Services on Demand
Journal
Article
Indicators
-
Cited by SciELO
-
Access statistics
Related links
-
Cited by Google
-
Similars in SciELO
-
Similars in Google
Share
Ingeniería e Investigación
Print version ISSN 0120-5609
Ing. Investig. vol.34 no.3 Bogotá Set./Dec. 2014
https://doi.org/10.15446/ing.investig.v34n3.42902
DOI: http://dx.doi.org/10.15446/ing.investig.v34n3.42902
J. J. Granada1, A. M. Cárdenas2 and N. Guerrero3
1Jhon James Granada Torres. Electronic Engineer and Magister in Telecommunications Engineering, Universidad Nacional de Colombia, Colombia. Affiliation: PhD Student, Universidad de Antioquia, Colombia. E-mail: jhon.granada@udea.edu.co
2Ana María Cárdenas Soto. Electronic Engineer, Universidad de Antioquia, Colombia. Ph.D in telecommunications, Universidad Politécnica de Valencia, España. Affiliation: Professor in Universidad de Antioquia, Colombia. E-mail: ana.cardenas@udea.edu.co
3Neil Guerrero González. Electronic Engineer and Magister in Industrial Automatization Universidad Nacional de Colombia. PhD in photonics, Denmark University of Denmark. Affiliation: Manager of High Speed Optical Networks in CPqD (Centro de Pesquisa e Desenvolvimento em Telecomunicações), Brazil. E-mail: neilg@cpqd.com.br
How to cite: Granada, J. J., Cardenas, A. M., & Guerrero, N. (2014). A Novel Dispersion Monitoring Technique in W-Band Radio-over-Fiber Signals using Clustering on Asynchronous Histograms. Ingeniería e Investigación, 34(3), 76-80.
ABSTRACT
Radio over Fiber (RoF) systems have been proposed as a promising solution for transmitting radiofrequency signals at high data rates over long distances. To reach data rates in the Gbps range, studies indicate using the W-Band (75 -110 GHz). However, in this frequency band, chromatic dispersion becomes an issue that increases the bit-error-rate. This paper presents a novel digital dispersion monitoring technique for RoF systems based on asynchronous histogram analysis. This method quantifies the intensity level of the distortion of a radiofrequency demodulated signal by a dispersion factor. This dispersion factor is calculated using an enhanced clustering approach, which carries out a Gaussian fitting technique through the expectation-maximization algorithm. Dispersion monitoring was performed on radiofrequency transmission simulations using non-return-to-zero and binary phase-shift keying modulated signals over 80 km of optical fiber at 60, 75 and 100 GHz. The bit error rate is estimated and compared to the dispersion factor, showing that the behavior of the dispersion effects are not proportional to the increase of carrier frequency, bit rate and distances. This novel monitoring method can be used to estimate the feasibility of RoF systems for future hybrid networks under specific transmission parameters such as fiber length, modulation format, and carrier frequency.
Keywords: Chromatic Dispersion, Optical Monitoring, Radio-over-Fiber.
RESUMEN
Los sistemas de Radio sobre Fibra (RoF), se proponen como una solución prometedora para transmitir señales de radiofrecuencia a altas tasas de transmisión y sobre largas distancias. Algunos estudios proponen el uso de frecuencias portadoras en la banda W (75 - 110 GHz), para lograr transmisiones en el rango de los Gbps. Sin embargo, en este rango de frecuencias la dispersión cromática, se convierte en uno de los principales factores para el incremento de la tasa de error del bit.
Este artículo presenta un nuevo método de monitoreo de la dispersión, basado en histogramas asíncronos para sistemas de RoF. El método planteado cuantifica el nivel de distorsión de la señal de radiofrecuencia, mediante un valor adimensional llamado: factor de dispersión. Dicho factor, se calcula mediante una técnica de agrupamiento, la cual se lleva a cabo usando ajustamiento gaussiano, mediante el algoritmo de máxima esperanza sobre histogramas asíncronos. El monitoreo de la dispersión se realiza sobre una plataforma de simulación, donde se transmiten las señales de radiofrecuencia, empleando modulación No retorno a cero (NRZ) y modulación binaria con desplazamiento de fase (BPSK), sobre 80 km de fibra óptica a 60, 75 y 100 GHz. Así, se estima la tasa de error de bit y se compara con el factor de dispersión; dónde se evidencia que los efectos de la dispersión no son proporcionales al incremento en la frecuencia de la portadora, la velocidad de transmisión y la distancia. El novedoso método de monitoreo, puede ser usado para estimar la viabilidad de los futuros sistemas de telecomunicaciones híbridos, basados en redes de fibra y bajo ciertos parámetros de transmisión tales como: la distancia, el formato de modulación y la frecuencia de la portadora.
Palabras clave: dispersión cromática, monitoreo óptico y Radio sobre Fibra.
Received: April 1st 2014 Accepted: September 3rd 2014
Introduction
The convergence of wired and wireless services suggests designing and implementing hybrid optical telecommunication platforms that allow signal transmissions of radio frequency (RF) carriers from central offices (CO) to base stations (BS) through optical fiber. Radio over Fiber (RoF) systems have been proposed as a solution to transmit RF signals at high data rates over long distances, centralizing equipment at COs, thus overcoming free-space attenuation and reducing system complexity at BSs (Taniguchi, et al, 2009). Important efforts in the standardization of research and development for the migration to millimeter wave (mmw) frequencies are in progress (Beas, et al, 2013). In RoF technology, different studies have been reported including full-duplex 60-GHz RoF system architecture that has enabled converged wireless services including multi-gigabit wireless data access over both 50-km SSMF (Hsueh, et al, 2008) and a WDM-RoF system based on remote up-conversion over 125 km (Hsueh, et al, 2012). RoF W-Band (range of 75 - 110 GHz) transmissions work on the availability of higher bandwidths for signal transmission in the Gbps range by avoiding the RF saturation of licensed bands (Stöhr, et al, 2009).
However, the intensity distortion of RF carriers and cyclic optical power variations become more critical for W-band RoF transmission due to optical fiber impairments such as chromatic dispersion (CD) and polarization mode dispersion (PMD) (Avo, et al, 2010), (Hilt, et al, 1998).
Estimation and compensation of CD and PMD have been extensively studied for the optical baseband of 40G and 100G coherent systems (Wang, et al, 2013). Several compensation methods using digital equalizations based on extracted information from asynchronous histograms (Minglun, et al, 2009), (Kozicki, et al, 2008) and digital signal processing in coherent optical systems (Faruk, et al, 2010) have been demonstrated and experimentally validated.
In this paper, we numerically show the CD effects on RoF transmission simulations using Non-Return-to-Zero (NRZ) and Binary-Phase-Shift-Keying (BPSK) modulated signals over 80 km at 60, 75 and 100 GHz. Demodulated signals present undesirable intensity levels increasing the bit error rate. Eye diagrams are obtained in a signal analyzer and a frame of the received signal is stored to calculate asynchronous histograms (AH). AHs are used as statistical tools to recognize undesirable patterns on the expected signal shape. A novel dispersion monitoring method is proposed, giving a dimensionless dispersion factor (DF) to quantify the distortion in the received signals. The DF is calculated using the Gaussian fitting technique, grouping the points of the AH and creating different clusters. The paper is divided into the following sections: RoF Systems Description, simulation framework, Chromatic Dispersion Effects over RoF Signals, Simulation Setup, Dispersion Monitoring Technique Description, Results and Conclusions.
Radio over Fiber Systems Descriptions
The convergence of wireless communications and optical fiber systems have become a promising technique to provide services for broadband wireless access in a range of applications including accessing network solutions, expanding coverage and radio network capacities. In this sense, Radio-over-Fiber (RoF) systems provide adequate synergy between optical and wireless communications, allowing for the fusion of these technologies, which have been essential in the development of telecommunications. It is expected that the next generation access networks will ensure the provision of broadband services and multimedia applications to end users at any time and any place, trying to achieve fixed network data rates in wireless communication (Granada, et al, 2011).
RoF systems are telecommunication platforms, where radiofrequency (RF) signals are optically modulated in a central office (CO) and transmitted into the fiber to different base stations (see figure 1). RoF systems transmit RF modulated signals over longer distance than wireless telecommunication systems due to the lower signal power attenuation of optical fiber in comparison to air. One of the most important advantages of RoF technology is the ability to centralize expensive high-frequency RF equipment at the CO. BSs are used only for the optical-electrical conversion, allowing for easy installation and operation. Therefore, generating high-frequencies in the BS is avoided (Milosavljevic, et al, 2009). Additionally, BSs are small and have low power consumption (Taniguchi, et al, 2009).
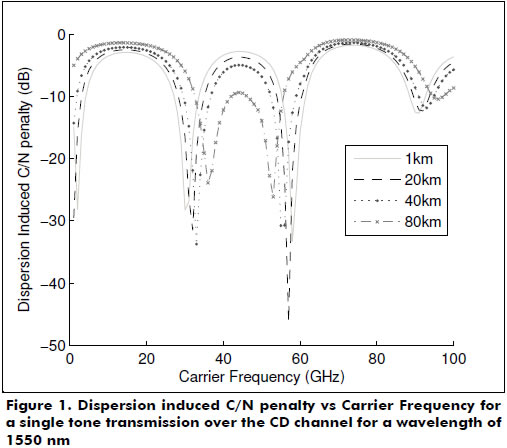
The high bandwidth of the optical fiber is efficiently exploited when signals are transmitted in the millimeter-wave band. However, when the transmitted signal is radiated through free space, it is attenuated, reaching only short distances. Therefore, mmw band systems are being considered for applications within buildings and indoors, using micro- and picocells. In this frequency band, optical transmission impairments, such as chromatic dispersion, more drastically affect the transmitted signal.
Chromatic Dispersion Effects over RoF Signals
Optical fiber impairments affect the transmitted information signal to a greater or lesser extent depending on the type of fiber, transmission distance, modulation formats and detection techniques used. One of the most influential impairments is the chromatic dispersion (CD). CD has been widely studied in optical baseband system and successfully compensated using several electrical and optical techniques. CD was not considered an important degradation factor in the first RoF systems due to their low data rates and carrier frequencies lower than 10 GHz ( Mitchell, 2009). However, the next generation of RoF systems are foreseen as high-capacity systems (above Gbps), reaching distances greater than a km in the W-Band (75 - 110 GHz) carrier frequency. For W-band RoF systems, intensity distortions of RF carriers and cyclic optical power variations due to CD become more critical.
CD is a linear effect inherent of the optical fiber, which results from differences in the propagation time (delays) of the different spectral components of the transmitted signal. CD is caused by the interaction of 2 dispersions effects: i) material dispersion and ii) waveguide dispersion (Agrawal, 1997). CD in Optical fiber can be modeled as a bandpass filter (Gliese, et al, 1996) as follows:

where z is the distance of propagation, D is the dispersion parameter of the fiber defined by the manufacturer, f is the offset frequency of the optical carrier, and c is the speed of light. The optical signal at the output of the fiber, Yout(f), is given by

where Yin(f) is the Fourier transform of the input signal (unmodulated) in the optical channel, Yin(ft), defined as

where fopt is the frequency of the optical carrier and fc is the radiofrequency carrier. The output signal in the time domain can be observed in equation (4), where its power intensity has a cyclical behavior depending on the systems parameters:

After detection, the transmitted signal has a power loss that depends on the different transmission parameters and mainly from the fc. From equation (4), we can derive that the transmitted signal power has a cyclical behavior due to sinusoidal functions. It can be shown in a carrier to noise ratio (C/N) plot as in figure 2, where C/N is induced by CD. A single carrier is transmitted without modulation through the channel modeled by equation (1). It is observed that the C/N penalty changes for each value of the carrier frequency in a non-logarithmic way for transmission distances of 1, 20, 40 and 80 km. Hence, the C/N and carrier frequency relation is not proportional to the transmission distance. For example, for fc = 20 GHz the lower C/N penalty is reached for 80 km and the higher C/N penalty is reached for 1 km, but for fc = 50 GHz, the opposite case holds.
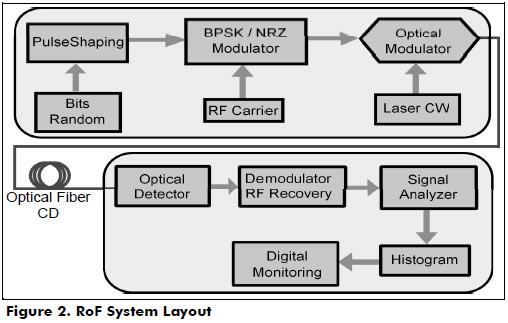
In RoF, the power penalty effects due to CD cannot be characterized as in figure 2 because each of the transmission parameters, including the modulation format, changes the transmitted signal drastically. Furthermore, the CD effects with other physical impairments, such as polarization mode dispersion, attenuation, scattering, non-linear effects, among others, can deteriorate the signal to a greater extent or even improve it due to the cancellation of some effects (Berceli, et al, 2008).
Figures 3.c and 4.a show an eye diagram of a 250 Mbps RoF transmission for 40 and 80 km, respectively. In spite of the eye-opening and a low power loss, the rising and falling edge in figure 3.c has a large slope, indicating a sensitivity to timing error. In figure 4.a, the signal distortion is represented by an appreciable jitter. In the eye diagram of figure 4.c the eye diagram is observed to have several amplitude power levels. In this case, a 1 Gbps RoF transmission at 40 km, the information cannot be recovered.
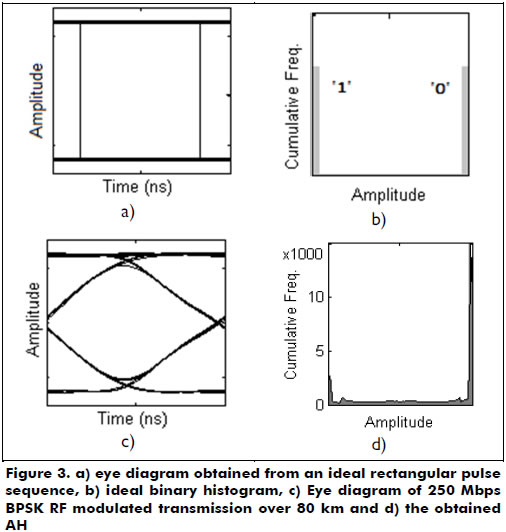
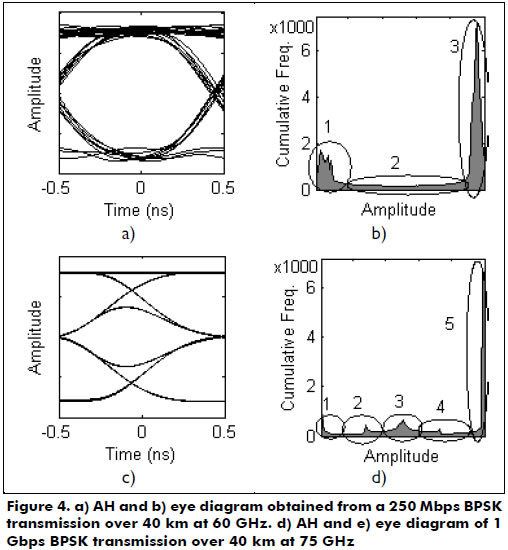
As we have mentioned and explained in (Maeda, et al, 2014), the received RF power level suffers from periodic power fading caused by cyclical changes in the relative phase between the lower sideband and the upper side band in the optical domain. This phenomenon is called dispersion-induced fading frequency. Optical single side band modulation using optical filtering has been proven to alleviate the fading effect. However, it also causes additional signal distortion (Chen, et al, 2013). In addition, the dispersion-induced phase noise increases with greater dispersion (different types of fibers) and wider laser linewidth, which was evaluated in (Wei, et al, 2014) for W-band transmission. Thus, monitoring dispersion is needed in W-Band RoF systems.
Simulation Setup
Radio-over-Fiber (RoF) transmission simulations, with Non-return-to-zero (NRZ) on-off keying and Binary-phase-shift-keying (BPSK) modulation, evaluate the impact of the Chromatic dispersion (CD) effects in the demodulated signals. The simulation setup (figure 2) is carried out in the Virtual Photonics Inc (VPI) software.
The transmitter consists of an optical source of continuous waves, with a linewidth of 2 MHz in the 1550 nm range. The output of the laser is connected to an optical external intensity modulator with ideal characteristics. The bit sequences are digitally modulated in an electrical format, and NRZ and BPSK have carrier frequencies of 60, 75 and 100 GHz. The optical power of the laser is 1 mW. The optical fiber model used is a standard single mode fiber (SSMF) with a length of 40 and 80 km. At the receiver, transmitted signals are detected with a photodiode that has a responsivity of 1 A/W. Electrical NRZ signal demodulation is performed using an envelope-detector Gaussian filter and electrical BPSK signal demodulation is carried out using a local oscillator which operates at the same frequency as the RF transmitter signal.
The recovered electrical signal is analyzed in the temporal domain to build asynchronous histograms, and a digital dispersion monitoring technique is performed from this.
Dispersion Monitoring Technique Description
The proposed digital dispersion monitoring technique is explained as follows:
i) Asynchronous histograms (AH) are generated by projecting the eye diagram of the electrical demodulated signal on its vertical axis and normalizing its amplitude. Figure 3a shows an eye diagram obtained from an ideal rectangular pulse sequence, the AH obtained from this eye diagram is shown in the figure 3.b. Cumulative frequencies represent the number of times that a specific amplitude value is repeated in the time domain signal. Intensity distortion due to the chromatic dispersion (CD) effects are seen as variations in the cumulative frequencies in the AH. For example, figure 3d shows an AH obtained from the eye diagram (figure 3c) of a RoF BPSK 250 Mbps transmission over 40 km at 75 GHz.
ii) The points of an AH are grouped in different clusters. The number of peaks and valleys in the AH are calculated. Each "peak" in the AH, means an intensity level in the time domain signal. The number of clusters (n) is defined as the number of peaks. However, if there is a valley in the x-axis center of the AH (figure 4.b), the number of clusters (n) is equal to the number of peaks plus one (figure 4.d).
iii) A Gaussian fitting technique using an expectation-maximization (EM) algorithm (Alder, 2001) is performed to calculate the number of points belonging to each cluster. The data inputs in the algorithm are n, points of an AH, and number of iterations.
iv) According to the total number of points in the AH, the statistical mean value (µ) for each cluster and the points proportionally (P) belonging to each cluster are calculated, as follows:

v) With n, µ, and P, a dimensionless dispersion factor (DF) is proposed in equation 6, the numerator is associated with the 2 clusters that represent the '1' and the '0' logic in the demodulated signal, and the denominator represents the cluster or the clusters located in unwanted regions of the AH. Therefore, a low-dispersion demodulated signal will have higher DF values than a high-dispersion demodulated signal.
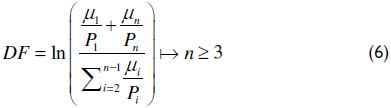
Results
Tables 1 and 2 show the dispersion factors (DF) and the bit error rate (BER) calculated for different RoF transmissions. Because the EM algorithm can converge with approximate values, DF was averaged after 5 runs. BER is calculated in the VPI simulator using a probabilistic estimation with a Gaussian distribution.
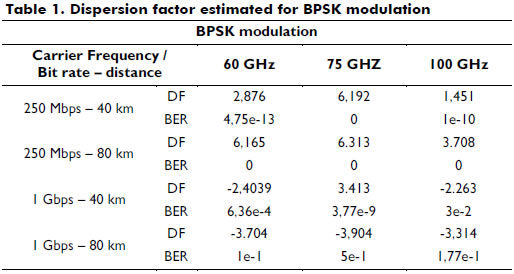
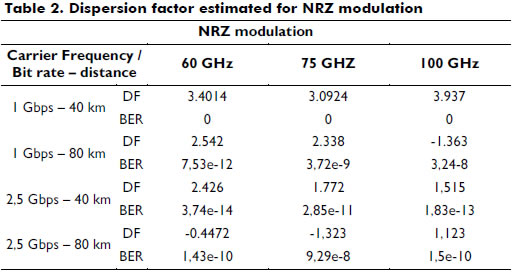
According to table 1 and 2, in most cases, NRZ shows better performance with higher DF values and lower BER values than the BPSK case. It is observed, comparing the results of the NRZ case for the 1 Gbps transmission over 80 km, that the DF values are between -1.363 and 2.542 for different carriers, whereas in the BPSK case, the DF values are between -3.314 and -3.904. Nevertheless, with a 75 GHz carrier frequency, the BPSK case shows better performance than the NRZ case, with a DF value of 3.413 compared to a DF of 3.094 in the NRZ case. Otherwise, making a comparison among the 3 different carrier frequencies (60, 75 and 100 GHz), the DF and BER values are not proportional as the carrier frequency increase. For example, in the BPSK case, a 250 Mbps transmission over 40 km, shows better DF and BER values with a 75 GHz carrier than with 60 GHz and 100 GHz carriers. In a 250 Mbps BPSK transmission, a better transmission performance is obtained over 80 km than over 40 km. However, at 1 Gbps a better performance was obtained over 40 km than over 80 km.
Dispersion effects are not proportional with the increase of carrier frequency, distances and bit rate because CD effects are related to the power penalty, which was explained in section III, where a non-logarithmic curve represented the carrier variation according to the distance (figure 2). Hence, BER has an inverse relation with regard to DF: when BER increases, DF values decrease. However, in some cases, transmissions have similar DF values but different BER values because CD is not the only parameter that increases physical impairments and other noises affect the transmission systems. Tables 1 and 2 prove that small variations in the parameters of RoF systems can significantly change the received signal quality.
Conclusions
In this paper, we proposed a novel dispersion monitoring method using asynchronous histograms. The EM algorithm performs Gaussian distribution fittings, and it estimates the statistical mean and the cardinality of each cluster generated in histograms and from these values, equation (6) determines the dispersion factor of the demodulated signals. DF values showed that small variations in the parameters of RoF systems such as fiber length, carrier frequency and data rate can significantly change the quality of the received signal. For future network scenarios, this method can be used to estimate the feasibility of implementing RoF systems that work with certain optical network conditions.
References
Agrawal, G. (1997). Fiber-Optic Communication Systems. John Wiley and Sons, Inc. [ Links ]
Alder, M. (2001). An introduction to pattern recognition: Statistical, Neural Net and Syntactic methods of getting robots to see and hear. ©Mike Alder. [ Links ]
Avo, R., Laurencio, P., & Medeiros, M. (2010). 60 GHz radio-over-fiber transmission impairments for broadband wireless signals. In Transparent Optical Networks (ICTON), 12th International Conference, (pp. 1-4). [ Links ]
Beas, J., Castanon, G., Aldaya, I., Aragon-Zavala, A., & Campuzano, G. (2013). Millimeter-Wave Frequency Radio over Fiber Systems: A Survey. Communications Surveys & Tutorials, (99), 1-27. [ Links ]
Berceli, T., Udvary, E., & Hilt, A. (2008). A new equalization method for dispersion effects in optical links. In 10th Anniversary International Conference Transparent Optical Networks (ICTON) (Vol. 4) (pp. 98-101). [ Links ]
Chen, D., Sun, C., & Yu, P. (2013). Origin of high linearity in radio-over-fiber link with cascaded filter. Avionics, Fiber-Optics and Photonics Conference (AVFOP) (pp. 53-54), IEEE. [ Links ]
Faruk, M., Mori, Y., Zhang, C., & Kikuchi, K. (2010). Multi-impairments monitoring from the equalizer in a digital coherent optical receiver. In Optical Communication (ECOC), 36th European Conference and Exhibition (pp. 1-3). [ Links ]
Gliese, U., Norskov, S., & Nielsen, T. (1996). Chromatic dispersion in fiber-optic microwave and millimeter-wave links. Microwave Theory and Techniques, IEEE Transactions, 44(10), 1716-1724. [ Links ]
Granada, J. J., Serpa, C., Varón, G. M., & Guerrero, N. (2011). Hacia la próxima generación de sistemas de Radio sobre Fibra de banda ancha: retos tecnológicos en la banda de las ondas milimétricas. Ingeniería y Desarrollo, 29(2). [ Links ]
Hilt, A., Vilcot, A., Berceli, T., Marozsak, T., & Cabon, B. (1998). New carrier generation approach for fiber-radio systems to overcome. In Microwave Symposium Digest (Vol. 3) (pp. 1525-1528). IEEE MTT-S International. [ Links ]
Hsueh, Y.-T., Cheng, L., Shu-Hao, F., Yu, J., & Chang, G.-K. (2012). A novel full-duplex testbed demonstration of converged all-band 60-GHz radio-over-fiber access architecture. In Optical Fiber Communication Conference and Exposition (OFC/NFOEC) and the National Fiber Optic Engineers Conference (pp. 1-3). [ Links ]
Hsueh, Y.-T., Chien, H.-C., Jia, Z., Chowdhury, A., Yu, J., & Chang, G.-K. (2008). 8x2.5Gb/s, 60-GHz Radio-over-Fiber acces network with 125-km extended reach using remote optical carrier suppression (pp. 798-799). IEEE Lasers and Electro-Optics Society. [ Links ]
Kozicki, B., Takuya, O., & Hidehiko, T. (2008). Optical Performance Monitoring of Phase-Modulated Signals Using Asynchronous Amplitude Histogram Analysis. Lightwave Technology, 26(10), 1353-1361. [ Links ]
Maeda, J., Kimura, K., & Ebisawa, S. (2014). Experimental Study on Variation of Signal Amplitude in Radio-Over-Fiber Transmission Induced by Harmonics of Modulation Sidebands Through Fiber Dispersion. Lightwave Technology, 32(20), 3536-3544. [ Links ]
Milosavljevic, M., Kourtessis, P., Gliwan, A., & Senior, J. (2009). Advanced PON topologies with wireless connectivity. In 11th International Conference Transparent Optical Networks (ICTON) (pp. 1-4). [ Links ]
Minglun, Z., Yangan, Z., Xueguang, Y., Jin-nan, Z., & Xiaoguang, Z. (2009). Analyses of amplitude histogram methods used for optical performance monitoring. In Communications and Signal Processing, ICICS, 7th International Conference (pp. 1-3). [ Links ]
Mitchell, J. (2009). Radio over fibre networks: Advances and challenges. In 35th European Conference Optical Communication (ECOC) (pp. 1-4). [ Links ]
Stöhr, A. (2009). 60 GHz radio-over-fiber technologies for broadband wireless services. Optical Networking, 8(5), 471-487. [ Links ]
Taniguchi, T., Sakurai, N., Kimura, H., & Kumozaki, K. (2009). Technical trends in millimeter-wave band Radio-on-Fiber acces system (pp. 24-27). Beijing: PIERS Proceedings. [ Links ]
Wang, D., Lu, C., Lau, A., Wai, P., & He, S. (2013). Adaptive CD Estimation for Coherent Optical Receivers Based. Photonics Technology Letters IEEE, 25(10), 985-988. [ Links ]
Wei, C., Lin, C., Huang, H., Liang, W., & Chi, S. (2014). Estimation and Suppression of Dispersion-Induced Phase Noise in W-band Direct-Detection OFDM Radio-Over-Fiber Systems. Lightwave Technology, 32(20), 3874-3884. [ Links ]