Introduction
Diesel engines are widely used due to their various advantages compared to gasoline engines. The highly efficient, durable, and reliable nature of diesel engines makes them more attractive and preferable (Kumar et al., 2020). Miscellaneous sectors such as transportation, agriculture, and power generation need this kind of engine due to its superior features.
Diesel engines have been conventionally powered by fossil-based fuels since their invention, although there have been some attempts for alternative fuel utilization. Unfortunately, fossil fuels have been faced with the scarcity of reality since the late 1970s and early 1980s (Tüccar et al., 2014). Therefore, efforts to decrease the dependency on fossil fuels are currently being made by scientists. Alcohols are one of the most impressive alternatives, as they can be directly used as an additive in engines. The production of alcohols is biologically achieved via fermentation, which is a cost-effective and environmentally friendly method (Yesilyurt, 2020). On the other hand, no engine modification is needed while using alcohols as a primary fuel or fuel additive (Çelebi and Aydin, 2019).
Methanol (CH3OH) and ethanol (C2H5OH) are regarded as lower alcohols, and they have been widely studied in engine experiments by researchers. The extra oxygen content in their structure and their enhanced soot oxidation due to the hydroxyl group (which leads to lower levels of opacity, especially at higher engine loads) can be listed among the prominent advantages of methanol and ethanol (Nour et al., 2017, 2019). Despite this common use, these alcohols have some disadvantages, such as their low heating value and high evaporation enthalpy, which causes a weak engine performance, increased carbon monoxide and hydrocarbon emissions, high auto-ignition resistance, long ignition delay periods, high vapor pressure, a low flash point (important for storage), poor lubrication, and weak miscibility with diesel fuel (Nour et al., 2019).
Besides lower alcohols, another group that has recently attracted attention is higher alcohols. The term higher alcohol indicates series of straight-chain alcohols that contain more than three carbon atoms, such as butanol (C=4), pentanol (C=5), hexanol (C=6), octanol (C=8), dodecanol (C=12), phytol (C=20), etc. (No, 2020).
Lately, there has been great interest in higher alcohols due to their from enhanced ignition quality, which can be attributed to the increased carbon chain lengths in their molecular structure (Koivisto et al., 2015). Other featured properties of higher alcohols include a high energy density, an increased cetane number, a better miscibility, and a lower hygroscopic tendency (Rajesh Kumar and Saravanan, 2016).
In recent years, alcohols, especially higher ones, have begun to replace conventional fuels, as their usability in internal combustion engines has become increasingly remarkable. Therefore, research has recently focused on this subject.
Pan et al. (2020) studied the effects of butanol isomers on diesel engine performance, emissions, and combustion behaviors under varying load conditions. They concluded that cylinder pressure and the heat release rate increased with diesel/butanol blends when compared to diesel fuel. As for emissions, butanol helps to reduce soot emissions, but it causes an increase in nitrogen oxides and carbon monoxide emissions at low loads. At high loads, butanol reduces particulate matter emissions.
Singh et al. (2020) used N-butanol as an additive to a eucalyptus biodiesel/diesel mixture in order to observe its effects on engine performance and emissions. They found that the brake power values of all fuel samples were nearly the same. When the butanol concentration was increased in the blends, lower brake specific fuel consumption and higher brake thermal efficiency values were obtained. On the other hand, butanol generated reduced levels of nitrogen oxides and increased levels of carbon dioxide with respect to pure diesel.
Pan et al. (2019) experimentally evaluated the spray and combustion characteristics of a compression ignition engine operated with n-pentanol/diesel blends. Results demonstrated that n-pentanol/diesel blends help to obtain better atomization characteristics than diesel fuel. Although the addition of n-pentanol to diesel fuel decreased soot emissions, increments in brake-specific fuel consumption and hydrocarbon and nitrogen oxide emissions were observed.
Radheshyam et al. (2020) studied the effects of adding 1-pentanol to diesel, as well as EGR (exhaust gas recirculation) on a common rail direct injection diesel engine. They observed that the engine can be operated with up to 30% 1-pentanol-mixed fuel with no need to modify the engine. Increasing the 1-pentanol percentage in mixtures yields a higher brake-specific fuel consumption and a lower thermal efficiency. Nitrogen oxide emissions decreased in all blends with 1-pentanol, while hydrocarbon and carbon monoxide values increased.
Thomas et al. (2020) sought to determine the effects of hexanol and biodiesel produced from waste vegetable oil on the combustion of a reactivity-controlled compression ignition engine. Their experiments revealed that nitrogen oxides and smoke emissions were reduced with biodiesel/ hexanol blends when compared to diesel. They finally suggested that biodiesel/hexanol mixtures should replace fossil fuels as an effective solution for reactivity-controlled compression ignition.
Ramesh et al. (2019) conducted an experimental study to understand the effects of Calophyllum inophyllum biodiesel and hexanol on diesel engine applications. Diesel/ biodiesel/n-hexanol blends were prepared. Hexanol in blends caused an increase in brake thermal efficiency and a decrease in specific fuel consumption in comparison with pure biodiesel. While hydrocarbon, smoke, and carbon monoxide emissions were reduced, those of nitrogen oxides and carbon dioxide increased when compared to diesel.
Aneeque et al. (2021) studied the effects of alcohols and Calophyllum inophyllum biodiesel while employing a response surface optimization methodology. They evaluated the impacts of alcohol (N-octanol, N-butanol) and biodiesel on the performance and emissions of a diesel engine, and they performed a response surface analysis to obtain the optimal engine efficiency values and emission profiles. The researchers finally suggested that hybrid blends of alcohol and biodiesel can be used directly in diesel engines with no need for engine modification.
Khan et al. (2020) evaluated the impacts of synthesized waste milk scum oil methyl ester and ethanol blends on diesel engine performance characteristics. At different ratios, binary blends (20% biodiesel + 80% diesel, 60% biodiesel + 40% diesel, 100% biodiesel) and ternary blends (20% biodiesel + 5% ethanol + 75% diesel, 20% biodiesel + 10% ethanol + 70% diesel, 20% biodiesel + 15% ethanol + 65% diesel) were prepared. The physicochemical properties of the blends were evaluated, and performance tests were conducted on a single-cylinder diesel engine. The authors concluded that the 20% biodiesel + 5% ethanol + 75% diesel ratio yields the best values regarding fuel consumption, the air-fuel ratio, and thermal efficiency.
Although many experimental studies on compression ignition engines operated with lower alcohols (methanol, ethanol) have been published, the combustion characteristics of higher alcohols still require further research. As stated before, higher alcohols have been intensively studied by scientists in recent times. Therefore, this study focused on determining the performance and emission characteristics of a diesel engine fed with diesel fuel and ternary higher alcohol additives (butanol, pentanol, and hexanol).
Methodology
Engine tests were performed at the Automotive Engineering Laboratories of Çukurova University. A naturally aspirated, 4-stroke, 4-cylinder compression ignition engine was employed for conducting performance and emissions tests at full load. The technical specifications of the engine are presented in Table 1.
Figure 1 presents a schematic of the engine test rig. The engine test set-up was basically composed of a diesel engine, a hydraulic dynamometer, performance and emissions measurement devices, and a control unit.
Diesel fuel and alcohols (butanol, pentanol, and hexanol) were purchased from a commercial firm in Adana, Turkey. Three different fuels were used in this study. The fuels employed were diesel fuel and diesel plus ternary alcohol mixtures with different ratios. Table 2 summarizes the fuels used in the experiments.
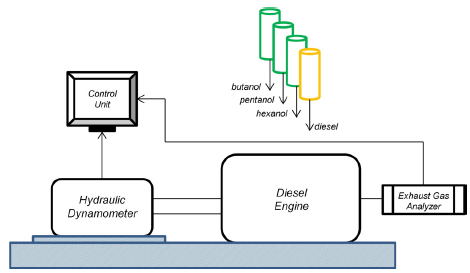
Source: Author
The mixing of diesel and alcohols was achieved with an ultrasonic processor (Sonic Vibra-Cell VC 750 model) in order to homogenize and stabilize the blends before using them in the engine.
An IKA-Werke C2000 Calorimeter, a Zeltex ZX 440 NIR petroleum analyzer, a Kyoto Electronics DA-130 density meter, a Tanaka AKV-202 automatic kinematics viscosity meter, a Tanaka MPC-102L pour point analyzer, and a Tanaka APM-7 flash point analyzer were used to determine the heating value, cetane number, density, viscosity, and flash point, respectively. Table 3 shows the physicochemical fuel properties of the D, DA5, and DA10 fuels. The measured properties were also compared to EN 590 standards.
The evaluation of fuel physicochemical properties is a crucial priority in interpreting the combustion, performance, and emission characteristics of an engine.
Results
Brake power (BP)
Figure 2 depicts the variations in BP with the engine speed. Diesel fuel showed the best performance. In comparison with reference diesel fuel, the average decrements in BP were 13,06 and 24,25% for DA5 and DA10, respectively. Increased alcohol concentrations in the mixtures caused further decrements in BP levels. As seen in Table 3, the lower calorific values of alcohols and blending them with diesel fuel led to a slight decrement in the heating values of the mixtures. The reduced BP values could be explained by the lower energy content of the mixtures (Appavu et al., 2020; Karabektas and Hosoz, 2009; Sundar Raj and Saravanan, 2011).
Brake-specific fuel consumption (BSFC)
The consumed mass of fuel per unit time can be expressed as the BSFC in order to obtain the unit power. This important parameter is directly related to the engine's fuel economy. Figure 3 shows that there is an increment in BSFC with the diesel-alcohol blending procedure when compared to diesel fuel. On average, 7,98 and 14,57% increases were obtained for DA5 and DA10 in comparison with diesel. One of the possible causes for the increased BSFC might be the lower calorific values of alcohols. Another reason could be the higher latent heat of evaporation, which contributes to extracting more heat from the combustion chamber. This cooling effect in the cylinder can reduce the combustion efficiency (Karabektas and Hosoz, 2009; Sundar Raj and Saravanan, 2011; Yilmaz et al., 2017).
Carbon monoxide (CO)
CO appears in engines as an incomplete combustion product, and it is one of the most hazardous and toxic gases released after combustion. As seen in Figure 4, alcohol utilization in the mixtures enhanced the CO emissions characteristics of the tested engine. Compared to diesel, on average, CO decreased by 8,07 and 12,87% for DA5 and DA10, respectively. The oxygen content in the molecular structure of alcohols may trigger a further oxidization of CO molecules. Therefore, a more complete combustion can be achieved, which leads to reduced CO levels (Devarajan et al., 2017; Kattela et al., 2019; Ramesh et al., 2019).
Nitrogen oxides (NO x )
The burning of fuel occurs in the presence of oxygen. Air contains not only oxygen, but also nitrogen and a small amount of other gases. Moreover, approximately 79% of the air consists of nitrogen. At elevated temperatures in the combustion chamber (~ above 1 600 °C), nitrogen reacts with oxygen to form nitrogen oxides. Therefore, temperature is an important factor in NOx formation. Figure 5 shows a decrement in NOx emissions with diesel-alcohol blends when compared to diesel fuel. On average, 8,73 and 14,92% drops were obtained for DA5 and DA10, respectively. These drops may be associated with the high latent heat of evaporation values of the alcohols in the blends. A higher latent heat of evaporation means a higher heat removal from the combustion chamber, which has a cooling effect. This phenomenon has been validated by many researchers in their studies (De Poures et al., 2017; Jeyakumar and Narayanasamy, 2019; Joy et al., 2020).
Conclusions
In this study, the performance and emission characteristics of a diesel engine operated with ternary higher alcohol biofuel blends were analyzed.
From this experimental evaluation, the following conclusions can be drawn:
The fuel properties of mixtures seem to be suitable for direct use in engines.
Blending alcohol with fossil fuels contributes to reducing the dependence on conventional fuels, which are becoming scarce.
Although there was a decreasing trend in performance parameters with alcohol blends, the emission values were improved. If less dependence on conventional fuels is desired and environmental concerns are a priority, using these fuels is recommended.
The increased alcohol ratio in the blends led to a weaker engine performance, albeit with reduced levels of CO and NO emissions.
The worst BP (due to reduced energy contents) and BSFC values (due to both the lower energy content and the higher latent heat of evaporation of alcohols) were obtained with a 24,25% reduction and a 14,57% increment for DA10 compared to diesel fuel. In addition, the best emission reductions were obtained with DA10 fuel, i.e., 12,87 and 14,92% decrements for CO and NOx, respectively. A decrease in CO emissions may be obtained by supplying more oxygen to the cylinder with alcohols. On the other hand, the lower NOx levels can be explained by the higher latent heat of evaporation of alcohol, which lowers the cylinder's internal temperature.