1. Introduction
In time, fossil fuels (petrol, gas and carbon) will necessarily and progressively have to be replaced by renewable raw materials for fuel and the manufacture of chemical industry products. Currently, mineral oil-based lubricants are gradually being replaced by green lubricants made using renewable raw materials. In general, bio lubricants are made using vegetable oils, which have excellent properties for application such as high viscosity index, high lubricity, low volatility and, in particular, low toxicity and high biodegradability [1-5]. Aspects that have stimulated the research into and development of lubricants based on harvestable resources in many parts of the world, to promote their generalized use for some types of application. However, vegetable oils have some limitations, which have to be technologically improved, in particular, those related to their thermo-oxidative stability, crystallization at relatively high temperatures, and the limited range of viscosity values available [6-8].
In general, vegetable oils consist of a mixture of triglycerides, glycerol molecules long-chain fatty acids joined to hydroxyl groups with ester linkage. Their structure offers desirable qualities in a lubricant, their long-chain fatty acids and polar properties provide a highly resistant lubricant film which interact strongly with metallic surfaces, reducing friction and wear. In addition, the strong intramolecular interactions are resistant to temperature changes providing a more stable viscosity or a high viscosity coefficient [6,9,10].
In recent years, a great variety of oleaginous plants have been studied in order to determine their characteristics and potential of every species for use as a possible lubricant. In particular, almond oil, extracted from sweet almond seed meal (Prunus amygdalus var. dulcis), with 52 % fatty acids, of which most are unsaturated: Oleic acid and Linoleic acid (see Table 1), is a raw material of interest for the manufacture of green lubricants, for its positive behavior at low temperatures. It was found that, with respect to other vegetable and mineral oils, almond oil is more fluid at lower temperatures due to its high concentration of olefinic double bonds [12,13].
The main purpose of this study was to determine the lubricant power of sweet almond oil, in order to assess its possible use as a base for green lubricants. The potential of almond oil as a lubricant was assessed using antiwear testing and extreme pressure in a four-ball tribometer. In order to establish differences between the lubricant properties of vegetable and mineral oils, the power of sweet almond oil with no additives was compared to the behavior of two mineral oils with different levels of viscosity, used under the same conditions in tribometer tests.
2. Materials and methods
2.1. Materials
Unrefined sweet almond oil was acquired in a local market and it presented the following characteristics: density: 0.916 g/mL, peroxide value: 1.3 meq/kg a 20 °C, kinematic viscosity: 34.2 cSt a 37.8 °C. Technical grade white mineral oil and delta 360 plasticizer were used as they were received, without additional purification or additives. Their characteristics are described below: Technical grade white mineral oil: density: 0.867 g/mL, kinematic viscosity: 24.42 cSt a 40 °C; 4.94 cSt a 100 °C, viscosity index: 125, flash point: 210 °C. Mineral oil delta 360 plasticizer: density: 0.865 g/mL, kinematic viscosity: 56.50 cSt a 40 °C; 7.86 cSt a 100 °C, viscosity index: 98, flash point: 240 °C.
2.2. Tribometer tests
2.2.1. Antiwear testing
The lubricant power of sweet almond oil and the mineral oils was determined using a four-ball tribometer. AISI 52100 chrome alloy steel balls were used, with diameters of 12.7 mm and hardness Rockwell C (HRC) of 60-66. Antiwear testing was carried out using the ASTM D 4172-94 method, with the following conditions: 75 °C, 1200 rpm, a load of 147 N, test time of 60 minutes. The parameters through which to evaluate the lubricity of the oils considered were the average wear track diameter (WTD) and the friction coefficient. The WTD was measured in mm using a digital microscope.
2.2.2. Properties of extreme pressure
The properties of extreme pressure, load before visible wear and weld point, sweet almond oil and the mineral oils were determined using the ASTM D 2783-03 method, with the following conditions: room temperature, 1760 rpm, variable loads: 59, 78, 98, 127, 157, 196, 235, 314 N, time for each test: 10 seconds.
2.2.3. FT-IR spectroscopy
Analysis using FT-lR was carried out using a Nicolet iS10 spectrometer (Thermo Fisher Scientific) equipped with a deuterated triglycine sulphate detector (DTGS) and KBr beamsplitter. The equipment was subjected to an automatic dehumidifier to reduce interference from the water vapor. Using the Horizontal Attenuated Total Reflectance (HATR) sample technique, the samples were placed in direct contact with the zinc selenide crystal in the Smart ARK™ multi-bounce accessory at 23 °C. The FT-IR spectra had a resolution of 4 cm-1, the number of sweeps was 32 and the spectral range 4000-650 cm-1. The spectra were analyzed using OMNIC 9.1.27 software (Thermo Fisher Scientific Inc.).
3. Results and Discussion
3.1. Antiwear testing (AW)
The WTD and friction coefficient presented for each of the oils studied are shown in Fig. 1 and Table 2, respectively.
It was found that the balls lubricated with almond oils presented a smaller wear track (WTD= 1.63mm) when compared to the balls lubricated with plasticizer mineral oil 360 (WTD= 2.45mm) and white mineral oil (WTD= 4.96mm).
Also, the friction coefficient observed when using almond oil is lower than that observed with mineral oils. When we compare the results for the different mineral oils, we can see that the wear track and the friction coefficient are lower with the 360 plasticizer oil.
Fig. 2 shows images of the wear track, observed in a digital microscope, after the antiwear testing. Greater wear is observed on the metal surface of the balls lubricated with mineral oils, whereas the sweet almond oil exhibits a greater lubrication capacity. This difference may be explained by the structure and chemical nature of the oils. Almond oil consists of a mixture of triglycerides formed by fatty acids (See Table 1), whereas the mineral oils are made up by blends of hydrocarbons. The polar nature of sweet almond oil, makes it more suited to metal surfaces and consequently greater lubricity with respect to non-polar mineral oils, made up by aliphatic hydrocarbons [10,14].
During the process, the polar heads of the fatty acid chains in vegetable oils join the metal surfaces through an adsorption process, which allows the formation of a monolayer film with the non-polar extreme of the fatty acids, protruding away from the metal surface. As such, the hydrocarbonaceous fatty acids chains offer a sliding surface that avoids direct metal-to-metal contact, reducing the friction coefficient between the two surfaces [6].
On the other hand, when comparing only the mineral oils, the more viscous one (mineral oil 360) has the greatest lubricating power.
3.1.1. Extreme pressure (EP) properties
The performance of the oil under extreme pressure was evaluated in terms of load before visible wear and the weld point. Fig. 3 shows the behavior of the average wear track diameter based on the applied load. The chart shows a change of incline based on the load before visible wear. This load is 127 N for sweet almond oil, and 127 and 98 N for mineral oils 360 and white, respectively.
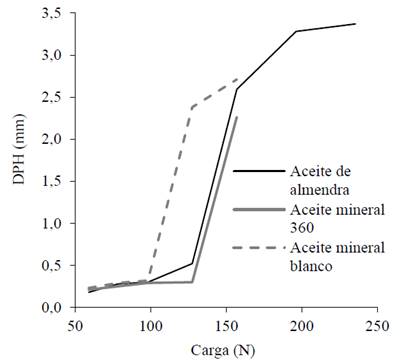
Source: Authors.
Figure 3 Average wear track diameter based on the load applied to the oils. Extreme pressure testing.
Almond oil presents a weld point at 314 N, whereas the mineral oils have the same weld point at 196 N (see Fig. 4). These results indicate that almond oil performs better under extreme pressure than technical grade white mineral oil and plasticizer 360.
This behavior is explained by the fact that the vegetable oil is made up of highly polar molecules that allow it stronger interactions with the lubricated surfaces, inhibiting contact between the metal surfaces and allowing the oil to withstand greater loads [6,14-16].
3.1.2. FT-lR spectrum: sweet almond oil
Bearing in mind that among the oils studied, sweet almond oil presented the greatest lubricant power. The oil was tested before and after the antiwear and extreme pressure testing, using FT-IR spectroscopy in order to establish variations in the chemical composition of the oil when subjected to the abovementioned processes in order to determine whether the technique presents any sign of degradation and loss of lubricant properties.
Fig. 5 shows the FT-IR spectra of sweet almond oil before and after antiwear testing.
It is possible to observe characteristic bands of olefinic double bonds (3007 cm-1), methylene group (2922, 2853, 1464 and 722 cm-1), methyl group (1464 and 1377 cm-1) and triglyceride ester group (1743, 1237, 1159 and 1097 cm-1). No changes are perceived between the spectra of fresh and used oil; there are no new absorptions originating due to oxidation products or any displacements in the bands' frequency values [17]. The IR spectra for the oil samples used in the extreme pressure tests do not present any differences with respect to the spectrum for fresh oil. These results indicate that almond oil does not suffer any changes in its functional groups in the conditions in which the tribometer tests were carried out. The results coincide with those obtained in previous studies whereby thermal analysis by DSC showed that for sweet almond oil thermal decomposition under an atmosphere of inert N2 begins at 181.48 °C and at a 174.00 °C in air atmosphere [13], so that the conditions in which the tests are carried out are not sufficient to degrade the vegetable oil.
4. Conclusions
The results of antiwear and extreme pressure testing carried out using a four-ball tribometer revealed that sweet almond oil has greater lubricant power than technical grade white mineral oil and delta 360 plasticizer. The balls lubricated with almond oil presented a smaller average wear track diameter and lower friction coefficient. In addition, almond oil performs better in conditions of extreme pressure, presenting a greater weld point when compared to the mineral oils. FT-IR Spectroscopy analysis of fresh and used almond oil allowed us to establish that in the conditions in which the tribometer tests were carried out, the oil does not oxidize. The results of this work suggest that sweet almond oil is a raw material of interest for the formulation of green lubricants. These results are a starting point subsequent studies that seek to improve the lubricant properties of sweet almond oil, by incorporating antiwear additives. The work follows a line of research that also relates the work of [18] for sesame oil.