Introduction
The growth of the world population and the increase of mega-farming to meet nutritional needs have contributed to the spread of bacterial infectious diseases worldwide (Lazcka, et al., 2007; Yue, et al., 2016; Muraoka, et al., 2011). Every year, millions of people are infected with pathogenic bacteria after consuming food contaminated, mainly with Staphylococcus aureus, a Gram-positive bacterium that causes skin infections (including abscesses), gastroenteritis, and food poisoning (Velusamy, et al., 2010; Liu, et al., 2017). In this context, the detection of bacterial pathogens is crucial in the fields of medicine, food safety, and public health, among others (Amiri, et al., 2018).
Several methods have been described to detect bacterial pathogens including routine bacterial agar plate culture, polymerase chain reactions (PCR), enzyme-linked immuno sorbent assay (ELISA), and molecular biology protocols based on nucleic acids (Xu, et al., 2012; Escamilla-Gómez, et al., 2007). Although the effectiveness and robustness of these methods are not under discussion, most of them are costly, complex, time-consuming, and have low sensitivity. Therefore, it is very important to develop innovative strategies to improve their performance.
Electrochemical sensors are powerful analytical tools that play a key role in the detec tion of environmental and biomedical analytes when combined with enzymes as biorecognition elements due to their simplicity, in situ real time analysis, rapidity, and convenience (Gao, et al., 2007). Enzymatic biosensors based on horseradish peroxidase (HRP) has received much attention in the last two decades for their high specificity towards hydrogen peroxide (Chen, et al., 2006). However, this type of peroxidase (POD) is potentially inactivated at high H2O2 concentrations, which limits its bioelectrocatalytic activity. Therefore, the search for new alternative sources of POD with improved biocatalytic properties is a challenge for biotechnological applications. Recently, a novel POD from Guinea grass (Panicum maximum) (GGP) was isolated and biochemically characterized (Centeno, et al., 2017). GGP was integrated with zinc nanoparticles in screen-printed platinum electrodes to detect H2O2, a molecule of environmental and biological significance that can be used as a biomarker in cellular apoptosis processes (Uribe, et al., 2019). In another study, GGP was integrated on the surface of screen-printed carbon nanotubes electrodes for triclosan detection showing an electrocatalytic activity of 370 mV at a redox potential and a linear response between 20 mM to 80 mM (Orduz, et al., 2019).
Several researchers have developed novel electrochemical biosensors for measuring bacterial populations based on common electrochemical methods such as voltammetry, amperometry/potentiometry, and impedance spectroscopy. For example, Pérez, et al. (2001) developed an amperometric culture-based method using 4-aminophenyl-p-D-galactopyranoside hydrolysis on an electroactive compound (4-aminophenol) for the rapid detection of viable Escherichia coli in water. Using a similar approach, Nistor, et al. (2002) fabricated a cellobiose dehydrogenase sensor to test E. coli cell viability. Alexandre, et al. (2018) achieved the rapid determination of Salmonella typhimurium in milk samples with a low limit detection (10 CFU/mL-1) using a specific biosensor developed from modified gold screen-printed electrodes. An electrochemical method using cyclic voltammetry successfully detected polyaniline-labeled E. coli O157:H7 with 7 CFU/mL (Setterington, et al. 2011) while real-time monitoring of bacterial contamination was done with a piezoelectric microelectrode array system exhibiting a detection limit of 102 CFU/ mL-1 and analysis time of 5 h. (Ren, et al., 2014). On the other hand, researchers have used impedimetric detection methods to measure impedance changes of small amplitude with a sinusoidal voltage signal as a frequency function while an anti-E coli antibody was immobilized on a gold electrode exhibiting a linear relationship between the electron-transfer resistance and E. coli concentration logarithmic values in the range of 3x103-3x107 CFU/mL-1 (Wang, et al., 2012). Other teams have used a microfluidic inmmunosensor based on nanoporous membranes to detect S. aureus through the blocking of the electrolyte current and monitored the change by impedance spectroscopy (Tan, et al., 2011).
These electrochemical techniques take advantage of the electroactive molecules excreted or taken by microorganisms to measure the concentration of different bacterial populations indirectly. S. aureus produces different toxins and enzymes which are virulent factors and possess many defense mechanisms based on enzymes such as catalases, which facilitate cell detoxification by neutralizing the bactericidal effects of H2O2 (HIS, et al., 2014). Catalase has been useful to differentiate between staphylococci and streptococci and, besides, it promotes the conversion of hydrogen peroxide to water and molecular oxygen. By using the S. aureus catalase it is possible to detect hydrogen peroxide and, thus, measure its consumption to determine the concentration of bacterial populations. Interestingly, this mechanism has been used for the rapid electrochemical quantification of Staphylococcus aureus through the indirect consumption of H2O2 by catalase in sample foods using platinum electrodes (Majumdar, et al., 2013). Despite all the advantages of platinum electrodes, there are still limitations that require novel strategies like the incorporation of PODs, enzymes that require H2O2 to carry out their biocatalytic functions, on the surface electrode to improve their performance.
The aim of the present study was the electrochemical detection of S. aureus through the monitoring of H2O2 consumption by the S. aureus catalase using screen-printed gold electrodes (SPGE) modified with cysteine and peroxidase from Guinea grass (Panicum maximum).
Experimental
Materials and chemicals
Screen-printed gold electrodes (SPGE, 220AT) were provided by DropSens (Oviedo, Spain). They are composed of a gold surface (working electrode, 4 mm of diameter), a silver pseudo-reference electrode, and a carbon electrode as an auxiliary electrode. All chemicals and culture media used in this study were commercially purchased from Sigma-Aldrich Corp. and Merck.
Extraction and partial purification of Guinea grass peroxidase
POD was partially purified from Guinea grass leaves as described by Rodriguez, et al. (2002). Briefly, leaves were triturated and homogenized with constant stirring in 30 mM phosphate buffer, pH 8.0 for 1 h at 25°C. The homogenate was filtered and centrifuged (7000 rpm, 15 min) and the solid was discarded. Then, the polyphenolic compounds were extracted by using a two-phase system containing 14% (w/v) poly (ethylene glycol) and 15% (w/v) (NH4)2SO4. The aqueous phase was applied directly to a Sephadex G-75 column (1.5 x 35cm) previously equilibrated with TRIS buffer 1mM, pH 8.0. Finally, the fractions containing the POD were concentrated by ultracentrifugation (7000 rpm for 25 min at 4°C) with Amicon membranes (10,000 kDa), and the activity was measured by UV-vis spectrophotometry following the oxidation of guaiacol 10 mM (e470= 5200 M cm-1) in the presence of H2O2 4 mM and 10 µL of the enzyme. Protein concentration was measured with the Bradford assay (Bradford, et al., 1976).
Preparation of the bacterial culture
We cultured a pre-inoculum of S. aureus ATCC 29213 and E. coli ATCC 25922 in Luria-Bertani (LB) and MRSA in Müller-Hinton (MH) agar at 37°C and 200 rpm during 12 h. Then, the culture of each strain was set at 0.5 with the MacFarland scale (108 CFU/mL-1) The bacteria were centrifuged at 6000 rpm for 5 min to remove the supernatant and then washed with PBS three times. Finally, bacterial culture was serially diluted in a range between 3x10-2 and 3x108 CFU/mL-1.
Modification of screen-printed gold electrodes
Before immobilizing the cysteine and the GGP, gold electrodes were carefully cleaned with a mild version of a piranha solution (50 mM H2SO4 / 25% H2O2) for 10 min and rinsed with milli-Q water. For the SPGE modification, a self-assembly monolayer of cysteine was formed on the surface of bare gold electrodes by soaking them in 1 mM cysteine solution for 10 min at room temperature (this electrode was denoted as SPGE-cys). Then, these SPGE-cys were gently rinsed with PBS buffer 10 mM pH 7.0 to remove free GGP and cysteine. To modify SPGE-cys with GGP, 4 µL of homogeneous EDC solution (25 mM) were mixed with 6 µL of GGP and magnetically stirred for 1 h. The conjugation reaction was carried out in the presence of sulfo-NHS to avoid competing reactions (Castillo, et al., 2014). Then the GGP activated with EDC was further dropped on top of the electrode surface. After 4 h of incubation at 4°C, the modified electrode was washed with phosphate buffer 10 mM pH 7.0 to eliminate EDC and GGP. Figure 1 shows a schematic representation of the SPGE modification with cysteine and GGP and the principle of S. Aureus detection.
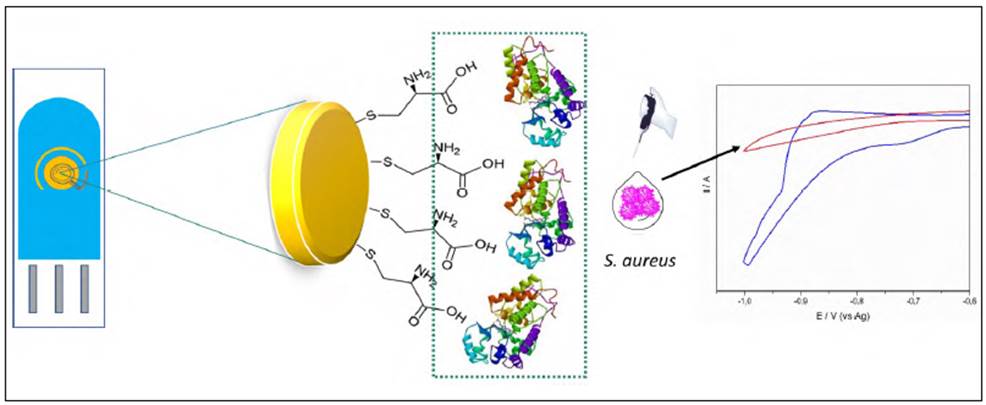
Figure 1 Schematic representation of gold modified electrode with GGP and cysteine and electrochemical principle of detection of S.aureus. (A) Gold screen printed electrode modified with cysteine and GGP; (B) Cyclic voltammograms of the detection of H2O2 1 mM in presence (red line) and in absence of S. aureus (blue line).
Electrochemical studies
Electro-analytical measurements were done with an Autolab PGSTAT101 (Echo Chemie, Utrecht) controlled by the NOVA 1.10.1.9 software (Metrohm, Filderstand). All the experiments were carried out at room temperature; before each cyclic voltammetry (CV) experiment, the solution was degasified with pure N2 and magnetically stirred for 30 s, then, ascorbic acid (oxygen scavenger) at 0.05 mM was added to the cell measurement.
CVs were used to study the electrocatalytic activity of GGP towards H2O2 reduction and to evaluate the electron transfer process by using potassium ferrocyanide ((Fe(CN)6)3-/4-10 mM and KCl 100 mM contained in a PBS solution 10 mM (pH 7.0) as a redox probe; they were carried out at a scan rate of 100 mV/s.
Electrochemical sensing of H 2 O 2 consumption by Staphylococcus aureus in pure culture and milk samples
The SPGE-cys-GGP electrodes were immersed in each of the S. aureus solutions in the presence of 1mM of H2O2. Then, the electrode was left for 3 minutes to evaluate the response at the rapid growth phase of bacteria. CVs at a range potential between 800 and -800 mV were measured for each concentration of the bacteria.
Considering the pathogenicity of S. aureus in contaminated food samples, we analyzed milk samples pre-inoculated with the bacteria (3x104 CFU/mL-1) as real samples to evaluate the performance of the SPGE-cys-GGP electrode.
Results and discussion
Partial purification of Guinea grass peroxidase
GGP is an anionic peroxidase with a molecular weight of 35 KDa; like many other PODs from plants it has a high concentration of coloured compounds that were removed by using the two-phase system PEG-ammonium sulfate.
As shown in table 1, after eliminating the pigments, GGP specific activity was 217.7 U mg-1. An additional increase in the purification factor was observed after exclusion chromatography and ultrafiltration with a specific activity of 351,7 and 470,8 U mg-1, respectively, which agrees with previous results by Centeno, et al. (2017).
Electrochemical characterization of screen-printed gold modified and unmodified electrodes
The electrochemical response of SPGE to ferrocyanide (Fe(CN)6)-4/ferricyanide (Fe(CN)6)-3 redox probe system was established to monitor the barrier created after each modifica tion step on the surface electrode. Figure 2A shows the ferro/ferricyanide couple CVs for the modified and unmodified gold electrodes. The CVs of the unmodified and modified electrodes with cysteine and cysteine-GGP, respectively, evidenced well-defined redox signals (ΔEp= 111 mV, ΔEp= 108 mV, and ΔEp= 74 mV for SPGE, SPGE-cys, and SPGE-cys-GGP, respectively), which reflects a quasi-reversible behavior controlled by the diffusion of the electroactive species moving to the electrode surface. The peroxidase-SPGE-modified electrode improved the transfer between GGP and the electrode compared to the bare gold electrode.
Bioelectrocatalytic reduction of H 2 O 2 by modified and unmodified electrodes
To evaluate the reduction of H2O2 by GGP, we measured CVs in the presence and in the absence of H2O2 1mM for bare SPGE electrodes and those modified with peroxidase. There was no appreciable change in the current reduction peak for the bare gold electrode and, as expected, when GGP sensed H2O2 we observed a decrease in the reduction peak around -457 mV (Figure 2B) thus demonstrating the role of PODs as an efficient biocatalyst. Most of the electrochemical biosensors based on plant peroxidases have shown similar potential for bioelectrocatalytic reduction of H2O2 (Gao, et al., 2007; Castillo, et al., 2006; Villamizar, et al., 2016). These results suggest that the electrocatalytic reduction of H2O2 by SPGE-cys-GGP has potential utility for monitoring the decrease in hydrogen peroxide concentration to detect catalase-positive bacteria.
Detection of Staphylococcus aureus by screen-printed gold electrode modified with cysteine and Guinea grass peroxidase
Staphylococcus aureus is a Gram-positive catalase-producing bacterium, an enzyme that allows the bacteria to better resist intra- and extra-cellular death by hydrogen peroxide. On the other hand, GGP needs hydrogen peroxide to oxide its typical substrates; therefore, these two features determine the sensing principle of the hydrogen peroxide consumed by the GGP in the absence or in the presence of S. aureus. This sensing principle was also used by Majumdar, et al. (2013) to detect S. aureus using platinum electrodes.
To evaluate the response of the SPGE-cys-GGP electrode we studied it in S. aureus concentrations ranging between 3x102-3x108 CFU/mL/-1 (Figure 1S,https://www.raccefyn.co/index.php/raccefyn/article/view/1019/2836).
CVs (Figure 3A) were analysed around -780 mV for the quantitative determination of S. aureus. As shown in figure 3B, the reduction current of H2O2 decreased while S. aureus concentration increased due to the consumption of the H2O2 by the catalase present in the bacteria. A linear relationship existed between the reduction of the H2O2 current and the S. aureus concentration logarithm. The linear fit obtained was I(mA) = 2,83x10-5 (UFC/ML-1) - 0,02091, with a 0,9816 correlation coefficient. The SPGE-modified electrode exhibited a wide linear range (102 to 108 CFU/mL-1), a sensitivity of 0.020 mA CFU-1, and a detection limit of 102 CFU/mL-1.
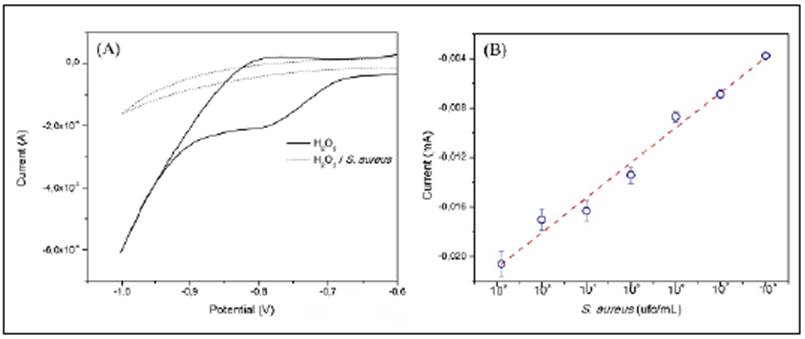
Figure 3 (A) Cyclic voltammetry response of SPGE-cys-GGP in 1 mM H2O2 in the presence of S. aureus 3x108 cfu mL-1 (dash line) and in the absence (solid line), scan rate 50 mV s-1, (B) Reduction current response of H2O2 1 mM by SPGE-cys-GGP at different concentrations of S. aureus, -780 mV potential.
To evaluate the ability of our sensor to differentiate between Gram-positive and Gram-negative bacteria, we followed the same procedure as with S. aureus but with E. coli, a Gram-negative, facultatively anaerobic, rod-shaped, and catalase-negative bacteria that we used as a negative control (Figure 4). It is worth mentioning that there are other types of catalase-positive bacteria that can affect the selectivity of the sensor, as well as other types of interferences (Han, et al., 2019; Xu, et al., 2018). The purpose of this experiment was to evaluate the ability of the sensor to differentiate the electrochemical response of S. aureus from E coli.
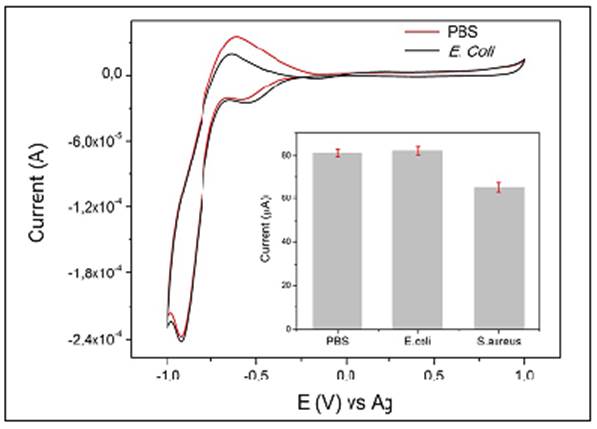
Figure 4 CV response of SPGE-cys-GGP in PBS + H2O2 1 mM and in the presence of E. coli culture + H2O2 1 mM; inset: comparison of H2O2 reduction current by SPGE-cys-GGP in PBS, E. coli, and S. aureus, 3x104 CFU/mL-1
The electrocatalytic current of H2O2 by the GGP on a gold electrode in PBS and E. coli 104 CFU/ML-1 remained almost unaffected but exhibited notable differences compared with S. aureus at the same concentration. The data indicated a cathodic current (Ic) value of nearly 73 µA for S. aureus, whereas PBS and E. coli exhibited an Ic of 90 and 91 µA, respectively (Figure 3A).
Additionally, the mean detection time using our modified gold electrode was about 20 min, shorter than routine microbiological methods (48 h). These results indicated that the modified gold electrode we developed had a rapid electrochemical response. Table 2 com pares the analytical performance in previous reports and the results obtained in this study.
Table 2 Comparison of electrochemical methods for sensing bacterial populations
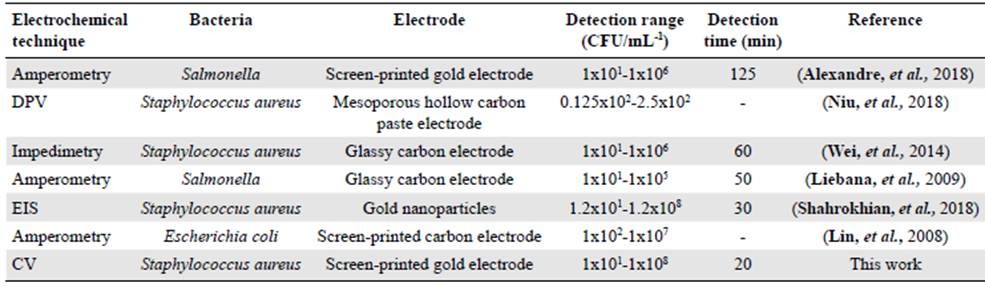
DPV: Differential Pulse Voltammetry; EIS: Electrochemical Impedance Spectroscopy; CV: Cyclic Voltammetry
To test the ability of our SPGE-cys-GGP electrode in real samples, we used a brand of commercial milk from a local store. The milk sample was previously spiked with H2O2 1 mM and S. aureus at a concentration of 104 CFU/ML-1 and we registered the electrocatalytic reduction current of H2O2 by GGP. A milk sample with no S. aureus was used as a control.
As is shown in figure 5B, the reduction current for H2O2 registered a value of 30 µA while in the milk sample with S. aureus it was 21 µA indicating that the GGP-SPGE-modified electrode was suitable for sensing S. aureus in real food samples.
Conclusions
A sensitive and rapid electrochemical method for the detection of S. aureus was successfully developed. The modification of the gold bare electrode with a cysteine layer and guinea Grass peroxidase increased the electron transfer and the electrochemical signal allowing direct detection of S. aureus in pure culture and milk simples without any pre-enrichment method with short times to obtain an electrochemical response. These features make the SPGE-cys-GGP electrode a promising bioanalytical tool for rapid and easy detection of S. aureus in food samples.