1. Introduction
The appropriate design of encapsulation systems and controlled release systems for multiple applications in the pharmaceutical, nutrition, agricultural, textile, paints and personal care, among others, can guarantee the delivery and preservation in the short, medium and long-term of different substances [1,2]. The use of nanoparticles, specifically polymeric nanoparticles in controlled release, is a subject of increasing interest of study [3-5]. Nanoparticles of biodegradable polymers are considered important for drug delivery systems since they are very stable, easily adapt to industrial manufacturing processes over other types of nanoparticles and can be modified at their surfaces to modulate their properties [1,6-9]. Additionally, polymeric nanoparticles can be engineered to control the rate of release of drugs as well as to control their release at a specific site in the body (9). The modulation of their characteristics is achieved through the properties of the polymer and the chemical composition of the surface of the nanoparticles [9,10].
To date, conventional bulk synthesis methods for the production of polymer nanoparticles have been studied, such as nanoprecipitation, emulsion-diffusion, emulsion-coacervation, double emulsion, polymer coating, supercritical fluids and layer by layer deposition, among others [11-13]. Despite advances in each of these methodologies, the scientific community continues to face a significant challenge, namely, to guarantee the properties and reproducibility of the nanoparticles as well as to scale these systems for use in clinical trials [14,15]. The main concern in conventional bulk synthesis methods is the heterogeneous mixing. This usually takes too much time and requires a lot of energy, which results in an expensive process and produces larger and polydisperse particles [16].
Recent studies, show that microfluidic platform can enhance the controllability and reproducibility of synthesized nanoparticles compared to the conventional bulk synthesis method, because the microfluidic platform can offer precisely controlled reaction environments like rapid mixing of reagents, control of temperature, and precise spatiotemporal manipulation of reactions that are difficult, if not impossible, in larger reactors. However, the productivity of microfluidic systems is lower than that of batch reactors due to low flow rates, which can limit the application of nanoparticles synthesized by microfluidic systems [17-20].
This work introduces a system for synthesizing polymeric nanoparticles with high productivity. The approach can easily control the characteristics of the nanoparticles obtained and does not require specialized equipment. Additionally, the system is quite flexible and can produce small amounts of nanoparticles or it can be readily adjusted to produce large amounts. The system is based on a continuous tubular reactor that initially recirculates the aqueous phase. The organic phase is injected into the aqueous stream. The turbulence of the system in the pipe section leads to the immediate formation of nanoparticles by the phenomenon of nanoprecipitation. The present work evaluated the flow relationship between the two currents, the molecular weight of the polymer and the influence of the surfactants in the system.
2. Materials
Polycaprolactone (PCL) Mw=14,000, 45,000 and 80,000 g??mol-1, Acetone and surfactants (Pluronic F-127, Poloxamer 188 and Tween 80) were purchased from Sigma Aldrich
3. Methods
3.1. Recirculating system
The recirculation system is based on a continuous tubular reactor with adjustable length and tubular diameters, depending on the performance requirements. The length of the system can be varied from 30cm to 15m and the internal diameter of the reactor from 0.79mm to 38mm. For this work, the diameter of the reactor used was 3.175mm. The material of the tubular reactor used was VMQ (Vinyl Methyl-Quality) solvent-resistant silicone tubing, used in the organic phase for this study. It is recommended that materials complying with USP Class VI specifications be used. The injection of the organic phase can be modulated by changing the injection needle (18G, 20G, 21G, 22G, 23G, 25G, 27G, 29G, 30G, 31G) and modulating the injection rate, thereby controlling the particle size distribution of the nanoparticles. For this work, the 30G needle was used. Peristaltic pumps were used to control flow rates in this system.
In this system, the flow rates of the organic phase and the recirculation stream can be adjusted, varying the turbulence and the flow ratio between the two, thus controlling the size and polydispersity of the nanoparticles obtained. The recirculation system is described in Fig. 1 and the process of preparation of the nanoparticles step by step in the Fig. 2.
3.2. Preparation of the nanoparticles
To prepare the nanoparticles, it was necessary to use two phases. First, the organic phase was prepared to contain the polymer, the solvent and the active compound to encapsulate. Subsequently, the aqueous phase was prepared with deionized water and one or more surfactants. Is possible to use some additives both phases in order to modify the properties of the final nanoparticles. Fig. 2 shows the process used to prepare the polymeric nanoparticles using the recirculating system from the preparation of the precursor solutions, the operation of the recirculation system, the recovery of the nanoparticles and the characterization of the nanoparticles.
3.3. Design of experiments
In previous work carried out by the research group, it was found that the diameter of the polymer nanoparticles strongly depends on the turbulence generated in the recirculation system. For this reason, the flow rate of the recirculation system was set at 162mL??min-1 for a Reynolds number of Re=1,249 [21]. The statistical analysis of this work was carried out using the software Statgraphics Centurion XV. For this design of experiments, three variables (molecular weight of the polymer, type of surfactant and ratio between the aqueous phase and the organic phase) were used, each with three level as shown in Fig. 3, for a total of 27 trials. The concentration of polymers in the organic phases used in this work was 4mg??mL-1 and the concentration of the surfactants in the aqueous phases was 0.25% w/v according to previous tests [21]. All assays were performed with 20 mL of the organic phase and the volume of the aqueous phase was adjusted according to the ratio of each assay (40mL, 100mL, and 200mL).
3.4. Morphology
Transmission electron microscopy (TEM) with TEM microscope (FEI Tecnai T20) was used to study nanoparticle morphology. A droplet of the sample was mixed with a contrasting agent (1% phosphotungstic acid, pH 7.0 adjusted with 1M KOH solution) was placed on a carbon grid. The grid was pushed onto the surface of the droplet in order to create a film over the grid. The prepared sample was then placed in the TEM after 5 min for analysis.
3.5. Size and size distribution
The nanoparticle size and polydispersity index (PI) were evaluated by dynamic light scattering (DLS) using a Malvern Zetasizer (Nano ZS, Malvern Instruments). All measurements were carried out at 25 °C using a refractive index of 1.44 and material absorption of 0.001.
4. Results
4.1. Statistical analysis of DLS results
With the experimental design employed, it was possible to study the effect of the three design variables on the recirculation system and their effect on the diameter and polydispersity of the nanoparticles. The analysis of variance for the average diameter (Zave) of the nanoparticles revealed that the molecular weight of the polymer (p = 0.0247) and the surfactant type (p = 0.0012) have both p<0.05. Therefore, these two factors have a statistically significant effect on Zave with 95% confidence level. Conversely, the relationship between the aqueous phase and the organic phase shows a value (p = 0.4378). This result is important since it indicates that in order to control the diameter of the particles, it is not necessary to work with highly diluted systems. Such a situation favors the later recovery of the nanoparticles, reducing the cost and recovery time.
From the analysis of the multiple range test for the average diameter with respect to the polymer, two homogeneous groups were found. There is no statistical difference between the results with PCL of 14,000 g??mol-1 and 45,000 g??mol-1, nor between PCL of 45,000 g??mol-1 and 80,000 g??mol-1. However, a significant difference was identified between the PCL of 14,000 g??mol-1 and 80,000 g??mol-1, as can be seen in Table 1 and Fig. 4.
The results of the multiple range test for the Zave relative to the surfactant showed two homogeneous groups. There is no statistical difference between the results from Tween 80 and Pluronic F-127, but there is a statistical difference between these two and Poloxamer 188, as can be seen in Table 2 and Fig. 5.
The results of the multiple tests of ranges for the Zave regarding the relation of the aqueous phase and the organic phase revealed a homogeneous group and no statistical difference was found between the three levels evaluated. This result was expected, due to the statistical non-significance of this variable in the variance test. The results obtained can be seen in Table 3 and in Fig. 6.
Table 3 Method: 95% Tukey HSD for Zave and different ratios of the aqueous phase and organic phase.
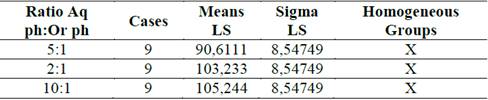
Source: Authors
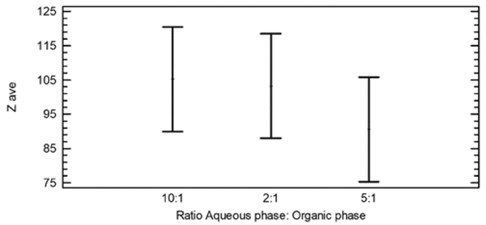
Source: Authors
Figure 6 Means and 95% Tukey HSD Intervals for Zave and different ratios of the aqueous phase and organic phase.
From the analysis of variance on polydispersity index (PI), we found that none of the variables (molecular weight of the polymer (p = 0.4208), surfactant type (p = 0.1403), the relationship between aqueous phase and organic phase P = 0.9546) has a significant effect on the PI response variable at the 95% confidence level. On the other hand, the average polydispersity of the system was 0.206 and the standard deviation among the tests for the said variable was 0.036. These results are very significant and reinforce the results from previous studies where the index of polydispersity and diameter of the nanoparticles was found to be dependent on the turbulence in the flow of the system [21]. We should point out that in this work we used a constant flow for all the tests, decreasing the variation of polydispersity index. This further demonstrates the stability and reproducibility of the nanoparticles generated using the recirculation system.
The morphology of the PCL nanoparticles obtained was determined by TEM imaging, with well-defined spherical particles and low polydispersity index (PI <0.250). No agglomeration of the nanoparticles was observed in the analyzed images. Fig. 7. shows three TEM images as well as their corresponding histograms obtained by DLS.
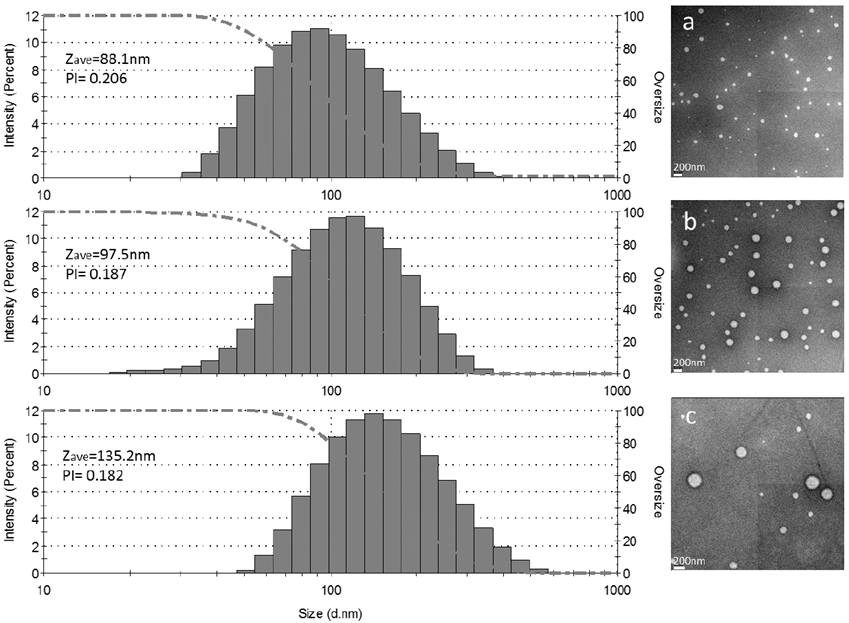
Source: Authors
Figure. 7 DLS histogram and TEM image a) PCL 14,000 g·mol-1, Poloxamer 188 and Ratio 5:1 aqueous phase and an organic phase, b) PCL 45,000 g·mol-1, Poloxamer 188 and Ratio 5:1 aqueous phase and organic phase, c) PCL 80,000 g·mol-1, Poloxamer 188 and Ratio 10:1 aqueous phase and organic phase.
Comparing the obtained results of diameter and polydispersity with other authors that employ different techniques, we find that the obtained results are quite promising. Most of the reports found in the literature talk about nanoparticles and nanocapsules but when the diameter is observed, it is usually higher than 100 nm and the polydispersity index is usually greater than 0.25. For example, Grillo et al obtained particles of polycaprolactone between 232 and 290 nm with a polydispersity close to 0.2 according to the interfacial deposition of pre-formed polymer method. The study development for Prieto and Calvo using the supercritical technic for encapsulation of Vitamin E in polycaprolactone obtain nanoparticles in the nanometer range, with mean size that varied between 8 and 276nm with polydispersity index around 0.26, which increased to 0.40 or 0.54 in some samples [22]. Othman et al. obtain nanoparticles of polycaprolactone using solvent displacement method with mean size that varied between 196 and 464nm and a polydispersity index between 0.128 and 0.389 [23]. Ezhilaras et al reported nanoparticles of polycaprolactone between 183 and 714 nm to encapsulate fish oil using freeze-drying method [24]. On the other hand, the work of Dash and Konkimalla collects different reports around polycaprolactone in which they show particles of 230-280 nm for the release of dexamethasone, particles of 257 nm for the release Etoposideproduced by the method of displacement of solvent and nanoparticles of 250 -300nm to encapsulate Tamoxifen and 180-340 nm to encapsulate Mitomycin-C using the solvent diffusion method [25].
5. Conclusion
The experimental design developed for this work showed that the molecular weight of the polymer and the type of surfactant used are relevant factors on the average diameter of the nanoparticles obtained. On the other hand, it was found that the relative proportions between the aqueous phase and the organic phase have no statistical significance on the average diameter of the nanoparticles obtained in the recirculation system developed. This is a very important result, since it demonstrates that it is possible to work with systems for nanoparticle generation that are more concentrated than commonly employed, without significantly affecting the diameter of the nanoparticles obtained. Additionally, the approach from this work allows faster, simpler and economic recovery of the nanoparticles as well as the solvent used.
The control of flow and mixing conditions in the recirculating system led to improved homogeneity of nanoparticles and control of particle size in a reproducible fashion. Continuous synthesis of nanoparticles in this recirculating system tends to have better reproducibility and controllability compared to batch-type bulk synthesis methods.
The results of the present paper show that the proposed recirculation system presents a high potential for production of polymer nanoparticles with good morphological characteristics, particle size distributions in the nano range with a low polydispersity.