valuable MSW RSC with a weak sustainability approach. The particularity of this proposal is to propose the modeling of conceptual systems as a RSC design strategy considering an internal economic technical vision, for the performance of the system, and the interaction of RSC with its environmental and social environments, as a way of transiting sustainability. This document is part of the current conversation on sustainable supply chain design based on conceptual system modeling, and continues the discussion on SC design, modeling and validation presented in Bendul et al. (2017), Schaltegger and Burritt (2014), Abdala and Barbieri (2014), Seuring (2013) and Hassini, Surti and Searcy (2012).
2.Theoretical framework
Under a linear economy model, the SC is responsible for the production of consumer-oriented goods and services, taking of the nature matters the energy necessary to meet the needs and expectations of the customer. Once the customer concludes with consumption, at the end of the useful life of the product, it becomes a waste intended for abandonment by its producer or holder, constituting a waste of resources with high impacts in the economic, environmental and social environments. Once the product is disposed of, it enters an MSW handling system to drive it to final disposal (open dumps or landfills). The production pattern of the linear economy has changed very little since the last 200 years (GRI, 2015) and is the form adopted by traditional SC (Chopra and Meindl, 2013).
Traditional or direct SC includes all parties directly or indirectly involved in the activities of the processes of supply, production and distribution of goods and services (Chopra and Meindl, 2013). Through this SC there is a physical flow of goods, raw materials, inventory in process and finished products, in addition to the information related to them (Bowersox, Closs, Cooper, and Martínez Suárez, 2007).
Some authors conceptualize SC as a system (Chopra and Meindl, 2013; Bowersox et al., 2007; Jiménez-Sánchez and Hernández-García, 2002), which is consistent with the vision of this work. Under a system engineering vision, a system comprises a complex combination of resources (in the form of humans, materials, equipment, hardware, software, installations, data, information, services, etc.), integrated in such a way that they fulfill a specific function, or a number of functions, in order to respond to the identified needs (Blanchard and Blyler, 2016). The resources involved in the flow of materials and information in the SC are sufficient; but traditionally focused on three functions: supply, production and distribution (Chopra and Meindl, 2013). Agents are thus grouped from their supplying function into vendors at different levels, depending on their relationship with the focal company. The agents responsible for the manufacture of a product, component or assembly develop the production function. Distributors are agents involved in the placement of the finished product in final consumer markets. Direct flows of goods are based on this functional structure of traditional SC.
Disruptively to the flow of the linear model, RSC includes the processes needed to retrieve a used product from a customer and reuse it (Guide, Jayaraman and Linton, 2003). The RSC consists of a serie of activities necessary to recover products at the end of its life, from customers, either for the recovery activities of their residual value for the market, or to eliminate them properly (Nakashima and Gupta, 2013). This work conceptualizes RSC as a series of processes and operations necessary to recover, post-consumer, used products, their components or associated materials to valorization them, which involves reusing, recycling, restoring, re-manufacturing or cannibalizing them (Guide et al., 2003; Kinobe, Gebresenbet, and Vinners, 2012; Nakashima and Gupta, 2013).
Agrawal et al. (2015) identify the following RSC operations: waste procurement or acquisition, collection (accumulation), inspection and classification, storage, valorization (repair, reuse, remanufacturing or recycling) or final disposal. However, as established by Ilgin and Gupta (2013), RSC operations depend on the type of waste being handled.
This work establishes four functions carried out by the RSC oriented valorization of MSW: generation of waste from final consumption, includes activities carried out in MSW generation sources, in which materials are identified as without any additional value once consumption of the original product is made; acquisition, focuses on the collection of waste produced and stored on the different generation sources , starting from the garbage management infrastructure; the MSW valorization, through industrialized technology and processes, the materials acquired are valorization to be reinstated to supply chain; reintegration, directs the flow of recovered products to the different phases of a traditional SC, resembling the process of distribution of direct SC. These functions are shown at the bottom of Figure 1, which presents the reverse flow of materials.
Figure 1 is a closed-loop SC model, featuring a continuous circular flow of materials, constantly generating and retrieving value in a closed cycle, through the direct and reverse channels. A circular economy model can only be realized through synchronization between traditional SC and RSC (Govindan et al., 2015). Global savings for companies adopting circular economy models are estimated to be hundreds of billions of dollars per year (UNEP, 2015).
From Figure 1 it is established that the disruption between the linear economy model and its transmutation into sustainable circular economy models involves the recovery of post-consumer waste through RSC. The works of Lacy and Rutqvist (2015) and Veleva and Bodkin (2018) also focus on the materialization of this disruption between linear and circular. To make this transformation possible, in addition to having a robust recovery industry, an MSW procurement system must be in place to sustainably supply the industry. The regulatory framework for valorization MSW RSC should be sustainable development.
The World Commission on Environment and Development defines to sustainability the use of resources to meet the needs of the present without compromising the ability of future generations to meet their own needs (WCED, 1987 cited by Winter and Knemeyer, 2013). In the field of MSC, the environmental dimension includes a group of objectives, plans and mechanisms that promote environmental responsibility and encourage the development and dissemination of environmentally friendly technologies (Winter and Knemeyer, 2013). In addition, the impact of the company or organization on the social systems within which it operates is important (GRI, 2015). Social sustainability refers to working conditions, diversity, connection inside and outside the community, quality of life, etc. (Gimenez and Tachizawa, 2012). Long-term success and competitiveness is the basis of the economic dimension (Winter and Knemeyer, 2013); in contrast to the environmental and social dimensions, the economic is quantitative and focuses on the efficient use of resources and the return on investment.
For Carter and Rogers (2008) cited by Varsei, Soosay, Fahimnia and Sarkis (2014), sustainable SC is strategic and transparent integration, as well as the achievement of an organization’s social, environmental and economic objectives in the systemic coordination of major business processes, of form interorganizational, to improve the long term economic performance of the individual company and its SC.
Taking as a design framework these sustainability requirements for an RSC, this work makes a conceptual design from the adaptation of the Blanchard and Blyler (2016) systems engineering process. The conceptual design procedure consists essentially of the construction of alternative conceptual system that are a solution to a design problem posed from the specifications, requirements and needs posed schematically (Horváth, 2004). Conceptual design synthesizes the solution through concepts, expressed in the form of schemes with interconnected elements describing the operation of the system to be designed (Horváth, 2004). The engineering approach continually relies on intellectual constructions to solve the problems it faces, implying an imaginative mix of prior knowledge, knowledge of the functioning of reality and information obtained from real observations (Miser, 1993).
3.Methodology
The Systems Engineering procedure (SEP) for the conceptual design of RSC for MSW valorization, in a sustainability framework, is shown in Figure 2. The SEP includes an initial definition of the problem and the identification of a need of the final consumer, an analysis of feasibility, the development of system operational requirements, the conduct of a functional analysis. Subsequently, there is the iterative process of evaluation and validation, and the incorporation of changes for product improvement (which can be itself a process) as needed. Although the procedure is more directed at the early stages of system design and development, consideration of activities in the later stages of production (or construction), operational utilization, maintenance and support of the system, as well as disposal practices; are essential to understand the consequences of the decisions that are being made in this regard, as well as the establishment of guidelines and benchmarks for the future. In other words, the feedback cycle (as identified in Figure 2) is critical and an integral part of the SEP.

Figure 2 Procedure for conceptual design based on Systems Engineering. Source: Basedon Blanchard & Blyler (2016).
Definition of a problem and needs analysis
At this stage a “dissatisfaction” or “desire” for something is identified and is based on a real (or perceived) deficiency. Here is a complete description of the problem in specific qualitative and quantitative terms, in sufficient detail to justify progress towards the next step. More specifically, the following questions should be asked what is the nature and magnitude of the problem, and what are the associated risks if the problem is not addressed?
Needs identified
A needs analysis is performed, with the aim of translating a widely defined “desire” into one or more specific requirements with respect to the system level. The basic objective is to achieve a satisfactory needs analysis, which can be best done through a broad approach involving the end customer, consumer (or user) (if different from the customer), the main contractor or producer and the major suppliers (as appropriate). This is done by using methods such as conducting surveys and interviews, using appropriate checklists, applying process analysis tools, and other related techniques and methods.
Feasibility analysis
Through requirements analysis, you identify the functions that a system must perform. On the one hand, there can be only one function. On the other, there may be multiple functions to perform, some primaries and some secondaries. To ensure that a good design concept is established from the outset, all possible functions must be identified, and the most rigorous functions must be selected as the basis, to define design requirements at the system level. It is important that all possibilities are addressed to ensure that the appropriate overall technical approach is selected when considering the design. Considering that the design is part of the general, from strategic to operational, emphasizing the technical part of the design allows to have concrete elements of the SC operation.
Functional analysis
An essential element of conceptual and preliminary design is the development of a functional description of the system that serves as the basis for identifying the resources needed for the system to achieve its goals. At this point, the goal is to specify the “what” and not the “how”; that is, what needs to be achieved compared to how it should be done. Functional analysis is an iterative process of breakdown of requirements from the system level to the subsystem, and to the extent of the hierarchical structure that is necessary to identify the design criteria and constraints for the various system elements.
Operational requirements
Once the basic need and the selection of a feasible technical design approach are defined, it is necessary to project this information through a more complete description of the anticipated operational requirements of the system. The objective is to reflect the needs of the consumer in terms of system implementation, utilization, effectiveness and fulfillment of the desired mission.
System synthesis, analysis and optimization of the design
Synthesis refers to the combination and structuring of components, in such a way that it represents a feasible configuration of the system, is related to the design. Initially, synthesis is used to develop preliminary concepts and establish basic relationships between the various components of the system. Later, when sufficient decomposition and functional definition has been developed, synthesis is used to further define “how” in response to “what” requirements. Synthesis involves selecting a configuration that can be representative of the form that the system will ultimately take, although a final configuration certainly should not be assumed at this point.
Integration of design
The objective, from a systems engineering perspective, is to ensure that the right specialists are available at the required time and that their individual contributions are properly integrated throughout. The selection of domain specialists depends to a large extent on the specific requirements developed through the functional assignment and analysis process.
System testing and evaluation (validation)
The goal is to acquire a high degree of confidence, as soon as possible in the lifecycle, that the system will eventually work as expected. Acquiring this trust, through a physical replica of the system (and/or its components), can be quite expensive. Nowadays there are many functions that can now be performed with a computer simulation that previously required a physical simulation of the system, a pre-production prototype model or both.
4. Results and discussion
The case of application of the SEP is an RSC focused on the acquisition of MSW for its valorization, which must operate sustainably in the Mexican municipality of Nezahualcóyolt. Territorially, Nezahualcóyolt is divided into 179 Basic Geostatistical Areas [BGA]. The BGA is the basic unit of the geostatistical framework employed by the National Institute of Statistics, Geography and Informatics (INEGI by its acronym in Spanish) (INEGI, 2010).
4.1. Stage 1. Defining the problem of design and identifying needs
Definition of the problem
Nezahualcóyolt is located in the central area of Mexico (Figure 3), which is the area that generates the most MSW nationally, it were the municipality with the highest population density in 2010, with 17,555.5 inhabitants per km2 (INEGI, 2011). In that year, 820 t of MSW was collected daily and its per capita collection rate was 0.746 kg/habitant/day (SEMARNAT, 2013). By 2016, the daily collection of MSW was 1200 t, with a per capita rate of 1,153 kg/habitant/day (SEMARNAT, 2016). Although its per capita collection rate is less than 2.0 kg, Nezahualcóyolt is one of the municipalities that has had the largest increase in MSW collection from 2010 to 2016, its growth is 46%, exceeding the national growth average in this area. At the national level, this municipality ranks tenth in the increase in the collection of MSW, in the metropolitan area of the valley of Mexico occupies the third place. In the municipality there is a final disposition site for MSW that receives an average of 1,200,000 kg of MSW daily, occupying the fourth place in terms of the amount of MSW received nationally (INEGI, 2018).
About 35% of MSW in Mexico are susceptible to valorization, such as paper and cardboard (13.8%), glass (5.9%), plastics (10.9%), ferrous (1.1%) and non-ferrous (0.6%) metals, aluminum (1.7%) and textiles (1.4%). However, of the 1200 t of MSW generated daily it is estimated that only 10% will be recycled by formal and informal channels (SEMARNAT, 2013), the rest, about 1080 t/day permanently lose their value when deposited in landfills.
The problem is a conceptual design of RSC that allows to recover the recoverable MSW from the sources of generation and lead them to the industrial recovery points outside the municipality, meeting in its operation with the three dimensions of sustainability. The scope of conceptual design is a MSW acquisition network that retrieves materials from different generation sources and leads them to transfer centers, for later industrial valorization.
Identification of needs
The needs of the RSC focused on MSW susceptible of valorization come from the economic, environmental and social dimensions, in addition, applicable laws and regulations framework. In this way, for each of the three functions of the RSC-MSW, the needs of the four dimensions must be included, at least in their environment, as shown in Figure 4.
Design needs come from the desirable sustainability attributes for MSW RSC. These attributes are safety, health and freedom of action in the social dimension, coexistence, conservation, stability and protection in the environmental dimension and efficiency, productivity and resilience in the economic dimension. Compliance with the national, state and municipal regulatory framework also becomes a design need.
For all this, the design problem is the modeling of a MSW acquisition network that allows the most waste to be collected from the various sources of generation; in addition, this network must supply the MSW valorization industry with the quantity, quality and frequency demanded; all this minimizing the impacts that acquisition operations generate on social, economic and environmental environments.
4.2. Stage II. System feasibility and functional analysis
Feasibility analysis
The main sources of MSW generation in Mexico City are room houses (with 48% of the generation), economic units such as markets, shops and services (with 37%) and other sources of generation such as public squares, avenues and streets (15%) (SEDEMA, 2017). Table 1 shows the main sources of generation in the study area.
Table 1 Sources of MSW generation in Nezahualcóyolt
Source | % de MSW | Daily generation [t / day] | Generation per capita |
---|---|---|---|
Residential Houses | 48 | 576 t/day | 2.05 kg/ day/ Room Houses |
Economic Units | 37 | 444 t/day | 9.095 kg/ día/ Economic Units |
Source: Author own elaboration, based on INEGI (2011, 2016, 2017).
From these sources of generation are obtained the valorization materials of the MSW, which are those that by their characteristics have the technical, economic and environmental possibilities of being reinstated to a process or treatment to allow their valorization (Secretaría de Medio Ambiente, 2015). The most common materials that can be rated are shown in Table 2. In addition, the presence of these materials in the MSW and the estimated generation in the municipality of Nezahualcóyolt is shown.
Table 2 Materials recoverable from MSW
Materials | Presence in MSW (%) | Dally generation [t/day] | Uses such as: |
---|---|---|---|
Paper and Cardboard | 13.8 | 165.6 | Pulp of paper and cardboard |
Plastic | 10.9 | 130.8 | Agglomerates, Pellets, flakes |
Glass | 5.9 | 70.8 | Glass |
Metals | 3.4 | 40.8 | Metallic products |
Source: Author own elaboration, based on INEGI (2018), SEMARNAT (2013) and (NADF-024-AMB, 2013).
The points of acquisition of the MSW are the private collection centers [CC] established in the municipality. According to data from the National Directory of Economic Units in Nezahualcóyolt there are 281 Economic units oriented to the commercialization of some type of waste, which are concentrated in sector 43431 of the Industrial Classification System of North America (INEGI, 2016). These economical units mainly engaged in the specialized wholesale trade of waste materials for recycling, such as metal, paper, cardboard, glass, plastic. The business of CC is: 145 markets metal waste, 68 paper and cardboard waste, 60 plastic waste and 8 commercialized other waste. Glass is an MSW material that is not marketed in the municipality.
From the data shown in Graph 1 it can be established that the market for the marketing of recoverable MSW is attractive to CC established in the municipality: each CC of metal waste has a catchment potential of 281 kg per day of waste; CC of paper and cardboard can collect up to 2435 kg per day each; CC of plastic can capture up to 2180 kg per day. It is noted that 70 t of glass is being wasted daily because it does not have a RSC. This data shows the feasibility of MSW acquisition networks.graph1
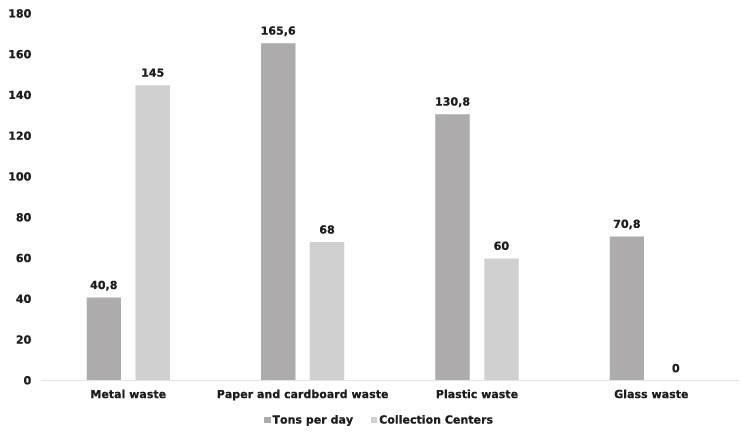
Graph 1 Amount of valorization susceptible MSW and Collection Centers. Source: Authorown elaboration, based on INEGI (2017) and INEGI (2016)
Functional analysis
Functional analysis is performed by functional disaggregation of RSC into more specific operations developed by particular agents. The agents involved in RSC functions are shown in Figure 5. Functional disaggregation identifies operational needs of the agents involved.
4.3. Stage III. Operational requirements and system synthesis
This stage describes the anticipated operating requirements of the system. The expectations of agents and stakeholders are translated into operational technical requirements for the operation of RSC.
Operational requirements
Table 3 shows the operational requirements are derived from the analysis of needs and functions, both internal, required for the operation and effectiveness of the system, as well as external, for sustained interaction with environmental, social and economic environments. Internal operational requirements are derived from the agents involved in the RSC, while external requirements come from stakeholders.
Table 3 Functional needs of RSC agents
Agent | Needs |
---|---|
Generation | Pay the lowest cost for collecting your MSWs. |
Have your MSW collected as often as possible. | |
Acquisition | Acquire the largest amount of recoverable MSW from generators. |
Get the maximum amount of handouts from generators. | |
Make the most of your MSW collection grants. | |
Supply the largest amount of MSW to the valorization industry. | |
Obtain the maximum sale price of the acquired MSW. | |
Operate at the lowest possible cost. | |
Valorization | Ensure a reliable supply of the acquirer (maximum quantity, quality and regularity). |
Supply of MSW at the lowest cost. | |
Operate at the lowest cost. | |
Take advantage of direct subsidies and tax incentives derived from the nature of its activity. |
Source: Author own elaboration.
The analysis of external requirements for a RSC to be sustainable must consider the needs of the stakeholders of the system. Stakeholders are any people or entities that are affected or concerned by RSC activities. Including these needs ensures the functionality of RSC in its relationship to economic, social and environmental environment. At this stage stakeholders are identified; their needs, interests and expectations; balance, reconcile and synthesize these aspects; integrate their needs with RSC strategies; identify the importance of each: power, influence, interest. The identification of stakeholders is shown in Table 4.
Table 4 Stakeholders identified in MSW RSC
Dimension | Stakeholders |
---|---|
Social | Employees of the RSC agents. |
Local community | |
Society as a whole | |
Economic | Other agents of the RSC-MSW or other RSC. |
Local economic units. | |
National economy | |
Environmental | Municipal, State and National Authorities |
Regulatory framework |
Source: Author own elaboration.
System synthesis
The synthesis of the conceptual design of the system is reflected through a set of indicators of the operation of the MSW RSC, which are detached from the operational requirements set out in step “II.b”. For the social dimension, the design is presented in Table 5, for the environmental dimension in Table 6 and for the economic dimension in Table 7.
Table 5 Integration of design in the social dimension
Social dimension | ||
---|---|---|
Requirement | Operational definition | Indicators |
Health | Workplace health risks, including heat, noise, dust, hazardous chemicals, unsafe machines, and psychosocial stress that cause occupational diseases and can affect the local community. | •Lists of social benefits provided to workers. |
•Lists of health services provided to workers. | ||
•Awareness of health issues | ||
•Workplace health risks. | ||
•Health risks to the local community arising from RSC operations. | ||
Leeway | Each person can dedicate himself to the legal activity he wants, without anyone being able to impose any form of work, or conditions other than those provided by law. | •Forced labor. |
Safety (and hygiene) | Prevention of occupational risks through the application of measures and the development of the activities necessary for the prevention of risks derived from work. | Awareness about security issues. |
• Knowledge of the steps / protocols to follow in case of emergency and accidents | ||
• Percentage of accidents / injuries in the organization. | ||
• Use of protective equipment. |
Source: Author own elaboration.
Table 6 Integration of design in the Environmental dimension
Environmental dimension | ||
---|---|---|
Requirement | Operational definition | Indicators |
Existence | Guarantee the existence of natural resources and favorable environmental conditions for future generations. | • Carbon Footprint |
• Water Footprint | ||
• Power consumption | ||
• Consumption of virgin raw materials | ||
• Required public area [m2] / available area /users of public space. | ||
Stability | It refers to the ecological balance and the harmonious relationship between man and living beings with their environment. | • The proportion of urban solid waste that is deposited in sanitary or controlled landfills |
• Tons of recovered waste / tons of waste generated | ||
• Air quality and stratospheric ozone in the last year. | ||
Conservation and Protection | It refers to the different ways that exist to regulate, minimize or prevent the damage that industrial, agricultural, urban, commercial or other types of activities cause to natural ecosystems. | •% of rural land lost per year for residential or industrial use |
• Volume of solid waste generated per year | ||
• Population growth rate per year | ||
• Adoption of measures for the responsible consumption of natural resources. | ||
• Number of protection laws passed at the municipal, state and national levels. | ||
• Number of environmental protection associations established in the territory. |
Source: Author own elaboration.
Table 7 Integration of design in the Environmental dimension
Economic dimension | |||
---|---|---|---|
Requirement | Operational definition | Indicators | |
Productivity | We understand productivity as the result we obtain from a process related to the resources used and the effort that this implies. | • Return on Assets. | |
• Return on equity | |||
• Inventory turnover. | |||
Efficiency | It involves the valuation of the largest amount of MSW at the lowest possible cost. | • Income / costs. | |
• MSW recovered [t] / MSW collected [t]. | |||
Viability | Probability that exists of carrying out what is intended or planned to be carried out, of actually achieving it. | • Cash Flow | • Cost structure |
• Reduction in public spending for waste management. |
Source: Author own elaboration.
Eighteen sustainability attributes were found in the literature, including very general indicators that are difficult to apply to particular production systems. In this work only nine of these sustainability attributes are taken as requirements, which are defined in Tables 5, 6 and 7. The main reason for not including further sustainability requirements is due to the marginality and informality conditions in which MSW RSC agents operate in the study area. Only 1% of identified CC are in the Federal Taxpayer Register, the operations of these centers are informal which limits their economic analysis.
To choose the appropriate indicators for procurement operations it is necessary to rely on the observation of real systems in operation or in the consultation of experts through participatory techniques. The scope of this work is limited to the structuring of indicators in requirements and dimensions, without having specific measurements to assess the performance of the proposed design.
4.4. Stage IV. Design integration and validation
Integration of design
The acquisition network for the eligible MSW RSC must operate in two phases, as shown in Figure 6: one local collection and one consolidated collection network operating at the municipal level. The first phase is characterized by the movement of waste over short distances by walking, in human and animal traction vehicles and even low capacity motor vehicles (up to 1 t).
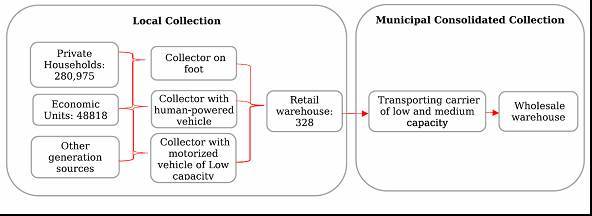
Figure 6 Conceptual design of the RSC for MSW in the municipality of Nezahualcóyolt, Mexico. Source: Author own elaboration.
The network that makes the first phase possible starts with generators that can produce up to 420 t of valorization susceptible materials per day. Each BGA produces 2.4 t/day of materials, which demands crews of about 20 collectors: a collection of 100 kg to 150 kg per day makes the activity of these collectors profitable. Maps 1 and 2 show the centroids of each BGA, colored in purple, which are the first nodes of the designed acquisition network.
Each retail collector, shown in red on Maps 1 and 2, has on average a collection area of 100 m2 can receive up to 1.4 t of valorization susceptible materials daily. The identified 281 CC cannot cover the MSW offer, so at least 47 more CC are required to cover missing BGA. Each CC demands three workers. To move the materials generated in each BGA, low-capacity vehicles (up to 3.5 t) are required, a travel of one of these vehicles is sufficient to move the collected waste inside each BGA.
Without posing a routing problem for this network, which goes out of reach of work, a low-capacity vehicle can make up to 6 trips per day, so a fleet of 30 carriers is sufficient. In addition to the operator, each unit requires an auxiliary for loading and unloading materials. To consolidate materials and transfer cargo to medium capacity vehicles (3.5 to 7.5 t) three transfer centers are required: one for plastic, one for paper and cardboard, and one more for metals and other special handling waste. The configuration of this network generates around 8000 jobs.
The second phase travels average distances and occupies low (up to 3.5 t) and medium capacity motor vehicles (more than 3.5 and up to 7.5 t). The local bulking phase should be the most agile to reduce affectations to the surrounding population, while the second phase must be efficient in lowering large-scale storage and transportation costs. Maps 1 and 2 show the acquisition network for local collection, this network constitutes the RSC to recover the MSW in the municipality. Map 1 shows the northern area of the municipality of Nezahualcóyolt and Map 2 shows the eastern part of the municipality. The red dots represent the centroids of the MSW generation sources, the BGA centroid, which are the points where the RSC starts. These points are connected by the municipality’s road infrastructure to retail CC. Retail CC are colored with violet. The roads in red represent the routes for waste traffic in the municipality.
System testing and evaluation (validation)
A validation of the conceptual system includes (Landry, Malouin, and Oral, 1983): the identification of stakeholders; formulating the objectives of the system; the determination of critical factors; trust in the model and; cost elements. For this conceptual design, three participatory techniques occupied by Hanington and Martin (2012): Contextual research for stakeholder identification and identification of critical factors are proposed. Brainstorming, with graphic organizers for formulating system objectives.
Realistically, a complete system evaluation, in terms of meeting the consumption requirements initially specified, cannot be achieved until the production system comes into operation. That is why an extension of the research is the test of the system in the field.
A RSC design like the one proposed in this work can reduce the volume of MSW that is finally available by 30%, increasing the rate of acquisition of materials rated in the MSW. From the figures presented, particularly in the feasibility analysis section, it was shown that about 240 t of valorization susceptible materials are wasted because they do not have an acquisition system. This involves reducing 32 MSW collector trucks (each truck with a capacity of 7.5 t) in the municipality, reducing waste management costs and CO2 emissions. The location of retail CC in the centroids of each BGA reduces collection distances by establishing more agile MSW flows that reduce the impact of managing valorizing materials. In addition, it concentrates the flow of materials in certain roads of the municipality, reducing the impact on the social environment by pollution of the urban landscape.
5. Conclusions
The problem of the recovery of MSW from the conventional flow from cradle to grave, characteristic of traditional SC, is a cornerstone for the disruption between linearity and circularity of production models in a framework of sustainable development. The RSC that allows MSW recovery is a type of SC with strong social and environmental implications. These implications pose a set of particular MSW RSC issues that go beyond operational efficiency and profitability.
The proposal presented in this work enriches the discussion on the sustainability of traditional SC and the need to expand its functions and scopes, particularly with regards to its shared responsibility for post-consumption waste generation. The functional disaggregation of SC direct and reverse flows, carried out through the application of the systems approach, shows the variety required for the implementation of circular economy models and the need to expand design tools. The Systems Engineering Procedure can be a useful tool for RSC managers and agents in their quest to contribute to sustainable development and MSW reduction.
The RSC design obtained can reduce by 30% the volume of MSW sent to final disposal, generating around eight thousand direct jobs. The RSC design establishing more agile MSW flows that reduce the impact of handling these materials in the area and driving circular economy models for metal waste, plastics, paper and cardboard, and even glass.
It is a limitation of this work to only present conceptual design, which is one of several possible designs for a MSW RSC. It will be an extension of this work to test the design, through a more thorough feasibility study, which constitutes the post SEP stage.