INTRODUCTION
Microplastic (<5 mm) pollution has gained major concern in the last decade as its presence has been found in a wide range of aquatic ecosystems around the globe. Due to their small size and ubiquity in the environment, they are subject to be mistaken for prey (Ory et al., 2017) and ingested by marine organisms. Ingestion has been reported in many organisms, including zooplankton (Sun et al., 2018), mollusks (De-la-Torre et al., 2020), fishes (Ory et al., 2018), and top predators (Thiel et al., 2018; Santillán et al., 2020). Microplastics leach plastic additives and interact with other hazardous chemicals in the environment (Torres et al., 2021; Hajiouni et al., 2022), like polycyclic aromatic hydrocarbons (PAH), polychlorinated biphenyls (PCB), organochlorine pesticides, and pharmaceuticals (Rochman et al., 2014; Camacho et al., 2019), that could compromise the health and population of many marine species and threatening food security (De-la-Torre, 2020).
Marine bivalves are filter feeders susceptible to ingestion and accumulation of microplastics in water and are a very popular shellfish served as seafood (Cho et al., 2019). These characteristics make them suitable as a route of human exposure to microplastics by consumption. Previous studies have investigated microplastic abundance in some mussel, oyster, scallop, and clam species sold in markets and fisheries globally (De Witte et al., 2014; Mathalon and Hill, 2014; Renzi et al., 2018, Cho et al., 2019) indicating microplastic contamination in shellfish for human consumption is generally occurring. However, the effects of human intake could pose are still poorly studied. In Latin America, only a few studies have characterized microplastics contaminating food products (e.g., fish, salts, foodstuffs) and drinking water (Kutralam-Muniasamy et al., 2020).
The mussel Aulacomya atra (Molina, 1782), locally known as "choro", is the second most-consumed bivalve species in Peru (PRODUCE, 2018) and is of commercial relevance in the region. Its distribution encompasses the southeast Pacific and southwest Atlantic Oceans coasts, including Peru, Chile, Argentina, Uruguay, and Brazil. This species is commonly eaten raw and thus presenting a higher chance of microplastic ingestion. Microplastic pollution is still poorly studied in Peru (De-la-Torre et al., 2020b), mainly focusing on marine sediments, non-commercial mollusks, and coastal fish species (Purca and Henostroza, 2017; lannacone et al., 2019), while dietary intake from shellfish and seafood remains unknown. To assess this issue, the objective of the present study was to report the abundance and characteristics of microplastics in commercial bivalve A. atra sold in fisheries from three Peruvian provinces. Additionally, a preliminary estimation of dietary intake from A. atra consumption was presented. We aim to provide initial insights into microplastic exposure through shellfish consumption in Peru.
MATERIALS AND METHODS
Sampled organisms were purchased from fishery markets located in three major Peruvian coastal provinces, Huarmey (S1), Lima (S2), and Pisco (S3), from March to April 2019 (Fig. 1). Lima is located within the region of Lima, and is the most populated province of Peru, accounting for around 30 % (~10 million people) of the total Peruvian population. Recent studies have reported a pronounced presence of marine litter along the coast of Lima, mainly associated with anthropogenic activities (De-la-Torre et al., 2021a; Dela-Torre et al., 2021b). Pisco and Huarmey belong to the region of Ica and Ancash, respectively, and are known for their highly active fishing activity and commerce. Following the sampling strategy by Cho et al. (2019), thirty (n = 30) A. atra individuals were purchased from several stores belonging to the main fishery market in each province (except for Huarmey, where only one store had A. atra for sale). The bivalves were delivered in plastic bags. The collected samples were stored in a clean cooler box with ice and transported to the laboratory in Lima. In the laboratory, samples were measured (valve length) and weighted (wet weight of the soft tissues).
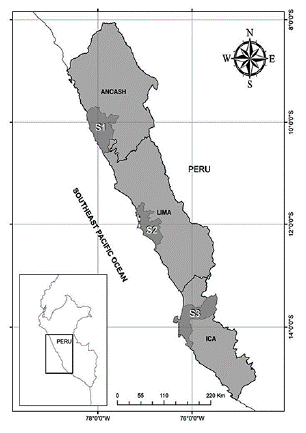
Figure. 1 Map of Peru displaying the three sampled provinces within their corresponding regions. S1: Huarmey province (An-cash Region), S2: Lima province (Lima Region), S3: Pisco province (Ica Region).
The extraction of microplastics from the soft tissues followed Protocol 1b as described by Dehaut et al. (2016) with minor changes. In brief, the soft tissues were extracted using a scalpel and placed in standard screw cab test tubes (two individuals were pooled). The tubes were filled with 10 % (w/v) potassium hydroxide (KOH) and incubated for 24 hours at 60 °C. The digestate was vacuum filtrated through a 20-25 urn pore filter paper (Whatman). Filters were placed in closed glass Petri dishes until visual identification.
To reduce external contamination, rigorous contamination prevention measures were conducted (Dioses-Salinas et al., 2020). Cotton lab coats and latex gloves were always worn. All the equipment and glass materials were rinsed with filtrated distilled water twice before use. Surfaces were wiped clean, and samples were immediately covered if they were not in use. For every batch of organisms, three blanks were prepared: (1) distilled water blank, (2) 10 % KOH blank, and (3) airborne blank. The airborne blank evaluated environmental microfiber contamination of the samples by placing a wet filter in a petri dish on top of the working table for as long as the sample treatments lasted and then scanned under a stereomicroscope. Particles found in the blanks matching morphological type and color than the ones extracted from the samples were subtracted from raw data.
Optical identification of microplastics was performed by scanning filters using a stereomicroscope and identifying their physical characteristics, like color, structure, and geometry, and lack of biological features (Hidalgo-Ruz et al., 2012; Desforges et al., 2014). Microplastic abundance, morphological type (fiber/line, fragment, film, or microbead), and color (red, blue, green, white, black, and purple) were recorded.
A larger segment of microplastics identified (>1 mm) was extracted and further analyzed using a Fourier transform infrared spectrometer (Perkin-ElmerTM) coupled with Universal attenuated total reflectance (ATR-FTIR) accessory set at wavelengths in the range of 600-4000 cm-1 and 30 scans. Recorded spectra were automatically compared with reference spectra from the FTIR library. Reference polymer spectra with a percentage of similarity higher than >75 % were accepted.
Microplastic concentration was expressed in microplastics per individual (MP ind-1) and microplastics per gram of soft tissue wet weight (MP g-1) ± standard error of the mean (SEM). Shapiro-Wilk test invalidated the assumption of the normality of the data (p < 0.05). Thus, Kruskal-Wallis and Dunn's multiple comparisons post hoc tests were conducted to compare microplastic abundance in A. atra between the three provinces. The significance level was set to 0.05 for all the analyses. Graphs and statistical tests were performed using GraphPad Prism (version 7.00 for Windows).
RESULTS
The mean length of the A. atra samples from S1, S2, and S3 were 5.87 ± 0.04 cm, 7.06 ± 0.12 cm, and 6.67 ± 0.08 cm respectively. All samples from the three provinces were contaminated with microplastics (Fig. 2), ranging from 1 to 11 per individual. The overall average microplastic abundance was 3.02 ± 0.36 MP ind-1 (0.56 ± 0.08 MP g-1). S2 presented the most contaminated bivalves on average, with 5.33 ± 0.70 MP ind-1 (1.04 ± 0.17 MP g-1), while S3 (2.20 ± 0.31 MP ind-1, 0.31 ± 0.04 MP g-1) and S1 (1.53 ± 0.24 MP ind-1, 0.34 ± 0.06 MP g-1) showed similar concentrations (Table 1). The three blanks showed mean microplastic contamination of 0.66 MP blank-1 and ranged from 0 to 2 microplastics per blank. Previous studies have considered <1 MP blank-1 as an accepted mean external contamination (Li et al., 2015), thus QA/QC measures deemed sufficient.
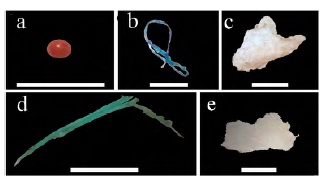
Figure 2 Microplastics of different morphological types and colors found in A. atra. a: Red microbead, unidentified polymer. b: Blue fiber, identified as polyester. c: White fragment, identified as polystyrene (PS). d: Green line, identified as polypropylene (PP). e: White film, identified as polyethylene (PE). Scale bar indicates 1 mm.
Table 1 Overall abundance, types, and color of the microplastics found in market A. atra samples in the three Peruvian provinces.
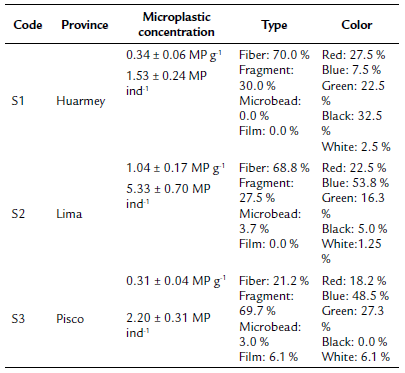
Fiber/lines were the most abundant morphological type (58.8 % of the overall abundance) and were dominant in S1 (70.0 %) and S2 (68.8 %), while fragments were the majority in S3 (69.7 %). Films and microbeads had a low occurrence in the three sites (<10 %). Regarding color, blue (40.5 %), red (22.9 %), green (20.3 %), and black (11.1 %) were the most representative colors for the overall microplastic abundance. Although blue was the most common color in S2 (53.8 %) and S3 (48.5 %), black was dominant in S1 (32.5 %).
There were significant differences in the microplastic abundance across provinces in terms of MP ind-1 (Kruskal-Wallis test, Chi-sqr = 24.04, p = < 0.0001) and MP g-1 (Kruskal-Wallis test, Chi-sqr = 17.61, p = 0.0001). Post hoc comparisons using Dunn's tests indicated that S2 was significantly different than S1 and S3 in terms of MP ind-1 and MP g-1. However, S1 did not significantly differ from S3 in terms of MP ind-1 and MP g-1 (Fig. 3).
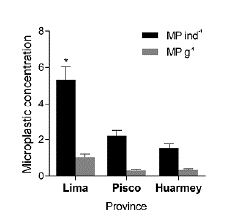
Figure 3 Bar graph displaying microplastic concentration (mean ± standard error of the mean) in Aulacomya atra from three Peruvian provinces. Asterisk indicates significant differences.
For ATR-FTIR analysis, only larger microplastics (>1 mm) were analyzed. These were seven fiber/lines, one film, and three fragments. The reduced number of selected particles was not suitable for statistical analysis. Results indicated that the 11 particles were confirmed to be synthetic polymers. The polymer identities were polyester (five fiber/lines), polypropylene (two fiber/lines), polyethylene (one film and two fragments), and polystyrene (one fragment). All the resulting spectra were accepted with a match of >75 % with the reference spectra.
DISCUSSION
The results ofthe present study are comparable to previous ones. Van Cauwenberghe and Janssen (2014) found 0.47 ± 0.16 MP g-1 in market oyster Crassostrea gigas (Thunberg, 1793) from France, similar to the overall abundance in the present study (0.56 ± 0.08 MP g-1). Although lower concentrations, other studies reported 0.35 MP g-1 in market Mytilus edulis (Linnaeus, 1758) from Belgium (De Witte et al., 2014), 0.34 ± 0.31 MP g-1 in Venerupis philippinarum (Adams and Reeve, 1850) from South Korea (Cho et al., 2019), 0.16 ± 0.18 MP g-1 and 0.13 ± 0.16 MP g-1 in V. philippinarum and C. gigas, respectively, from Canada (Covernton et al., 2019). A more recent study carried out in Peru, however, reported a concentration of 1.64 ± 0.08 MP g-1 in A. atra from different fisheries in Lima (Valencia-Velasco et al., 2020). In our previous study, we determined a mean microplastic concentration of 0.13 ± 0.03 MP g-1 in Argopecten purpuratus (Lamarck, 1819), purchased from fisheries in Lima (De-la-Torre et al., 2019). Table 2 summarizes the results from the present and previous studies.
Expressing microplastic concentration in MP g-1 (wet weight) may be subject to bias due to the size and age of different species. However, many studies opted for expressing their results in MP g-1 only. Standardization of the unit of expression and methods is required for further studies. We suggest that further research include MP ind-1 as one of the MP abundance units. Thus, making results more comparable among studies. In the present study, 11 microplastic samples (>1 mm) were analyzed by ATR-FTIR. However, it is necessary to analyze a more consistent subset of sampled microplastics (with a special focus on those smaller than 500 µm) to provide reliable data (Ivar do Sul, 2021). One of the main flaws in the studies conducted in Peru is the lack of rigorous analytical analyses. An important limitation in microplastic monitoring is driven by the lack of advanced analytical equipment readily available in developing countries (Alimi et al., 2021).
Fibers have been mostly reported as the dominant microplastic type in market bivalves (De Witte et al., 2014; Mathalon and Hill, 2014; Van Cauwenberghe and Janssen, 2014; Li et al., 2015, 2016; Rochman et al., 2015; Vandermeersch et al., 2015; Renzi et al., 2018) except for Cho et al. (2019), where fragments were the most abundant. As microfibers shed from laundering clothes Browne et al. (2011), wastewater treatment plants (WWTPs) are key to the release of microfibers to ocean waters (Sun et al., 2018). This is supported by the ATR-FTIR results since polyester (most of the fiber/line microplastics analyzed) is a common material used in clothing. Other sources of fiber/lines are the degradation of disposed fishing nets and lines. It is plausible that contamination with fiber/lines would be associated with fishing techniques requiring nylon- or polypropylene-based nets and gear. Moreover, the discharge point from the largest WWTP in Lima (La Chira) near the finishing grounds may be a focalized source of microfibers into coastal waters (De-la-Torre et al., 2020a). It is important to consider the supply chain and handling process as a possible source of contamination due to the lack of hygiene standards (Barboza et al., 2018).
Seafood contamination serves as one major pathway for microplastics to reach the human gastrointestinal tract. However, Catarino et al. (2017) suggest the ingestion of microplastics through contaminated mussels is reduced compared to airborne microfibers that fall into our meal. Despite this, microplastic intake through contaminated seafood ingestion varies among regions and eating habits. Cho et al. (2019) estimated that the Korean population intakes 212 MP person-1 year-1, while high shellfish consumers in Europe may ingest up to 11 000 MP year-1 (Van Cauwenberghe and Janssen, 2014). In Peru, 2688 tons of A. atra were extracted for fresh human consumption in 2017 (PRODUCE, 2018). The Peruvian population in 2017 was 31.24 million people (Instituto Nacional de Estadística e Informática [INEI], 2018), and considering the overall results in the present study (0.56 ± 0.08 MP g-1), the Peruvian population could be ingesting ~48.18 MP person-1 year-1 on average just from A. atra consumption. However, this is a rough estimation, as people living in coastal regions may be ingesting higher amounts of shellfish due to their proximity to port areas. The potential threat microplastic contamination could cause to food security has been a major concern in recent years (Barboza et al., 2018; Hantoro et al., 2019), although its direct effects on human health are still unclear. It is necessary to continue monitoring microplastic pollution in marine species captured for consumption, with a special focus on those eaten as a whole and sold in fishery markets ready for consumption. Characterization studies focusing on food items and foodstuffs must be accompanied by dietary intake estimations, expressed in consumed microplastics per person per year in order to elucidate the microplastic exposure derived from food ingestion. We hypothesize that a major source of microplastic contamination in shellfish and seafood is associated with the handling and supply chain. Future research must focus on determining pollution hotspots across the entire supply chain, from extraction or cultivation to consumption.
CONCLUSIONS
The consumption of shellfish and seafood in general as a pathway for microplastics to enter the human body is a major concern as many other environmental contaminants could be associated. Species that are eaten as a whole, like A. atra, present a higher risk of microplastic ingestion. In the present study, we surveyed the commercially popular A. atra from fishery markets in three Peruvian coastal provinces and reported the abundance, morphological characteristics, and polymer identity of the larger fraction of the microplastics in the soft tissues. Our results indicate that A. atra consumption in Peru may be a significant pathway for microplastic ingestion. In addition, a rough estimation of the annual dietary intake of microplastics in the Peruvian population via A. atra consumption was reported, although it is necessary to obtain data from a larger number of consumed organisms and food products to better understand microplastic intake through consumption. Microplastics have been evidenced in seafood around the globe, however, little is known about their effects. There is a need for further market surveys covering a broader range of commercially important shellfish species and contributing to the dietary microplastic intake of the Peruvian population.