1. Introduction
Metamorphic belts of high-pressure (HP) and/or ultrahigh-pressure (UHP) are composed of blueschists and eclogites, widely spread in the world’s orogenic belts, and are significant in understanding plate subduction and continent collision [1]. Eclogites are metamorphic rocks, which typically result from HP and/or UHP metamorphism of mafic igneous rocks (e.g., basalts or gabbros). They have been reported from several orogenic belts [1,2-17]. They are a volumetrically minor component in orogenic belts, although they record important information on the dynamics of orogens [18-21]. Eclogites in orogenic belts can be classified into two types [1,22-25]: Alpine-type (collision or continental type), which is characterized by passive margin protoliths related to deep continental subduction [13-14,20,26-33], and Pacific-type (cordilleran or oceanic type), which consists of active continental margin protoliths in an accretionary mélange related to oceanic crust subduction [34-39]. In turn, eclogites play a fundamental role as indicators of paleosuture zones [7,25,40]. However, the definition and localization of these suture zones responsible for the formation and/or exhumation of HP and/or UHP metamorphic rocks along old orogenic belts are among the most challenging questions in geological research [40], which is usually due to poly-metamorphic and poly-deformation histories, where former structures are modified and HP and/or UHP mineral assemblages are obliterated by medium-P (MP) to low-P (LP) mineral assemblages [3,8,11,15,17].According to Elvevold and Gilotti [41], while fully equilibrated mineral assemblages only record a point in the PT space, partially equilibrated rocks can record major parts of their PT evolution. The presence of inclusion suites in growth zoned garnets makes it possible to extract information on segments of the prograde PT evolution during burial to depths of 65 km or more [8,42-44]. On the other hand, several disequilibrium textures resulting from retrogression [45-47], such as compositional zoning, exsolution, symplectites, coronas and reaction rims can form during the subsequent unloading to lower crustal levels, and provide information on the shape of the decompressional PT path [41].The Arquía Complex is believed to have a complex tectonometamorfic evolution that reflects the development of accretionary edges along the northwestern margin of South America perhaps since the Paleozoic, representing an excellent example of a metamorphic complex with a typical PT path of metamorphism in subduction zones [48]. According to Maya and González [49], this metamorphic complex forms part of a suite of lithodemes that outcrop in the Central Cordillera of the Colombian Andes and consists of metagabroids, garnetiferous amphibolites, amphibole-bearing schists, quartz-phengite schists, graphite-phengite schists and quartzites of MP to LP, as well as metamorphic rocks of HP (lawsonite and glaucophane schists, eclogites and serpentinized ultramafic rocks). In this paper, we report mineralogical, petrological and geochemical data for the eclogites from the Arquía Complex on Pijao at the Central Cordillera. We suggest a PT path based on mineral relationships, mineral chemistry and reaction textures present in these rocks. In addition, we intend to contribute to the understanding of the metamorphic evolution of the Arquía Complex.
2. Geological background of the HP Arquía Complex
The N-S trending Arquía Complex occurs as a narrow, elongated and discontinuous metamorphic strip that extends from Antioquia to Nariño on the western flank of the Central Cordillera, with the Silvia-Pijao (in the east) and Cauca-Almaguer (in the west) fault systems as its tectonic limits [49-54]. According to Pardo and Moreno [50], it represents a tectonic mixture of blocks of different origins (e.g., oceanic crust, subduction zone, marine sedimentary rocks, etc.) produced by the shearing zone between the Caribbean-Colombian Cretaceous Igneous Province proposed by Kerr et al. [55] and the Colombian western margin during the Cretaceous period. Several ages have been proposed for the Arquía Complex. Nivia et al. [53] suggest that this complex is from the Neoproterozoic and link its origin to the rocks of the Cajamarca Complex. Other authors [52,56] suggest that the Arquía Complex is an accretionary complex formed in front of the Cretaceous volcanic arc or trans-American arc proposed by Pindell [57]. The MORB rocks of this metamorphic complex suggest that its protoliths originated in an environment of mid-ocean ridge or retro-arc basin, but may also include seamounts [58], which in addition to the conditions of metamorphism, is interpreted by Villagomez et al. [56] as sufficient evidence to suggest that the Arquía Complex represents a subduction channel of the Quebradagrande arc, which was exhumed between 117 and 107 Ma. Bustamante et al. [59] suggest that the Arquía Complex contains an association of heterogeneous blocks including Pre-Triassic fragments, Aptian-Albian metamorphic blocks and Maastrichtian-Danian rocks. According to Ruíz-Jiménez et al. [58], the metabasites of the Arquía Complex were formed from protoliths originated in an oceanic environment of MORB type, which represent remnants of oceanic crust likely formed in a marginal oceanic basin of supra-subduction during the Early- to Middle- Cretaceous period. Therefore, the age of this metamorphic complex is still uncertain, although its emplacement probably occurred during the Cretaceous period. The metamorphic sequence of the Arquía Complex of interest in this study extends from Génova (on the south) and Río Verde (on the north) and mainly consists of metagabroids, garnetiferous amphibolites, amphibole-bearing schists, quartz-phengite schists, graphite-phengite schists and quartzites of MP to LP, as well as eclogites and serpentinized ultramafic rocks of HP. The eclogitic rocks are typically found as meter scale lenses enveloped by strongly deformed garnetiferous amphibolites. Fig. 1 shows the distribution of metabasites (light and dark purple) from the Arquía Complex on southwestern Pijao where garnet-omphacite eclogites occur. They are represented by the HP mineral assemblage garnet+omphacite±rutile. Associated rocks are garnetiferous amphibolites and locally actinolitic schists, which show a well-developed schistosity and are in faulted contact with highly fractured serpentinized peridotites. On the western part, black graphite-phengite schists with a well-developed schistosity occur. Considering the preliminary nature of this study, the main contributions of this work are the detailed petrographic description and metamorphic petrology of the eclogites from the Arquía Complex on southwestern Pijao, Central Cordillera (Colombia Andes), including the recognition that the PT path may extend close to UHP conditions, and the proposal of alternative tectonic settings for the origin of the Arquía Complex.
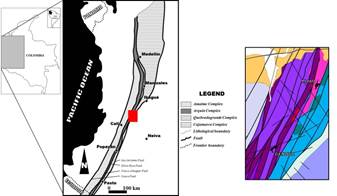
Source: Adapted and Modified from Pardo y Moreno [50].
Figure 1 Tectonic complexes of the Central Cordillera. Tectonic limits of the Arquía Complex are described in the text. (1) Sabaletas Schists; (2) Arquía Complex; (3) Lisboa-Palestina Schists; (4) Río Rosario Complex; (5) Bugalagrande Group; (6) Bolo Azul Complex; (7) Blueschists belt (e.g., Jambaló); (8) Buesaco metamorphic sequence.
3. Field sampling and analytical methods
Fieldwork was primarily focused on the occurrence of metabasites. The sampling strategy consisted of taking garnet-bearing metabasites from several outcrops in fresh road cuts and new quarries along the roads. A well exposed outcrop of retrogressed eclogites was investigated. Petrographic analyses were conducted using a trinocular Nikon (Labophot2-POL) transmitted light microscope to identify the rock-forming mineral phases. Mineral abbreviations are after Kretz [60]. Rock samples were crushed in steel crushers and grinded in an agate mill to a grain size of <200 mesh. Major, trace and REE analyses were carried out at ACME Analytical Laboratories Ltd., Vancouver, Canada. Major and trace elements were determined by wavelength dispersive sequential X-ray fluorescence (WDSXRF) spectrometry. Selected trace and rare-earth elements (REEs) were determined by inductively coupled plasma mass spectrometry (ICP-MS). The detailed analytical conditions and procedure are as follows: 250 g of rock were crushed, split and pulverized to 200 mesh; FeO was obtained by titration 0.5 [61]. Electron microprobe analyses were performed using a JEOL JXA 8800M electron probe microanalyzer of the Geoscience Department (Universidad Nacional de Colombia), under the following analytical conditions: accelerating voltage 15 kV and probe current 20 nA (analysis) and 300 nA (mapping). Data acquisition and reduction were carried out using ZAF correction procedures. Natural and synthetic minerals were used as standards. Mineral compositions were determined by multiple spot analyses.
4. Petrography
The retrogressed eclogites are fine-grained rocks consisting of garnet porphyroblasts set in a matrix of omphacite, amphibole (hornblende or barroisite), plagioclase and zoisite; rutile, often rimmed by titanite occur as the main accessory mineral phase, phengite is quite rare whereas quartz is nearly absent. In general, they show HP mineralogy with omphacite, garnet, rutile and zoisite; although these rocks show local carbonates, there is no evidence of the existence of aragonite. They are characterized by the local occurrence of fine-grained symplectitic textures, which appear as strongly lobate vermicular forms, with each lobe showing an optical continuity with its parent grain. According to Bucher and Frey [62], they are the result of hydration reaction products, such as margarite, preiswerkite and Na-Ca-amphibole (barroisite), as well as spinel, corundum and diaspore. The symplectites can consist of different mineral intergrowths, which occupy distinct domains beside each other, with an outer corona of plagioclase surrounding the symplectitic intergrowths [41]. Garnet in eclogites occurs as porphyroblasts (up to 1.0 cm in diameter), is compositionally zoned and exhibits an inclusion-rich (quartz, amphibole, zoisite and rutile) core overgrown by an inclusion-poor rim. The mineral assemblage represented by amphibole and zoisite inclusions reveals that the garnet growth history started in the epidote-amphibolite facies before entering the eclogite facies where omphacite inclusions within the rim regions should be observed. Amphibole is present as a prograde phase included in garnet and as a retrograde phase formed by the breakdown of garnet and omphacite, being strongly zoned from hornblende to barroisite. However, barroisitic amphibole is the main mineral phase occurring in these regions, which is typical along with the appearance of intermediate plagioclase (oligoclase) from retrogressed eclogites. In zoisite eclogites, garnet shows a zoisite-rich core, and the zoisite recrystallized in the matrix. Zoisite forms coarse prismatic crystals, up to 25mm long, and contains quartz and rutile inclusions. Rutile occurs as subhedral grains in the matrix and inclusions in garnet, omphacite, hornblende and zoisite, and locally in plagioclase. It is commonly rimmed by titanite. Garnet sometimes shows this reaction texture in the rim. Plagioclase showing twinning and partial alteration to sericite locally occurs in the contact between garnet and amphibole. However, it was not observed as inclusions in garnet and therefore, it cannot be considered a mineral phase from the pre-eclogite facies. Retrograde plagioclase is formed by replacement reactions taking place in intergrowths with clinopyroxene in symplectites after omphacite. Retrogressed eclogites are associated with very coarse-grained garnetiferous amphibolites, which contain subhedral to euhedral garnet porphyroblasts (up to 8.0mm in diameter). Poiquiloblastic garnet includes abundant pale green Ca-amphibole, zoisite and rutile. Fig. 2 summarizes the main petrographic features of the retrogressed eclogites and associated metabasites.
5. Whole-rock chemistry of eclogites and associated rocks
The SiO2 ranges from 47.21 to 49.30%, with Al2O3of 13.48-15.17%, CaO of 11.67-13.42% and MgO of 7.48-8.47%, with minor Na2O (2.35-2.69%) and very low K2O (0.03-0.20 %). Mg⁄(Mg+Fe) ranges from 0.14 to 0.44. The K2O/Na2O ratio varies between 0.01 and 0.09. The aluminum saturation index (ASI = A/CNK) (molar Al2O3/(CaO+Na2O+K2O)) values are between 0.95 and 1.08.
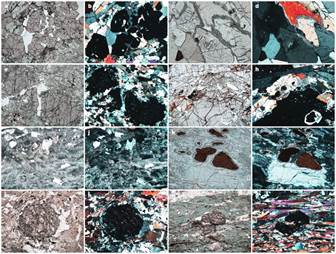
Source: The authors.
Figure 2 Photomicrographs in plane and cross polarized light illustrating typical textures in retrogressed eclogites and associated metabasites. (a)-(b) Granoblastic texture defined by primary association garnet + omphacite in eclogite. (c)-(d) Omphacite porphyroblasts are mantled by fine-grained vermicular symplectites of clinopyroxene+plagioclase. (e)-(f) Retrogressed eclogite with garnet porphyroblasts in a matrix mostly composed of hornblende. (g)-(h) Zoisite porphyroblasts around a large garnet porphyroblast. (i)-(j) Fine-grained symplectitic textures. (k)-(l) Rutile rimmed by titanite. (m)-(n) Inclusion-rich garnet porphyroblast in amphibolite. (ñ)-(o) Tectonic dissolution of euhedral garnet porphyroblast in actinolitic schist.
In the total alkali vs. silica (TAS) diagram (Fig. 3a) of Cox et al. [63], the analyzed samples have a composition similar to sub-alkaline tholeiitic basalt. The general trends of the primitive mantle-normalized [64] element concentration diagrams are shown in Figs. 3b and 3c. Chondrite-normalized REE patterns (Fig. 3b) show an almost horizontal trend in heavy REEs, except for sample ARQ-378, which shows a depletion in light REEs, followed by an enrichment in medium REEs and a depletion in heavy REEs with regards to light REEs. The La/Yb and Gd/Yb ratios of 0.78-1.31 and 1.32-1.60, respectively, reveal an igneous protolith. The La/Nb ratio is lower than 2.50 (1.40-2.15), which is common in oceanic basalts. The NMORB-normalized spider diagram of Fig. 3c shows negative Nb and Ta anomalies, except in sample ARQ-214, where Ta shows a slightly positive anomaly. A positive La anomaly is also observed. In addition, the low contents of Ti (<2%) confirm an oceanic environment affinity of NMORB type for the protoliths of eclogites.
On the other hand, the trace element concentrations of eclogites and associated rocks show a MORB-type affinity based on several discrimination diagrams [65], though detailed consideration of trace element contents will be made elsewhere.
The Nb/Y vs. Zr/TiO2 composition diagram (Fig. 4a) of Winchester and Floyd [66] was used to determine the protolith composition, considering the mobile nature of the major elements; all samples were located in the field of basalts. In relation to the tectonic setting of the protolith, according to the contents of Nb, Zr and Y, eclogites are located in the fields that correspond to basalts from normal type mid-ocean ridges (NMORB) as shown in Figs. 4b-4d.
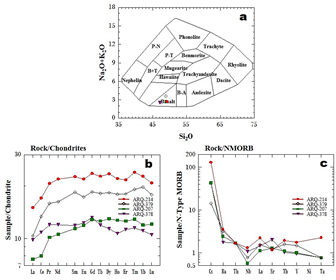
Source: The authors.
Figure 3 (a) Na2O+K2O vs SiO2 diagram (normalized values; Cox et al. [63]) for the chemical classification of volcanic rocks. (b) Chondrite-normalized REE patterns [64] for samples from the eclogites and associated rocks. (c) NMORB-normalized spider diagram [64] for samples from the eclogites and associated rocks.
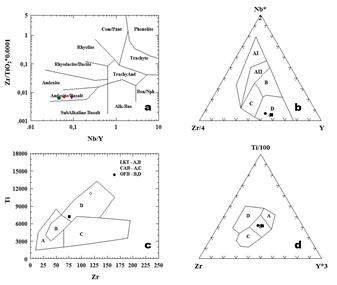
Source: The authors.
Figure 4 (a) Nb/Y vs Zr/TiO2 composition diagram of Winchester and Floyd [66]. (b) Discrimination diagram for basalts of Meschede [67]. AI, within-plate alkali basalts; AII, within-plate alkali basalts and within-plate tholeiites; B, E-type MORB; C, within-plate tholeiites and volcanic-arc basalts; D, N-type MORB and volcanic-arc basalts. (c) Ti/Zr discrimination diagram for basalts of Pearce and Cann [65]. A, island-arc tholeiites; B, MORB, calc-alkali basalts and island-arc tholeiites; C, calc-alkali basalts; D, MORB. (d) Ti-Zr-Y discrimination diagram for basalts of Pearce and Cann [65]. A, island-arc tholeiites; B, MORB, calc-alkali basalts and island-arc tholeiites; C, calc-alkali basalts; D, within-plate basalts.
6. Petrological interpretation and PT evolution
Representative mineral analyses, as well as compositions used for geothermobarometry, are provided in Table 1. Nomenclature for pyroxene and amphibole is after Morimoto et al. [68] and Leake et al. [69], respectively.
Table 1 Representative microprobe analyses from garnet-omphacite eclogite, clinozoisite eclogite and garnetiferous amphibolites (formula calculated based on four cations and six oxygens).
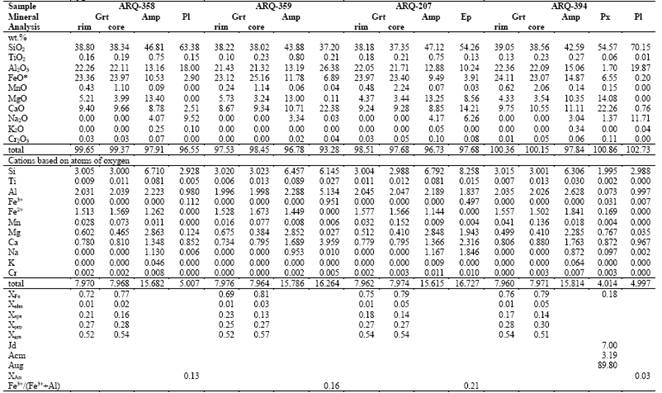
XFe = Fe/(Fe+Mg). *Total Fe as FeO + Fe2O3.
Source: The authors.
6.1. Mineral zoning related to prograde growth
Mineral zoning and mineral composition can be sensitive indicators of metamorphic reactions, and are thus of great importance in the interpretation of PT evolution of metamorphic rocks [70]. The zoning characteristics of garnets in eclogites and associated rocks from the Pijao locality are closely related to the diameter of the individual crystals and the degree of retrogression. Garnet porphyroblasts with a diameter up to 1.0 cm have commonly preserved parts of their prograde growth zoning. They are enriched in almandine (Xalm = 0.50-0.58; XFe ratio = 0.69-0.83) and grossular (Xgrs = 0.25-0.30), with lesser amounts of pyrope (Xprp = 0.12-0.23), and relatively low spessartine (Xsps = 0.01-0.05). Examples of prograde garnet zoning in the retrogressed eclogites are shown in Fig. 5. Garnet from these rocks shows strong and complex zoning, but generally with a decrease of Xsps and an increase of Xprp from the core to the rim, with Xgrs increasing from the core to the inner edge and decreasing from the mantle to the rim, with a small decrease in the XFe ratio. No increase in Xsps at the garnet rims was observed, which reflects no late resorption of garnet or cation exchange. The composition maps do not show well-preserved, idiomorphic growth zoning which is parallel to the crystal faces, except in garnet from sample ARQ-358. Modification of the prograde zoning is absent. The core region, which contains numerous epidote inclusions (in some cases defining an older foliation), tends to be homogenous with respect to the XFe ratio. The profile in this sample shows an oscillatory pattern with respect to Xgrs. However, this cannot be attributed to the abundant epidote inclusions, because there is no relationship between zoning and the distribution pattern of inclusions.
The contents of jadeite, acmite and augite in the analyzed clinopyroxene are 7.00, 3.19 and 89.80 mol%. It shows a XMg ratio of 0.82. Clinopyroxene in the symplectite with plagioclase and amphibole is diopside with low jadeite content (7 mol%). Amphibole from retrogressed eclogites is sodic-calcic, according to the classification proposed by Leake et al. [69], ranging in composition from Mg-taramite to Mg-katophorite and similar to compositions reported by previous studies [71-72]. However, amphibole in the matrix is mainly barroisite, Mg-katophorite, Mg-taramite and pargasite, with minor Mg-hornblende, whereas in the symplectite, it is Mg-hornblende, Mg-katophorite and pargasite. The SiIV ranges from 5.955 to 7.265 and the Mg/(Mg+Fe2+) ratio ranges from 0.54 to 0.80. AlIV and AlVI in amphibole are 0.735-2.045 and 0.253-1.257, respectively. The anorthite content (XAn) of plagioclase is variable in the retrogressed eclogites (0.03-0.23). The zoning patterns are not revealed yet because only symplectite plagioclase was analyzed. Epidote contains minor amounts of Mn (0.003-0.009). The Fe3+/(Fe3++Al) ratio ranges from 0.16 to 0.22. It commonly shows optical zoning, but its chemical zoning patterns often demonstrate inconsistent relationships. Rutile inclusions in garnet rims (some of which have been partially replaced by titanite) probably contain a higher Fe content, whereas less Fe-rich (more Mn-rich) inclusions are located in garnet cores.
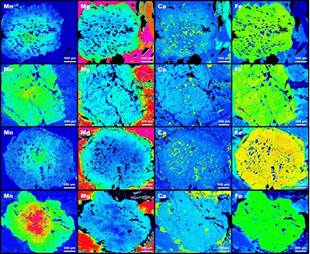
Source: The authors.
Figure 5 Mn, Mg, Ca and Fe compositional maps of a prograde zoned garnet from the retrogressed eclogites. Red shows areas of high concentration while blue represents areas of low concentration (black is very low concentration). Inclusions within the core region of garnet are amphibole.
6.2. Reaction history
The original mineral assemblages from the eclogite facies are partially preserved in some of the samples investigated. A decompression process caused the destruction of the eclogitic mineral assemblages and the formation of new mineral phases, mainly as disequilibrium textures (symplectites, coronas or reaction rims), which indicate that the reactions stopped occurring before they reached completion. The following reaction textures can be identified in the Pijao eclogites:
Transformation of omphacite (clinopyroxene) into a symplectitic intergrowth of less Na-rich clinopyroxene+plagioclase, which can be explained during decompression by the reaction omphacite + SiO2 = less Na-rich clinopyroxene + plagioclase [41,73], which can be considered a discontinuous precipitation reaction [74]. However, omphacite in the matrix is decomposed to amphibole (green hornblende to bluish green barroisite) + plagioclase symplectites. Replacement of omphacite by symplectites of amphibole + plagioclase requires participation of H2O and additional mineral phases in the reaction as suggested by Elvevold and Gilotti [41]. On the other hand, zoisite breaks down to amphibole. Therefore, we suggest the following omphacite decomposition reaction: omphacite + zoisite + H2O = amphibole + plagioclase. Rutile is commonly rimed by secondary titanite, which can be explained by the reaction rutile + SiO2 + CaO + H2O = titanite. This chemical reaction reveals that the rock experienced a change to lower PT conditions after Ppeak [75]. However, the nearly ubiquitous presence of titanite without rutile also indicates relatively low PT conditions [76]. According to Lucassen et al. [77], several interfaces are discerned in titanite growth around rutile: (1) between the Ti source and the titanite, i.e. rutile-titanite, (2) grain boundaries between titanite - titanite in the overgrowth, and (3) between the titanite and the Ca and Si source, i.e. titanite - fluid. Dissolution of rutile, shown by 3D-patterns after removal of the overgrowth, is controlled by the overgrowth patterns of titanite, especially the titanite - titanite interface. Garnet breaks down to an aggregate of plagioclase + hornblende + titanite, with plagioclase and hornblende penetrating garnet porphyroblasts, which contains inclusions of zoisite. There is no textural evidence of the occurrence of kelyphitic garnet. The original HP omphacite is usually absent in the matrix, although armored relicts are trapped in garnet and zoisite. However, matrix omphacite forms symplectites composed by Na-poor omphacite + Na-rich plagioclase, which is a typical texture in retrogressed eclogites [78-79]. The widespread development of these symplectites reveals that omphacite was abundant in the Ppeak assemblage, although the composition of the Ppeak omphacite is unknown [41].
6.3. PT estimates
There are several methods to estimate the PT conditions of metamorphism relying upon the fact that mineral pairs vary their compositions as a function of pressure and temperature. Several garnet-clinopyroxene thermometers are available for eclogites [80-85]. However, the lack of reliable barometers for this mineral pair makes pressure estimation difficult [85]. Mineral assemblages in domains formed at different stages, such as composite inclusions in garnet, minerals in homogeneous domains in matrix, those infilling cracks or pull-apart domains of garnet, and those in symplectites or reaction zones, can be distinguished based on textural relations [35]. Chemical compositions of the minerals, where more minerals are in direct contact, were selected for PT calculations. In addition, garnet and clinopyroxene rim compositions were used. Metamorphic PT conditions were estimated from the eclogites and are consistent with phase-equilibrium constraints. One of the most frequently used geothermometers for eclogitic rocks is the Fe+2-exchange thermometer between garnet and clinopyroxene, although the clinopyroxene-plagioclase symplectite geothermometer can also be used, considering that the phase transition omphacite = clinopyroxene + plagioclase is classified as a discontinuous precipitation reaction [74]. As geothermometers appear to be abundant, reliable geobarometers for these rocks are scarce, and most of them are based on reactions involving transfer of Al from tetrahedral to octahedral coordination sites [86]. Barometry is a challenging task as different barometers applied to a specific sample can produce a wide range of pressures. However, we have used the experimental data of the Johnson and Rutherford [87] and Schmidt [88] calibrations, which are expressed as P = 4.23Altot-3.46 and P = 4.76Altot-3.01, respectively. Therefore, the Al-in-hornblende geobarometer was useful to estimate the following ranges of pressures: 5.7-8.8 kbar after Johnson and Rutherford calibration, and 7.3-10.8 kbar after Schmidt calibration. Fig. 6a shows a diagram Fe/(Fe+Mg) vs. Altot, based on the calibration of Schmidt [88], who consider that the two sets of experimental data were consistent and that the offset in Al at fixed pressure was due to the higher temperature reported by Johnson and Rutherford [87]. We have estimated the following pressure conditions: 7.5-8.5 kbar, although widely spread data are in the pressure range of 9.0-11.0 kbar. Fig. 6b graphically depicts the new barometric expression and, for comparison, the calibration curves for the two sets of experiments from which it is derived. Note that the Johnson and Rutherford [87] calibration produces lower pressures and higher temperatures, whereas the Schmidt [88] calibration produces higher pressures and lower temperatures. Laird and Albee [89] compiled amphibole compositions associated with different metamorphic terrains, whose origin was associated with different ratios of pressure and temperature, which is illustrated in Fig. 6c as a AlVI+Fe+3+Ti vs. AlIV diagram. Eclogite amphibole of the Arquía Complex is located within the Dalradian, and Sanbagawa and Franciscan metamorphic belts, which were formed under middle and high P/T conditions, respectively. As shown in the Na(B) vs. AlIV diagram (Fig. 6d), analyzed amphibole belong to the shadow area of Shuksan (USA) and Sanbagawa (Japan) metamorphic belts, with pressure conditions of approximately 7.0 kbar. We also used the garnet-amphibole-plagioclase-quartz geobarometer of Kohn and Spear [89]. However, this produced very high-P conditions: 16.8-18.0 kbar. The PT conditions generated by different calibrations range from 8 to 13 kbar and from 470 to 610 oC.
7. Tectono-metamorphic evolution (reaction history and PT path)
The petrological data and mineral chemistry of eclogites to the southwestern Pijao provide insights on the P-T path of the eclogites. The tectono-metamorphic evolution of the eclogites, inferred from the petrological data and reaction curves, is given by a clockwise PT path in Fig. 7, which is defined by 4 stages. At the protolith stage (not shown in the PT path), represented by Fe-Ti oxide-bearing gabbroic rocks, ilmenite and magnetite were probably the stable oxides. The tectono-metamorphic evolution involved several aspects, including change in style of deformation, tectonic transport of the protolith to deeper levels and crustal shortening and thickening. The early prograde stage of metamorphism can be constrained from mineral inclusions in the prograde zoned garnets. The mineral assemblage amphibole + plagioclase + zoisite included in garnet cores reveals that garnet began to grow in the greenschist facies (point 1 of the PT path at about 3.5 kbar and 410°C). The second step cross the amphibolite facies is marked by entering the eclogite facies (point 2 of the PT path; maximum depth at Ppeak at about 10.5 kbar and 535°C), which was accompanied by the breakdown of igneous Fe-Ti oxides and the formation of rutile, which became the stable Ti phase. According to Ríos et al. [92], during the first phase, schistosity developed and rock-forming minerals would be those that form the foliation (zoisite, amphibole, clinopyroxene, quartz and rutile), and during the second phase nucleation and growth of garnet porphyroblasts occurred, which include some of the aforementioned minerals. After Ppeak, the tectonic transport did not increase crustal thickness; pressure did not remain constant and temperature increased during uplift until it reached Tpeak (point 3 of the PT path at about 8.5 kbar and 575°C). The transition from the eclogite facies to the amphibolite facies (symplectite stage) reflects a decompression event due to tectonic transport to shallower crustal levels and is characterized by the replacement of high-P eclogitic minerals by very fine-grained hydrated phases, where clinopyroxene (omphacite) is rimmed by a symplectite composed of Na-rich plagioclase and Na-poor omphacite. The exhumation mechanism of the Arquía Complex eclogites can be clarified by studying their decompression reaction textures, which are characterized by the occurrence of very fine-grained mineral intergrowths that replace eclogite-facies phases. In some cases, these textures appear to record a two-stage exhumation history, with initial rapid decompression (accompanied by a slight increase in temperature) followed by formation of the intergrowths on a trajectory, and therefore, the onset of symplectite growth probably marks the transition from buoyancy-driven uplift to a slower, tectonically-driven mechanism at this depth, perhaps controlled by extensional shear zones [93]. The rocks cooled through amphibolite facies conditions (point 4 of the PT path at about 4.5 kbar and 530°C), although it is not clear whether cooling took place within the kyanite or sillimanite field, with lower amphibolite facies retrogression and alteration of rutile (and probably ilmenite) to titanite.
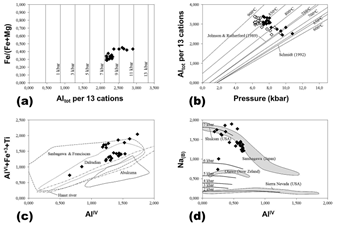
Source: The authors.
Figure 6 (a) Fe/(Fe+Mg) vs. Altot. Isobars are based on the calibration of Schmidt [88]. (b) Graphical expression of revised Al in hornblende barometer calibration. The calibrations of Johnson and Rutherford [87] and Schmidt [88] are shown for comparison. (c) AlVI+Fe+3+Ti vs. AlIV after Laird and Albee [90]. (d) Na(B) vs. AlIV after Brown [91], showing the typical pressure conditions of important geological frameworks, such as Shuksan and Sierra Nevada in USA, Sanbagawa in Japan, and Otawo in New Zealand.
8. Discussion
The tectonic development of the Arquía Complex’s eclogites is reflected by a clockwise PT path. The prograde part of the path reflects the subduction of a continental crust, under the northwestern margin of the South America plate.
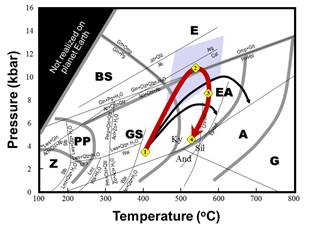
Source: The authors.
Figure 7 PT path for the retrogressed eclogites and related HP rocks of the Arquía Complex. Points 1, 2, 3 and 4 on thePT loop are constrained by textural relationships and mineral compositions. The metamorphic facies field boundaries are after Spear [70]. Z, zeolite; PP, prehnite-pumpellyte; GS, greenschist; EA, epidote-amphibolite; A, amphibolite; G, granulite; BS, blueschist; E, eclogite. The chemical reaction labeled Jd+Qtz=Ab is after Tsujimori et al. [94]. Al2SiO5 triple point from Holdaway [95].
The tectonic development of the eclogites is reflected by the clockwise PT path deduced from the retrogressed eclogites on the southwestern Pijao. This metamorphic belt has evolved into an accretionary prism that developed in the convergent margin along the northwestern continental margin of South America, which promoted a prograde part of the path, with increasing P through the epidote-amphibolite facies, as a result of crustal thickening and imbrication along N-S trending faults, as well as a probable shearing. However, evidence commonly cited for the subduction of continental margins, such as slabs of eclogitic ophiolites that mark suture zones [96] or garnet-peridotites that would suggest crustal interactions with mantle material along a subduction zone [97-98] are absent in this locality. With the convergence progression, mineral assemblages from the eclogite facies appeared. The eclogitic step certainly involved, among others, changes in the style of deformation, tectonic transport of the protolith to deeper levels and shortening with crustal thickening. During the first phase schistosity developed and minerals constituting the rock at this stage would be those that form the plane of foliation (omphacitic clinopyroxene, amphibole, plagioclase, zoisite, quartz, rutile and opaque minerals), while during the second phase, the nucleation and growth of garnet porphyroblasts occurred, which include some of the aforementioned minerals.
OurPT path records an increase in temperature from the prograde greenschist facies (410°C) to the point of deepest burial through the beginning of exhumation at HP eclogite facies conditions (~ 535°C). We consider that aPT path where Ppeak is followed by Tpeakis the most reasonable interpretation of our data, since we expect a certain amount of thermal relaxation to follow crustal thickening. The main part of the exhumation took place along a rather steep decompression path accompanied by cooling. Thrusting combined with significant erosion is the favored exhumation mechanism. Evidence for subduction of oceanic lithosphere along the continental margin is provided by the mineral assemblage garnet + clinopyroxene + hornblende + plagioclase ± clinozoisite + rutile ± titanite. The composition of amphibole shows high Na(B) and AlVI indicating amphibole formation at moderately high-P conditions. The pyroxene + plagioclase and amphibole-plagioclase symplectites suggest the presence of a Na-rich precursor clinopyroxene. The geochemical characteristics of the Arquía Complex's retrogressed eclogites indicate protoliths that correspond to basalts from normal type mid-ocean ridges (NMORB). All these features suggest that ophiolite remnants are involved in the subduction and final architecture of the Arquía Complex. Continued convergence led to the formation of the eclogite facies assemblages at the maximum depth in the continental crust. Geochronological data for the eclogite stage in the Arquía Complex are not yet available. Exhumation of the Arquía Complex high-P eclogites resulted from and occurred during tectonic emplacement associated to accretion of a terrane(?) to the pre-existing continental arc systems of the Eastern Cordillera [99]. The characteristics of the subducted lithosphere is a critical factor in determining the transition from subduction to exhumation of the high-P eclogites. However, considering the complex mélange of tectonic blocks that constitute the Arquía Complex, it is likely that the involved lithosphere slab depends on several factors, such as crustal thickness, subduction angle, convergence rate and thermal structure.
9. Conclusions
The tectono-metamorphic evolution of the eclogites, characterized by a clockwise PT path, is defined by 4 stages. Regional high-P metamorphism with peak conditions of at approximately 10.5 kbar and 535°C in eclogites have been derived for the Arquía Complex. The transition from the eclogite facies to the amphibolite facies (symplectite stage) reflects a decompression event due to tectonic transport to shallower crustal levels and is characterized by the replacement of high-P minerals by very fine-grained hydrated phases, where clinopyroxene (omphacite) is rimmed by a symplectite composed of Na-rich plagioclase and Na-poor omphacite. The mechanism of exhumation of the Arquía Complex eclogites can be clarified by studying their decompression reaction textures. However, the real significance of this metamorphic complex and its tectono-metamorphic history will become clearer as soon as geochronological data become available for the several stages.