INTRODUCTION
Banana is one of the most important crops around the world, it is a staple in the diet of millions of people in addition to contributing significantly to the export income of less developed countries around the world (FAO 2011, 2014). However, banana production is affected by different pathogens; one of the most persistent is the Ascomycete fungus Mycosphaerella fijiensis M. Morelet, cause of the Black Sigatoka disease (Churchill 2011, Marín et al. 2003). The cost of chemical control of this disease and its impact on non-target species has led to the search for new molecules and strategies that are more specific and sustainable (Arzate et al. 2006, Ayala et al. 2014, Ceballos et al. 2012, EPA 2005, Martinez et al. 2005, Niño et al. 2011, Osorio 2006, Viveros and Castaño 2006). Antifungal proteins appear as potential control agents due to their substrate specificity (i.e chitin, peptides, glucans) and diversity that allows them to cleave or modify different cellular components of an organism (i.e phytopathogens) (Fernández et al. 2011, Hegedüs and Marx 2013, Ngai et al. 2005, Wong et al. 2010). In this study we have focused on the mushroom Ganoderma lucidum (Curtis) P. Karst., a lignolytic fungus traditionally known for its medicinal properties (Ansor et al. 2013, Manavalan et al. 2015, Paterson 2006, Suarez et al. 2013). We report the inhibitory effect of G. lucidum protein extracts over M. fijiensis growth and also determined its enzymatic DNase, RNase, glucanase, protease and chitinase activities, and the presence of potential antifungal proteins in order to elucidate the possible mechanism responsible for the growth inhibitory activity.
MATERIALS AND METHODS
Organisms and growth conditions
Ganoderma lucidum (Curtis) P. Karst cultivation. A G. lucidum (Curtis) P. Karst strain, donated by Dr. Shu-Ting Chang from the University of Hong Kong to the Biotechnology Laboratory of the Universidad de Antioquia, was used in this study. The culture was preserved on FC1 agar (Sacarose, yeast extract, barley flour and agar).
The biomass used for protein extraction was obtained from Bioreactor cultures, which were prepared by inoculation of 4.5 L of culture medium reported by (Zapata et al. 2009). in a seven liter BIOFLO 110® Bioreactor by 0.1% w/v of pre-inoculum and incubated at a temperature of 25 °C ± 1 °C under constant agitation at 150 rpm and blue light over 5 days (Zapata et al. 2009).
Mycosphaerella fijiensis M. Morelet cultivation and identification. Three M. fijiensis isolates were obtained from infected banana leaves from the Urabá region in Colombia provided by The Colombian Banana Growers Association (AUGURA), through the isolation of monosporic cultures that were incubated in PDA at 25 °C in the darkness for over 30 days before their subcultivation. The isolates were identified using the Internal transcribed spacer regions 1/2 (ITS) (Johanson and Jeger 1993) and actin (ACT) primers. The sequences were aligned in BlastN and the analysis was made using the software MEGA 6.0.
Protein isolation from G. lucidum. The biomass was kept in plastic bags at -20 °C, for seven days. The bags were thawed, and the recovered biomass was washed with PBS pH 7.4, 1.5 mM and filtered through a membrane of 50 mm using a vacuum pump, and proteins were extracted using mechanic lysis following previous reports (Crowell et al. 2013, LaMontagne et al. 2016). The protein extracts were then incubated overnight at 4 °C before their use.
Three independent experiments were carried out and biological replicates were pooled to minimize biological variation. Protein content was measured using the Bradford method with bovine serum albumin BioRad® as the standard (Bradford 1976, Crowell et al. 2013).
Enzyme assays. All enzymatic assays were carried out using the extracts obtained from G. lucidum submerged cultures biomass. All assays were performed in triplicate and the mean values calculated. Spectrophotometric methods were used to determine glucanase (Jijakli and Lepoivre 1998, Liang et al. 1995), chitinase (Cretoiu et al. 2012), protease (Gabarro et al. 2016, Tian et al. 2004), deoxyribonuclease (Kunitz 1946) and ribonuclease (Kalnitsky et al. 1958, Kunitz 1946) activities.
Fractionation
Preparative isoelectric focusing and Polyacrylamide gel electrophoresis (SDS-PAGE). Isoelectric focusing (IEF) was performed on the mini Bio-Rad Rotofor preparative IEF system (Bio-Rad Laboratories Ltd., Hemel Hempstead, UK). After the completion of the preparative IEF run, the fractions were harvested and assayed for antifungal activity (Khalyfa et al. 1993, O’Loughlin et al. 2014), and sodium dodecyl sulfate (SDS)-PAGE was performed according to the method of Laemmli. The gels were stained with silver nitrate (Amresco®) (Chevalier 2010, Laemmli 1970).
Assay for fractions’ antifungal activity. The inhibition tests were performed based on the CLSI method M38-A2 for fungi susceptibility to antifungals (CLSI 2009).
Statistical analysis. A totally random design (TRD) was performed with twenty experimental units. Statistical analyses included parametric tests and ANOVA (Montgomery 2012) using GraphPad Prism 6 software.
Mass spectrometry identification
Trypsin digestion and LC-MS/MS. Gel plugs were washed twice using 500 µL of HPLC grade water and 500 µL of acetonitrile (ACN) 50/50 ACN/ 25 mM ammonium bicarbonate (AMBIC). The gel plugs were first dehydrated, reduced, vacuum dried and digested with trypsin. Lastly, the trypsin was removed, and the samples were washed with 25 mM AMBIC. Every tube was analyzed independently (Lapolla et al. 2004). The extracted peptides were analyzed in a MALDI-TOF-TOF Proteomics Analyzer. The mass spectra were processed and identified using MASCOT 2.0 (Dekroon et al. 2011).
RESULTS
Organisms and growth conditions
Ganoderma lucidum cultivation. For all the batches cultivated an average of 54.3 g/L of G. lucidum wet biomass was obtained per batch after 5 days of incubation under the determined conditions (Table 1).
Table 1 Average values of biomass concentration, protein concentration and enzymatic activities
Biomass (g/L) | Protein concentration (mgmL-1) | Protease (Abs 450) | Glucanase (µmol min/mL) | Chitinase (Chitobiosidase U/mL) | Chitinase (Glucosaminidase U/mL) | RNase activity (U/mg) | DNase activity (U/mg) |
---|---|---|---|---|---|---|---|
54,3 ± 1,97 | 2,5 ± 0,02 | 0,610 ± 0,011 | 6,52 ± 0,02 | 0,053± 0,002 | 0,063± 0,00001 | 760 ± 10 | 0,076 ± 0,018 |
Mycosphaerella fijiensis cultivation and identification. After thirty days of cultivation, colonies of five centimeters were obtained; the three isolates were satisfactorily identified as M. fijiensis.
Protein isolation from G. lucidum. The protocol allowed us to obtain approximately 2.5 mg/mL of soluble protein, starting with twenty grams of wet biomass; these extracts were used for the activity assays consistently.
Enzymatic assays. All of the enzymatic assays performed turned positive, indicating the presence of peptidases, β glucanases, chitinases (chitobiosidases and glucosaminidases), ribonucleases and deoxyribonucleases (Table 1).
Chitinase activity. For every extraction evaluated, the chitobiosidase test indicated that the G. lucidum extract showed an activity three times higher when compared to the control chitinase corresponding to 0.053 UmL-1, whereas for the glucosaminidase test the G. lucidum protein extract had half the activity of the control chitinase (0.063 UmL-1). However, on average the glucosaminidase and chitobiosidase activity values are close (0.05-0.06 U/mL) (Table 1).
Protease activity. All the extractions performed presented proteolytic activity under the conditions assayed, besides, under the concentrations tested (Table) the commercial trypsin showed an activity 2.6 times higher than the succinylated casein, which had an activity corresponding to an absorbance of 0.610 at 405 nm when a concentration of 600 ppm was used, as it is shown in Table 1.
Deoxyribonuclease activity. The results of the DNase activity assay revealed that 125 mg/mL (dilution 1:20 of the concentrated extract) of protein extract caused an increase in absorbance of 0.003/min, showing a specific activity of 0.076 Kunitz U mg-1 (Table 1), which was much lower compared to the commercial DNase I activity used as control.
Ribonuclease activity. The extract showed a specific activity of 760 Kunitz U mg-1, which was much lower compared to the commercial RNase A activity, however, the negative control did not show an increase at A260 either.
Glucanase activity. With regard to the β-1, 3-glucanase activity, the assay indicated a maximum of 6.5 U (μmol*min/mL) (Table 1), although the activity was much lower compared to one from the control enzyme used.
Preparative isoelectric focusing fractionation. A correlation was observed between fractions and pH, indicating an accurate separation on the Rotofor (Figure 1). Interestingly, the range of the isoelectric points where most of the proteins were located was between 3 and 6, though few proteins were found in the range of 6 to 10. The molecular weight of the proteins ranged from 6 to 113 kDa.
Antifungal assay. The highest growth inhibition percentages corresponded to fractions with isoelectric points (IP) between three and five, which had an average of 55%, and the ones with IP nine and ten, which had inhibition percentages of around 79%, indicating the presence of diverse active proteins (Figure 2).
Functional classification of the identified proteins. From the proteomic study performed, 46 identified proteins were chosen as potential antifungal candidates; they were classified in 33 functional categories (Figure 3) according to biological roles that could be implied in their antifungal activity. Figure 5 depicts the functional classification that was sub-grouped into peptidases (11.11%), glycoside hydrolases (58.59%), glucanases (13.13%), chitinases (9.09%), RNases (4.04%) and DNases (4.04%).
DISCUSSION
Currently the limitations of biological antifungal compounds and the negative impact on the environment of the current chemical ones have spurred an urgent need for the development of alternative means that could meet future demands of pest control. We detected 46 potential antifungal proteins and classified them in five groups based on enzymatic mechanisms that could be involved in the growth inhibition process, these groups were chitinases, proteases, glucanases, deoxyribonucleases and ribonucleases, their presence was confirmed with the enzymatic tests and mass spectrometry analysis performed. An additional group, with a higher number of proteins, was found in the mass spectrometry analysis, it corresponded to glycosyl hydrolase, which includes chitinases, glucanases, glycosyltransferases, hexamidases and exo-1,3-β-glucanases (Figure 3).
Only one antifungal protein has been isolated from G. lucidum, it is called Ganodermin and exerted in vitro antifungal action on the phytopathogen fungi Botrytis cinerea, Physalospora piricola and Fusarium oxysporum (Wang and Ng 2006). Other proteins have been characterized from G. lucidum, but their antifungal activity has not been studied, and thus our study suggests for the first time the relationship between active proteins derived from G. lucidum submerged cultures and their antifungal potential towards the phytopathogenic fungus of the banana plant M. fijiensis Morelet.
Identification of proteins involved in chitin hydrolysis
Though a few studies have made reports about G. lucidum-derived chitinases (Manavalan et al. 2015, Yu et al. 2015), our analysis reveals the identification of five different chitinases, three of which are presumed to have chitin binding capability. This information was complemented with the enzymatic tests, which indicated the presence of exochitinases, enzymes able to catalyze the progressive release of acetylchitobiose and N-acetylglucosamine from the non-reducing end of chitin. Other fungi have chitinases with antifungal activity, this is the case of Penicillium ochrochloron, whose antifungal activity against Aspergillus niger and F. oxysporum was superior when using purified chitinase, and exhibited a chitinolytic activity of 60.04 ± 0.12 UmL-1 over chitobiose and an activity of 53.84 ± 0.22 UmL-1 over glucosamine (Patil et al. 2013), these values are higher compared to the ones obtained in this study (Table 1), however this was a purified enzyme (Adams 2004). In another study one antifungal chitinase was isolated from A. niger LOCK 62 (Brzezinska and Jankiewicz 2012), obtaining a specific activity of 9.46 Umg-1. Crude and purified chitinase was evaluated against F. culmorum, F. solani, and Rhizoctonia solani. The growth of F. solani was strongly inhibited by crude chitinase (73%); the growth of B. cinerea, Alternaria alternata, and F. oxysporum was not inhibited. Compared to our results this chitinase activity is higher and the antifungal activity is a comparable, since we observed values around 80% (Figure 2).
Identification of proteins involved in peptide degradation
Proteins are important components of membranes in addition to being important structural and catalytic metabolic agents for cells. In this study, a positive protease activity was observed and the presence of five different kinds of proteases was found (Aspartyl peptidase with zinc ion binding, serine endopeptidase, aspartic endopeptidase, glutamate carboxypeptidase II and a peptide hydrolase with metal ion binding domain) (Rawlings and Barrett 2014, Yike 2011), inferring a possible involvement in the degradation of membrane proteins, which could cause a breakdown of them, or even of relevant metabolic inner peptides (Yike 2011). Compared to other studies, the occurrence of this kind of enzymes in different species of mushroom can also be related to their participation in binding to the carbon source matrix and to the plant-pathogen process interaction itself (Inacio et al. 2015). In the specific case of M. fijiensis growth inhibition experienced in this study, peptidases appeared acting like potential dissolvers of some membrane proteins, since the membrane seemed to be collapsed inside the hyphae (Figure 4). Currently few proteases obtained from mushrooms have been reported to show antifungal activity, one example is the study conducted with the fungus Aureobasidium pullulans (Banani et al. 2014), from which a purified alkaline serine protease with inhibitory activity up to 90% over the spore formation of the phytopathogen fungi Monilinia laxa, B. cinerea and P. expansum and maximum proteolytic activity of 100 U was isolated, a value higher than the one found in this study 0.610 Umg-1 (Table 1). In another study, fungal isolates from Piper hispidum Sw, showed that their proteases reduced the growth of the phytopathogen fungi A. alternata, Colletotrichum sp., Phyllosticta citricarpa and Moniliophthora perniciosa by approximately 54 to 65% (Orlandelli et al. 2015), values lower than the ones we were able to obtain over M. fijiensis Morelet (Figure 2).
Identification of proteins involved in nucleic acids degradation
We found two deoxyribonucleases, one of them is an endonuclease that catalyzes the hydrolysis of ester linkages within nucleic acids by creating internal breaks. The other is a DNase I type protein, which catalyzes the hydrolysis of various bonds, e.g. C-O, C-N, C-C, and phosphoric anhydride bonds (Nishino and Morikawa 2002). Two T2 type ribonucleases were also found, which catalyze the two-stage endonucleolytic cleavage to nucleoside 3'-phosphates and 3'-phosphooligonucleotides with 2', 3'-cyclic phosphate intermediates (Luhtala and Parker 2010). These results confirmed the deoxyribonuclease and ribonuclease tests which showed positive results. To date, several deoxyribonucleases were isolated from fungi, but none of them have been connected to antifungal activity (Chen et al. 2012, Ye et al. 2005, Zhang et al. 2014). In other organisms, like plants, some deoxyribonucleases have shown antifungal qualities, like is the case of the deoxyribonuclease isolated from Asparagus officinalis seeds: it had a specific activity of 1584 Umg-1, a value higher than ours (Table 1), and it exhibited antifungal activity towards cultures of B. cinerea but not towards F. oxysporum and Mycosphaerella sp. (Wang and Ng 2001). In another report a Pumpkin 2S albumin from Putranjiva roxburghii showed DNase activity against both supercoiled and linear DNA and exerted antifungal activity against cultures of F. oxysporum (Tomar et al. 2014a).
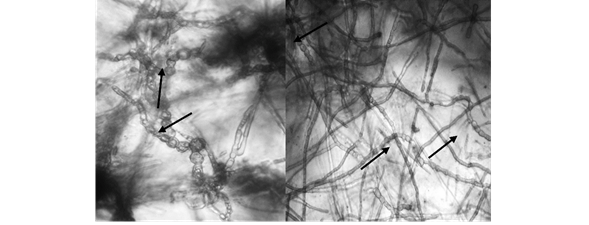
Figure 4 Collapsed hypha after inhibition assay. Photos taken directly from the wells with the Cytation 5 ® Imaging reader.
Regarding ribonucleases, in a study performed by Zhang et al 2014, one ribonuclease was isolated from the mushroom Ramaria formosa, it was proved to have antifungal and antibacterial properties, and an activity in the crude extract of 45.2 Umg-1 was observed (Zhang et al. 2015), which compared to the results obtained in this study is 760 Umg-1 lower (Table 1). Another case is the ribosome inactivating protein restrictocin from A. fumigatus and A. restrictus, which demonstrated suppressive activity towards the fungi Alternaria longipes, F. oxysporum, Colletotrichum gloeosporioides, Trichoderma viride, and Paecilomyces variotii. However, its antifungal activity was highly dependent on the integrity of the disulfide linkage (Rao et al. 2015). One ribonuclease from Pleurotus sajor-caju also displayed antifungal activity over mycelial growth of M. arachidicola and F. oxysporum (Ngai and Ng 2004).
Identification of proteins involved in glucan degradation and other glycoside hydrolases
Accordingly, in our study we identified five glucanases, the particular presence of β 1,3 glucanase was confirmed by the Laminarin hydrolysis assay, which was composed of β 1,3 glucan with 1,6 branches. Other glycoside hydrolases with different metabolic activities were found, plus other enzymes: one related to ATP and GTP metabolism and a ligase forming nitrogen-metal bonds. Glucans are a major component of the fungal cell wall. The endoglucanases are enzymes that catalyze several cleavages like β 1-3, β 1-4 and β 1-6 D-Glucosidic linkages in glucans, so that cell lysis can occur as a result of the hydrolysis of glucans (Munro 2013). The endo-β-1, 3-glucanases are abundant proteins widely distributed in seed-plant species (Magnin-Robert et al. 2007, Wróbel-Kwiatkowska et al. 2004), fewer reports are found in fungi (Murphy et al. 2012, Sánchez et al. 2012). A protein called lentin from Lentinus edodes, with N terminal sequence resembling endoglucanase, repressed growth in B. cinerea, M. arachidicola and P. piricola (Ngai and Ng 2003).
Most of the proteins found in this study belong to the category of proteins related to carbohydrate metabolism, this can be due to the fact that G. lucidum was grown using a complex source of carbon, which could induce the production of enzymes that can degrade the compounds present in the cell wall of plants and fungi (Figure 5).
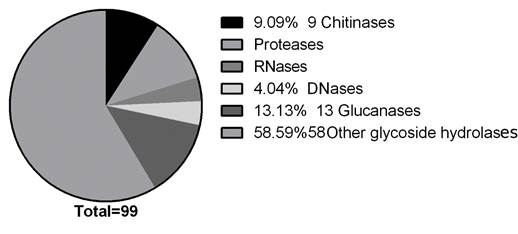
Figure 5 Pie chart depicting the identified proteins potentially related to antifungal activity of G. lucidum. Representation of proteins, identified by LC-MS/MS, classified according to their function gene ontology (GO) category and summarized in six subgroups.
As shown in Figure 3, to analyze the G. lucidum protein extract further, the 46 proteins selected were subjected to analysis by gene ontology (GO) (Yu et al. 2015). A total of 46 proteins were classified across the GO and classified into 33 functional groups, with six involved in cellular components, twelve in molecular functions and eleven in biological processes. Among these GO classifications ‘hydrolizing-O-compunds’ (seventeen proteins), ‘carbohydrate binding’ (six proteins) and ‘chitinase activity’ (six proteins) were dominant.
The mix of enzymes analyzed in this study inhibited the mycelial growth of the phytopathogenic fungi M. fijiensis, and the antifungal potential of each of the enzymes is similar to that of previously reported antifungal proteins (Binder et al. 2011, Patil et al. 2013; Tan et al. 2013, Tomar et al. 2014b, Wang and Ng 2004). Thus, the production of these proteins could have important implications on disease management in banana crops.
In addition, the presence of potential antifungal proteins with different enzymatic activities contributes to the scanty literature related to proteins in this medicinal mushroom.
However, it is not clear yet which of them are responsible for this inhibitory growth activity, or if they have a synergic effect. In summary, this study confirmed the existence of many putative proteins predicted from the G. lucidum genome, enhancing the G. lucidum protein database (Yu et al. 2015) and showing their potential to be used in the development of a bioinput for the control of Black Sigatoka Disease in banana crops.
CONCLUSIONS
This study reports for the first time an analysis of G. lucidum active proteins against the fungus M. fijiensis. We demonstrated the expression of proteases, nucleases, chitinases, glucanases and other glycosyl hydrolases enzymes, and we successfully performed antifungal assays with them. Hence, G. lucidum is a potential candidate for the production of enzymes capable of interfering in the vital development of a phytopathogen fungus like M. fijiensis Morelet.