1. Introduction
Atrazine is an organochlorine herbicide from the triazine family and is used as a selective pre- and post-emergent herbicide to control weeds and as a non-selective herbicide in non-cultivated regions [1]. Being one of the most widely used herbicides in the world, particularly for energetic biomass crops such as maize and sugar cane [2], its annual global production is between 70,000 and 90,000 tons [3-5].
Due to its high water solubility, extensive use and persistence in soil, atrazine presents a high risk of leaching, resulting in the pollution of soils and underground and surface water sources [6], which presents a potential threat to human health due to its association with endocrine disruption and possible teratogenic and carcinogenic effects [4, 7, 8]. Authors such as Graymore 2001 [9] indicate that atrazine has been found in concentrations of up to 1000 μg/L in waters adjacent to treated fields and up to 80 μg/L in drinking water (DW).
In the production of DW, the conventional or complete treatment technology (coagulation, flocculation, sedimentation and conventional filtration), is most often used; however, most of these processes were not designed to remove organic compounds such as pesticides, which due to their heterogeneity are difficult to reduce [10,11]. In contrast, one of the most used and efficient techniques for the removal of organic compounds in water is adsorption [12]. Given that production and regeneration costs are its main limitation, different adsorbent media have been implemented, derived from low-cost materials such as tires, wood, bone, fruit peels and agricultural waste, with low inorganic content and high carbon content [12,13].
In this sense, the effectiveness of the conventional treatment comprised of clarification (coagulation, flocculation and sedimentation) and conventional filtration, followed by a second stage of filtration with two adsorbent media of mineral granular activated carbon (MAC) from bituminous coal, as well as vegetal granular activated carbon (VAC) made from coconut shell, was evaluated. Raw water from the Cauca River, doped with atrazine to reach concentrations close to 0.50 and 1.00 mg/L, was used.
2. Methodology
2.1. Preparation and characterization of atrazine-doped water
Raw Cauca River Water, coming from the intake of the Puerto Mallarino drinking water treatment plant (DWTP), was doped with 0.44 and 0.86 mg/L atrazine. Turbidity (NTU), UV254 (cm-1: due to its use for the qualitative estimation of the presence of organic substances [14]) and atrazine (mg/L) were measured.
2.2. Clarification
The clarification tests (coagulation - flocculation - sedimentation) were performed in jar test devices according to the recommendations of [15] and [16] (Table 1); the experimental unit corresponded to a two-liter acrylic jar. Ferric chloride (FeCl3) was used as coagulant due to its high use in DWTPs [17,18]; a constant coagulant dose (22 mg/L) was determined from previous jar tests.
2.2. Conventional filtration
As the experimental unit for the conventional filtration and GAC filtration, transparent glass filters of 19 mm in inner diameter, 25 mm in nominal diameter and 40 cm in length were used; the filter bed thickness was 15 cm, with a metal mesh as support to avoid plugging the filter outlet with the filter medium [19-21]. The outlet consisted of a silicone hose with one end attached to the bottom part of the filter and the other end placed above the upper part of the filter bed to promote an available hydraulic load so that the bed would not run out of water (Figure 1). The experiments were performed in triplicate with a total of six experimental units.
For the conventional filtration, sand and anthracite were used with the configuration defined by [22]: sand (30%) with an effective size (d10) of 0.61 mm and anthracite (70%) with an effective size (d10) of 1.16 mm, with a flow rate of 12 ml/min. The filtration was performed for seven hours; the effluent water was subjected to the next phase of the treatment. Turbidity (NTU) (2130B) and UV254 (cm-1) (2510B) were measured every 15 minutes, and the atrazine (mg/L) (EPA 523) was measured at the beginning and end of the experiment [23].
2.3. GAC filtration
The water obtained from the conventional filtration was stored for the second filtration stage, which lasted four hours. The filtration columns consisted entirely of the selected adsorbent media: mineral granular activated carbon (MAC) and vegetal granular activated carbon (VAC) [24-26]. According to authors such as Mukherjee et al., 2007; Giraldo & Moreno 2008; Giraldo & Moreno 2012; and Vukčević et al., 2015 [27-30], the GAC adsorbent media must be washed several times to remove impurities and residual chemical substances from the GAC activation process; in this case, the media were washed seven times with distilled water. The same was performed for sand and anthracite.
The experiments were performed in triplicate using three experimental units for each adsorbent medium (MAC and VAC) and atrazine concentration, resulting in a total of 12 experimental units. A flow distribution system and a constant load tank were used for the dosing of the conventional filtered water. Figure 2 shows the experimental configuration for one concentration.
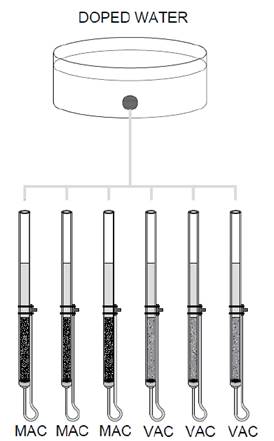
Source: The authors
Figure 2 Flow distribution system and filter configurations for each evaluated atrazine concentration.
The filtration was performed for four hours, and the samples collected during the filtration test for the two concentrations were measured for turbidity (NTU) and UV254 (cm-1) every 15 minutes during filtration; the atrazine (mg/L) was measured at the beginning and end of the process.
2.4. Statistical analysis
An analysis of variance (ANOVA), using the statistical program Minitab 17 [31], was performed to determine if there were statistically significant differences between the evaluated filter media configurations.
3. Results and discussion
3.1. Clarification
Table 2 presents the turbidity, UV254 and atrazine concentration of raw water, atrazine-doped water and clarified water.
Table 2 shows that the addition of atrazine to the doped water did not alter the turbidity level because it is primarily related to colloidal and suspended matter [32]. In contrast, the UV254 absorbance increased with the addition of atrazine due to its relationship with the dissolved material and the organic matter content [33,34].
After the clarification process (coagulation, flocculation and sedimentation), the turbidity decreased by 98 and 99% for concentrations 1 and 2, respectively. In contrast, the low performance was confirmed based on organic compound reduction in terms of UV254 and the atrazine concentration; the UV254 efficiency reductions were 38 and 39% for concentrations 1 and 2, respectively.
Regarding atrazine reduction, a reduction efficiency of 20% was obtained for concentration 2. Notably, atrazine is characterized by its high solubility (28 mg/L) and partition coefficient (2.30) [35]. According to [36], compounds with partition coefficients above 3.20 are more easily reduced by this type of process; therefore, it is likely that conventional processes are not very effective for the reduction of atrazine.
3.2. Evaluation of conventional filtration and GAC filtration
3.2.1. Characteristics of filter media and adsorbents
Table 3 shows the characteristics of the filter media and absorbents used.
Sand and anthracite, which have a smaller effective particle size and uniformity coefficient than GAC, which in turn favors the entrapment of particles that cause turbidity, were used for the conventional filtration. Thus, the conventional media are expected to have a higher turbidity reduction efficiency than that of GAC [37,38].
The GAC media used differ in the precursor and the effective particle size. In this study, MAC presented lower values; in its manufacture, there is a tendency to form a wide range of pores, and therefore, it is more often used for applications in which the compounds to be retained are of different molecular sizes, such as the substances in water, whereas the VAC manufactured from coconut shell tends to present small pores [12]. According to these characteristics, it could be expected that MAC would present a greater decrease in organic compounds.
3.2.2. Turbidity
Figure 3 shows a box-plot of the turbidity results of the conventional and GAC filtrations for the two concentrations used.
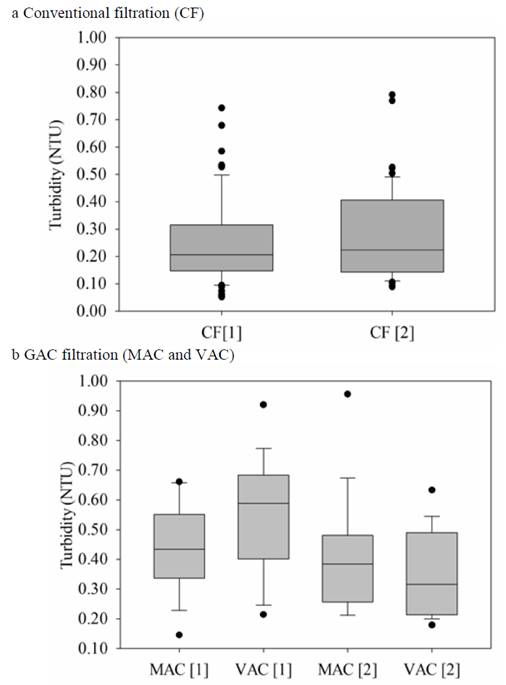
Source: The authors
Figure 3 Turbidity box-plot for: (a) Conventional filtration and (b) GAC filtration for the two evaluated concentrations.
In Colombia, the maximum permissible level of turbidity in drinking water is 2 NTU [39], whereas the Pan American Health Organization (PAHO) [40] recommends a turbidity below 1.00 NTU for achieving an effective disinfection, and the World Health Organization (WHO) [14] recommends that the turbidity median must be lower than 0.10 NTU. The Environmental Protection Agency (EPA) [41] establishes 1.00 NTU as the maximum limit for treated water and a 95th percentile of 0.15 NTU and a maximum of 0.30 NTU for the control of the microbiological risk due to cryptosporidium.
With the conventional sand and anthracite filtration, minimum values between 0.05 and 0.09 NTU were achieved for concentrations 1 and 2, respectively; in the second filtration with GAC, the minimum values obtained were higher (between 0.15 and 0.21 NTU); in this sense, authors such as Feng et al., 2012 [42], indicate that filters that use GAC are more susceptible to turbidity infiltration. Another important aspect that could affect the turbidity of the GAC filtration effluent is the washing of the adsorbent medium [27-30].
The statistical analysis by the ANOVA test shows that for the conventional filtration, there are no significant differences between the two evaluated pesticide concentrations. Likewise, no significant differences were found between the two adsorbent media used (MAC and VAC) with each evaluated pesticide concentration; notably, in all cases, the data were below 2.00 NTU, the upper limit of the Colombian standard for drinking water [39].
3.2.3. UV 254 absorbance
Figure 4 shows a box-plot of the UV254 results of the conventional filtration and GAC filtration for the two concentrations used.
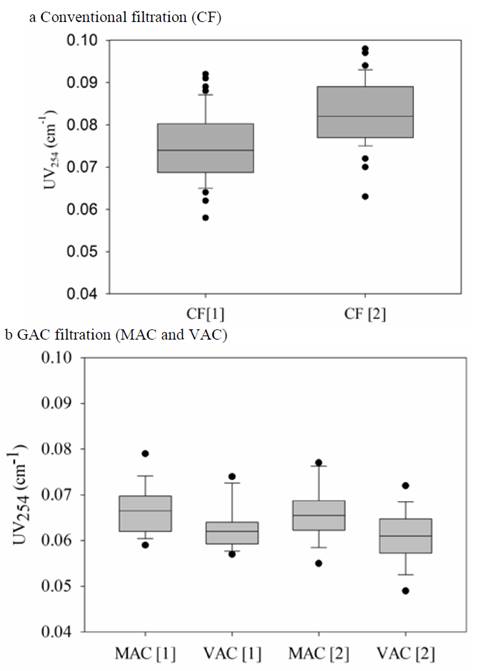
Source: The authors
Figure 4 UV254 box-plot for: (a) Conventional filtration and (b) GAC filtration, for the two evaluated concentrations.
The conventional filtration effluent varied between 0.065 and 0.092 cm-1 for concentration 1 and between 0.075 and 0.098 cm-1 for concentration 2. The ANOVA tests indicate that there are statistically significant differences between the two pesticide concentrations, being lower under the lowest concentration condition (CF1). According to [43], when using a conventional treatment with sand filters, the UV254 values of the filtered water decrease slightly.
Regarding the second filtration with GAC, lower values were obtained compared to conventional filtration (between 0.049 and 0.079 cm-1), which confirms the higher organic compounds removal capacity (represented in terms of UV254) of the GAC filter media. The application of the ANOVA tests showed that there are significant differences between the media used only for concentration 2, because this concentration corresponds to the highest doped concentration (0.86 mg/L). Previous studies have shown that GAC filters are more efficient than sand filters and dual media composed of sand and GAC or other media in the removal of organic matter (UV254) [44, 45, 42, 21], which is in accordance with this study.
3.2.4. Atrazine
To determine the reduction of atrazine, its concentration was measured in raw water (1.92 × 10-3 mg/L), in water doped with the two pesticide concentrations, and at the end of each of the following processes: clarification (coagulation - flocculation - sedimentation), conventional filtration and GAC filtration (MAC and VAC). Table 4 presents the concentrations and the reduction percentages in the different processes.
Table 4 Atrazine concentrations and reduction percentages throughout the treatment process
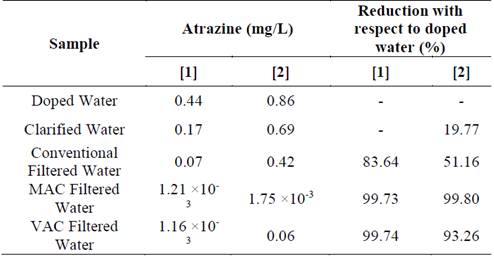
Source: The authors
Although the pesticide concentration was reduced in each stage of the treatment, the GAC filtration (MAC and CAV) stage is what obtained a sufficient reduction (99.73 and 99.80% for MAC with concentrations 1 and 2, respectively, and 99.74 and 93.26% for VAC with concentrations 1 and 2, respectively), with MAC being more efficient.
Factors such as the contact time and the presence of dissolved organic matter (DOM) in water influenced the absorption efficiency of the media used [46, 47], perhaps because the contact time may have been insufficient for adsorption of the pesticide, and the presence of DOM reduces the GAC effectiveness; these conditions can be explained because most of the results are within the limit established by the WHO [48] (0.002 mg/L), and the efficiencies with concentration 2 are lower.
Adsorption with different materials has been demonstrated to effectively reduce pesticides, such as in [49], where GAC filters were used for the removal of chlorpyrifos, diazinon and carbofuran, attaining removal efficiencies on the order of 80%, subsequent to the conventional treatment, which had a maximum removal efficiency of approximately 20%. For atrazine, wood charcoal has been used with a maximum efficiency of 95.50% [50], coconut charcoal with reduction efficiencies between 85.90 and 86.30% [3], and sawdust with efficiencies of approximately 74.70 - 80.50% [3]; notably, these studies were performed with distilled water or drinking water doped with the pesticide.
Regarding the regulation for drinking water, the EPA [41] establishes a concentration of 0.003 mg/L as the permissible limit of atrazine; the WHO [48] establishes a limit of 0.002 mg/L; and in Colombia, according to [39], the sum of the pesticide concentrations cannot exceed 0.010 mg/L. The concentrations evaluated in this study (0.44 and 0.86 mg/L) are concentrations that are found in surface water in the local and international context [51]. The atrazine concentrations at the end of the treatment process varied between 1.16 × 10-3 and 1.75 × 10-3 mg/L. Thus, they complied with the regulation in all cases other than concentration 2 filtered with VAC, which presented a concentration of 0.06 mg/L.
4. Conclusions
The clarification processes, in addition to conventional sand and anthracite filtration, reduced turbidity by 97%, with turbidity values below 0.80 NTU; however, these treatments are inefficient in the reduction of organic compounds, represented in this study in terms of UV254 absorbance and atrazine.
In general, the second filtration with both types of evaluated GAC (MAC and VAC) showed a favorable effect on the reduction of UV254 and atrazine, obtaining atrazine values within the international and national standards for drinking water.