1. Introduction
Gas Metal Arc Welding (GMAW) is a widely-used process in the welding industry because of its technical and economic advantages over other joining processes like SMAW or GTAW. Its main features include: high deposition rate, it enables to make welds in all positions and long welds without interruption, it can be operated in mechanized or automatic manufacturing systems and it offers the possibility to weld most commercially available alloys [1]. Nevertheless, one of the main problems in said industry is poor arc stability in GMAW caused, most times, by the wrong selection of welding parameters. This is the result of limited available technical information, the broad range of welding parameters (wire feed speed and voltage) provided by consumables or shielding gases manufacturers and setting welding parameters according to iterative (trial and error) or subjective criteria.
The metal transfer mode (MTM) in GMAW depends on several process variables such as polarity, current, electrode extension, wire composition, wire diameter and shielding gas [1]. Welds made with a given MTM have particular conditions of bead penetration, heat input, deposition rate, arc stability, surface condition, and spatter; thereby, a weld made with some MTM has specific performance characteristics, properties and applications. Therefore, MTM is considered an essential variable in many welding codes (AWS, ASME, API, among others) because it might impact the quality and performance of weldments, particularly, their mechanical properties.
Short circuit metal transfer mode in GMAW is a MTM characterized by the lowest range of currents and voltages of GMAW which produce small and fast-freezing weld pools by means of periodic contacts (short circuits) between the electrode tip and the weld pool. A metal droplet is detached on the weld during each short circuit. This MTM produces low heat input that enables to weld thin plates and work in all welding positions. GMAW used in this MTM is sometimes called GMAW-S and such procedures must be qualified by testing according to AWS D1.1 Code (Clauses 3.2.2 and 4.14.1) because of their tendency to produce a lack of fusion [2].
Stable metal transfer in GMAW-S requires uniform and periodic detachment. In this regard, Liu and Siewert [3] studied the change in short circuit frequency in GMAW-S caused by the variation of current and voltage. They found that the stability of the process can be described by the statistical variation of droplet-transfer period and droplet rate. Among all the statistical parameters that were examined, average and standard deviation of the transfer period were the best for characterizing the stability of the process. When maximum stability is achieved, there is a minimum average short circuit period (maximum short circuit frequency) and the standard deviation of the short circuit period is also a minimum. This indicates that, during welding, the population of short circuit periods in a stable metal transfer has a close distribution and the process is less erratic. To show the effect of welding current and voltage, Liu and Siewert plotted maps with droplet-rate (transfer frequency) isopleths in order to identify regions of maximum stability. Hermans and Den Ouden [4] also found that, when the droplet frequency is maximum, the standard deviation is minimum and the process has maximum stability. Furthermore, maximum short circuit frequency was correlated with weld pool oscillation frequency. They found that, when these frequencies are the same, the greatest stability of the process is achieved. Besides, weld pool frequency was estimated through a model developed by Sorensen and Eagar [5].
Both AWS D1.1:2015 [2] (Table 4.5(14)) and Section IX of ASME B&PVC [6] (Table QW-255 paragraph QW-409.2) consider the change in transfer mode in GMAW like an essential variable. Other codes and specifications (such as AWS D1.5:2015, AWS D1.6:2017, and AWS B2.1:2014) have identical approaches to the transfer mode in GMAW. Some of them are more specific and state that a change from short circuit transfer to globular, spray or pulsed, or vice versa, requires requalification. The GMAW-S process is not allowed in prequalified procedures and it must always be qualified by mechanical testing. However, sometimes it is very difficult to establish the actual metal transfer mode obtained with a particular set of welding parameters. There have been several studies about the simulation or prediction of MTMs in GMAW. For instance, Zhao and Zung [7] simulated the transition of metal transfer between globular and spray. In turn, Rudas et al. [8] tried to predict the MTM in GMAW through modeling. Both studies are mathematically complex and difficult to extrapolate to industrial situations. US Patent 9,808,879 B2, dated November 7th 2017, claims a welding diagnostic device for identifying MTMs during welding with GMAW and a method to identify MTMs, basically short circuit, globular and spray transfer [9]. There are no reports of a short circuit frequency map constructed for the widely-used combination of ER70S-6 filler metal and 75%Ar-25%CO2, which is one of the most preferred gas for GMAW-S because CO2 added to Argon improves wettability [1] and reduces the amount of spatter compared to 100% CO2 [10]. This work presents a particular MTM map and short circuit frequency map as tools to define boundaries of transfer modes around short circuit and process stability for ER70S-6 wire and 75%Ar-25%CO2 shielding gas mixture. The methodology for the construction of these maps is also discussed.
2. Experimental method
A Miller Invision 456MP welding power source and a Miller 70 Series wire feeder Miller 70 Series were used to run the testing welds. The welding gun was fixed and the base metal was held on a sliding or movable table, as shown in the photograph in Fig. 1(A) . Bead on plate (BOP) welds, 70 mm long, were deposited on 4.8mm (3/16”) thickness ASTM A36 steel plates, as shown in Fig. 1 (B).
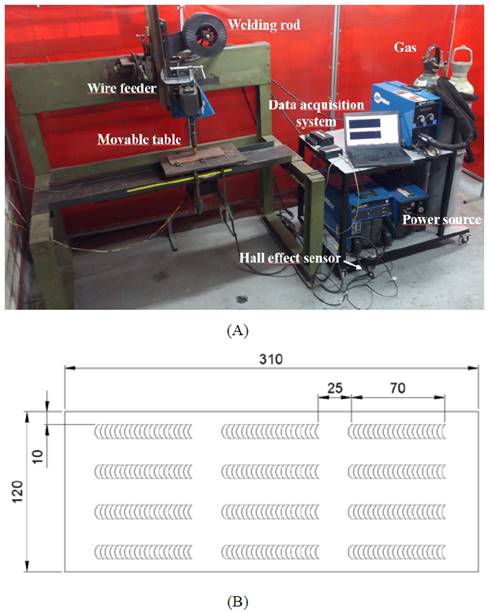
Source: Authors’ own work.
Figure 1 (A) Welding equipment and devices used during the study. (B) Dimensions in mm of the welding test coupon used for the construction of frequency and MTM maps.
The fixed variables used in the experiment are shown in Table 1 and constitute typical parameter values for GMAW applications [1]. The independent variables were wire feed speed (WFS) and voltage setting in the power source. WFS, according to recommendations given by the electrode manufacturer, was varied between 100 in/min and 600 in/min with increments of 50 in/min, and voltage was varied from 12 to 34 volts with increments of 1 volt. Two hundred and twenty (220) welding beads were applied and replicated in order to develop both frequency and MTM maps.
Current and voltage signals were recorded using a data acquisition system with a Hall Effect Current Sensor and a voltage divisor. The sampling rate was 5000 samples per minute for every signal. Signals were captured for 10 seconds during each weld deposition and stored for subsequent analysis. A moving average with a window of three data was used to smooth signals and reduce noise. The beads were visually inspected to correlate weld quality to signals, transfer modes and instability boundaries. Some typical signals and bead photos are shown in Section 3.
The first step in signal analysis was metal transfer mode identification. This step was completed through visual observation of droplet detachment during welding and the analysis of the recorded signals based on the shape of each signal and some of their statistical parameters. For short circuit and globular-short circuit transfer modes, a frequency boundary of 20Hz was established based on values given by Scotti et al [11], the US Patent 9,808,879 B2 [9] and annex C-3 of AWS D1.1 [2]. Several data and some statistical parameters were obtained and analyzed, including average arc time, average short circuit frequency, short circuit period, short circuit time, and standard deviation of short circuit frequency and period for each weld. RMS values of welding voltage and current were calculated from the recorded data. The maps were plotted with RMS current and RMS voltage because they are obtained with the usual welding meters when signals are not lineal (like current clamps specified as “True RMS”, and ammeters and voltmeters of the welding power sources). Furthermore, with direct current, RMS and average values are relatively similar and provide suitable computation values of arc power in order to make correct estimates of heat input in welds [12,13].
3. Results and discussion
3.1. Typical signals for several MTMs
Before the construction of the short frequency map, it is necessary to see the big picture of different metal transfer modes; therefore, the first step was to construct the MTM map by analyzing the characteristics of each signal obtained with different sets of welding parameters. Some typical signals registered in this study for each MTM are shown and analyzed in this section in order to describe the general method to of construction of the MTM map.
Fig. 2 shows an illustration of the detachment of one molten metal droplet in GMAW-S (short circuit MTM) and the behavior over time of the current and voltage signals during this metal transfer. The time span during which the voltage is near zero is referred to as short circuit time (instants 1 through 3). During this time, the electric arc is extinguished and the current rises towards a maximum level that depends on system conditions (wire diameter, wire material, inductance, stability and electric characteristics of the power source). As the welding current increases, so does the electromagnetic force pinching the molten metal which, along with surface tension, drives the metal drop into the weld pool [11]. The form of the current signal during up and down periods (called “Inductive effect”) maintains an almost equal slope disregard the voltage used. This is in line with the work by Scotti et al. [14], who observed that the change in voltage values does not have an influence on the up and down current rate for the same inductance setting in the welding equipment. During the short circuit time, the arc does not burn and the heat input is low due to the low voltage in this period, as shown in the instant power plot on top of Fig. 2, therefore the weld pool freezes and short circuits [4].
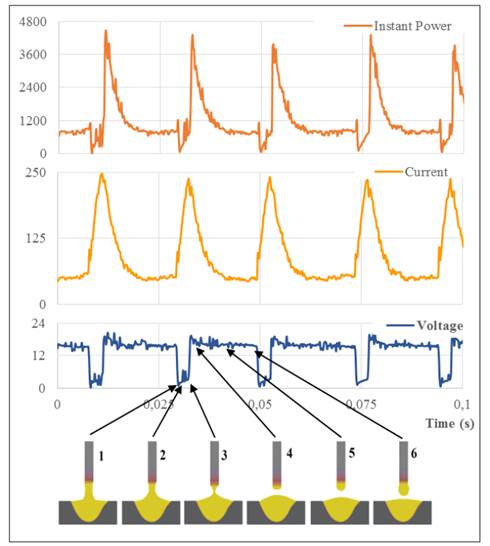
Source: Authors’ own work.
Figure 2 Schematic of the metal transfer between electrode and weld pool in the short circuit transfer mode in relation to current, voltage and instant power signals.
At instant 4 in Fig. 2, the arc is reignited and maintained until instant 6. Over this time span, called arc time, the voltage tends to be constant and near a maximum level; besides, the current begins to fall towards a constant arc current at a speed inverse to the inductance level of the power source [1,11]. During this period, the electric arc starts to form and grow a new metal drop on the electrode tip and heats the weld pool. At the end of the arc period, after instant 6, the drop touches the weld pool and a new short circuit time starts.
Other different kinds of metal transfer signals in GMAW, or mixed modes composed of two or more MTMs, were acquired during the present study. They are shown in Fig. 3 and explained below.
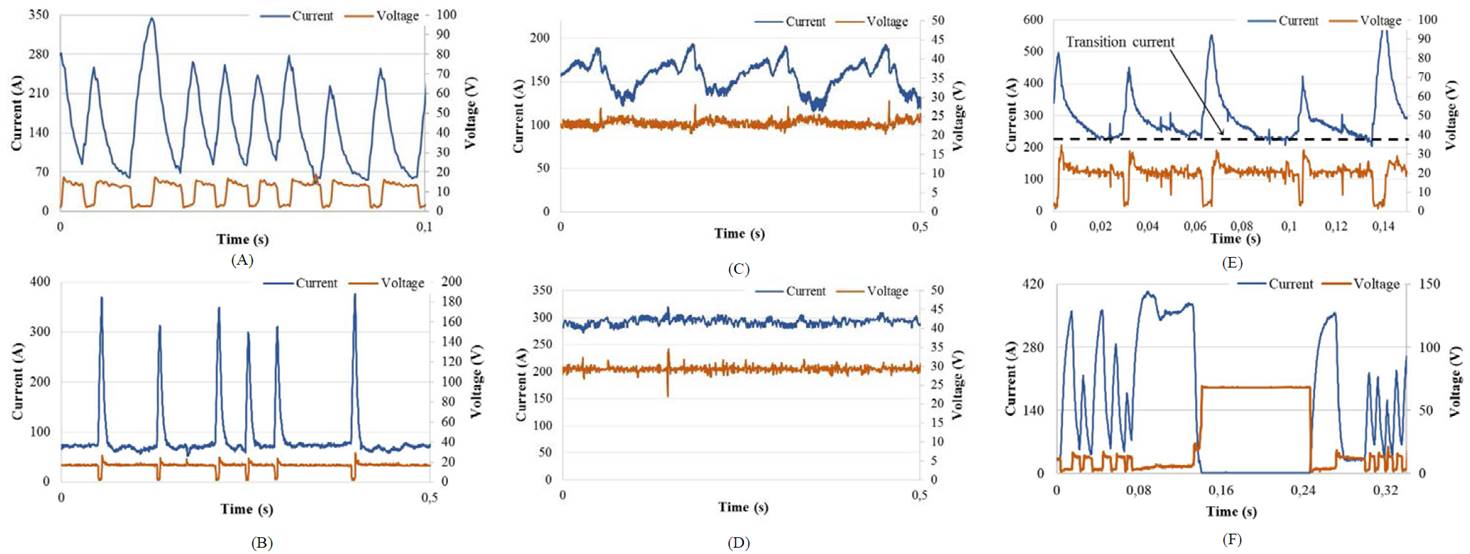
Source: Authors’ own work.
Figure 3 Signal forms acquired from several metal transfer modes: (A) Short circuit, (B) Short circuit-Globular, (C) Globular, (D) Spray, (E) Short circuit-Spray and (F) Momentary arc interruption.
Fig. 3(A) shows a typical signal for GMAW-S, included for comparison purposes. A mixed Short circuit-Globular metal transfer (Fig. 3(B)), as described by Scotti [16], occurs at relatively high voltages and low wire feed speeds. The droplet grows in a globular like shape before touching the molten weld pool and detaching. In this case, the signals are similar to those of a short circuit transfer mode (Fig. 3(A)), but with longer arc times and lower frequencies, as shown in Fig. 3(B). It could be considered to be just short circuit transfer mode, but the fact that gravitational force becomes significant for molten metal detachment enables to consider it a particular case. Welding in vertical, horizontal or over-head positions becomes difficult due to the effect of gravity on the droplet. In order to have a practical classification, a droplet is assumed to detached in Short circuit-Globular transfer mode when the short circuit frequency is lower than or equal to 20 Hz.
Globular transfer is characterized by the formation of large droplets, 1.5-3 times the wire diameter. It is a free flight MTM and the main driving force allowing metal detachment is due to gravity, with values around 0.2-1.5g-f, calculated from the formula provided by Pires et al. [15]. This mode of transfer occurs at voltages higher than required for Short circuit-Globular. Within the scope of this paper, globular transfer may refer to axial globular or repelled globular transfer modes alike. The current signal of globular MTM, Fig. 3(C), oscillates due to the change in arc length while the droplet grows with the corresponding response of the constant voltage characteristic of the power source. As shown in Fig. 3(C), voltage remains almost constant during the weld, while current varies.
Spray transfer mode is characterized by small droplets detached from the electrode tip smaller than the electrode size. It occurs at relatively high voltages and currents. The current must be above the transition current level and, because of its high value, droplet detachment is promoted by an electromagnetic pinch force [11, 15]. This MTM is common when high penetration and deposition rates are required in flat position or in out-of-position with pulsed applications. Further classification of this MTM is possible by differentiating projected spray and streaming spray, but it is necessary to use high speed filmography. Note in Fig. 3(D) that voltage and current are constant over time.
Short circuit-Spray transfer, see Fig. 3(E), is an interchangeable metal transfer mode in which current values are high enough to allow droplet detachment by an electromagnetic pinch force, but the voltage (hence, the arc length) is not enough to maintain a stable free flight transfer mode. Then, a short circuit occurs (voltage drops to zero) detaching material or even pieces of electrode and droplets detach as in spray transfer when the arc is reestablished. When the arc is momentarily extinguished at the lowest voltages (Fig. 3(F)), the current drops to zero and the voltage reaches the OCV value of the power source; this cycle may be repeated several times per second. This kind of metal transfer mode is not commonly used because it results in high spatter formation and increased weld pool size.
3.2. Metal transfer modes map and weld’s appearance
Fig. 4 shows the MTM map constructed in this study, which is the big picture of metal transfer modes for this particular gas-filler metal combination. Each point in the MTM map is determined by the analysis of the voltage and amperage signals acquired during the application of each weld, as was shown in Fig. 3. Eight different areas can be observed: short circuit (SC), globular (G), spray (S), short circuit-globular (SC/G), short circuiting/spray (SC/S), short circuit/Globular/Spray and arc interruption (AI) where stubbing begins. This map shows the short circuit metal transfer region and its boundaries in terms of RMS and equipment setting values for voltage and current. Additionally, the relationship of RMS current vs wire feed speed is shown in Fig. 5 for two groups of MTM: free flight transfer (Globular and Spray transfer modes) and contact transfer (short circuit, Short circuit-Globular and Short circuit-Spray transfer modes). The WFS vs RMS Current plot is particularly useful when the current value is known and it needs to be correlated with the WFS in order to set-up the GMAW equipment. Welds with arc interruptions were not included in some plots due to the increase in RMS Voltage as consequence of an open arc voltage (OCV), as shown in the signal of a stubbing included in Fig. 3(F).
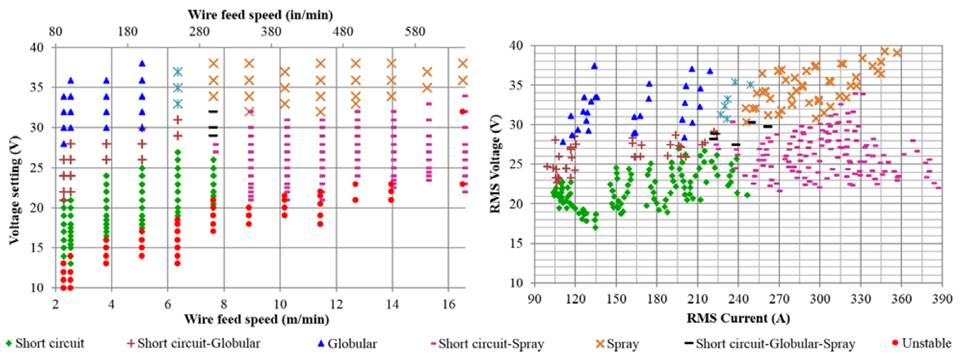
Source: Authors’ own work.
Figure 4 MTM map as a function of the voltage setting in the power source vs WFS (left) and actual RMS Voltage vs RMS current (right).
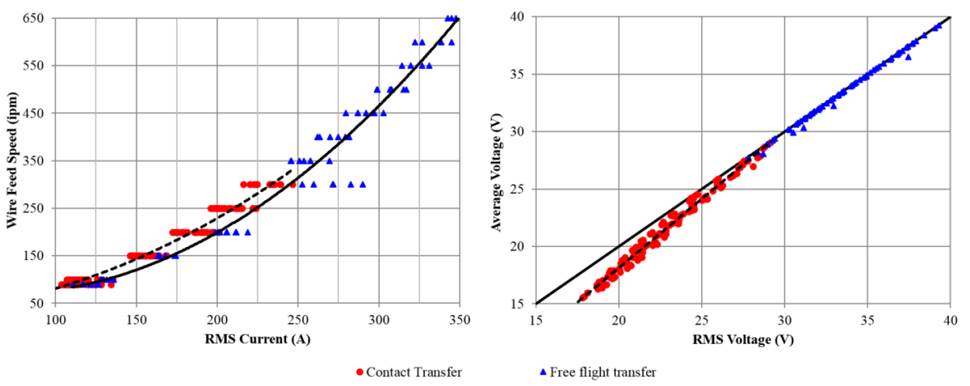
Source: Authors’ own work.
Figure 5 RMS current vs wire feed speed (left) and Average Voltage vs RMS Voltage (Right).
In each MTM area, the weld bead appearance was observed and recorded with the corresponding pictures. Photographs of typical weld beads obtained with different parameters and MTMs are shown in Fig. 6. All BOP welds in globular or mixed with globular (A, B and C in Fig. 6) exhibit spatter. Welds in unstable areas, like E and I, are not uniform in appearance, just like their arc signals, because of considerable fluctuations in voltage and amperage. Short circuiting and spray welds are smoother and present lower spatter levels as well. Interestingly, the welds in the short circuiting-spray area (a very wide zone in the MTM map, Fig. 4) are free of spatter and have a good appearance (Fig. 6(H)); this aspect, combined with relatively high arc powers (between 5 and 7 kW), opens a window to explore possibilities for use this MTM to weld higher thicknesses than allowed for GMAW-S (up to 6-10 mm).
3.3. Frequency map construction
The frequency of short circuit was determined from the voltage signal. Data were analyzed so that that every time the voltage dropped below a low threshold near zero, a short circuit was recorded and accounted for. Fig. 7 shows the distribution of short circuiting frequencies for each weld according to its welding parameters (RMS Voltage and RMS current). The frequencies reported in the graph are ±5Hz; as a result, some welds with approximately the same frequency are grouped within the same marker.
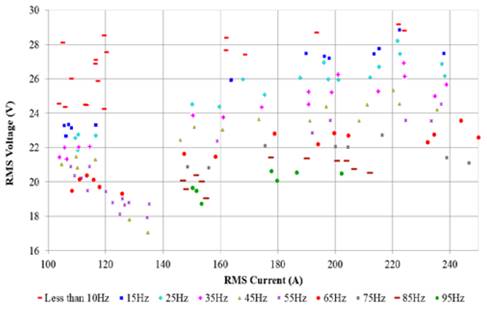
Source: Authors’ own work.
Figure 7 Short circuit frequency map for ER70S-6 electrode and 75%Ar + 25%CO2 shielding gas.
The frequency map in Fig. 7 has a similar distribution to those developed by Liu and Siewert [3] for an ER70S-6 wire with 100% CO2 and 98% Ar +2%O2 gas mixtures. However, in contrast with their findings, in this study stubbing and arc interruptions were found to take place with lower voltages at a given current value. Stubbing is an instant of welding instability that occurs when the electrode fusion rate is lower than the wire feed speed, therefore, there is contact between the solid electrode tip and the weld pool without proper material detachment and subsequent arc re-ignition [4]. This situation makes the welder feel that the weld gun is being pushed backwards and it increases the risk of lack of fusion due to an increase in weld pool freezing.
Average short circuiting period versus voltage for some of the wire feed speeds and standard deviation of single short circuit periods against voltage are shown in Fig. 8. These graphs are similar to those developed by Liu and Siewert with 98%Ar+2%O2, but constructed here for 75%Ar+25%CO2 shielding gas. In these curves, the minimum average periods and the minimum standard deviation of periods are obtained with the same voltage values for 2.28 and 2.54 m/min (90 and 100 ipm). At such voltage values, the arc is more stable, metal deposition is more uniform and better weld quality can be achieved. As a result of a decrease in just one volt, the mean period and its standard deviation increase, arc stability becomes poor due to electrode stubbing and the weld quality is affected because the electrode tip approaches to the weld pool and hits it repeatedly; the welder can feel the impacts in the welding gun. Decreasing the voltage even more produces arc interruptions and the weld cannot be deposited.
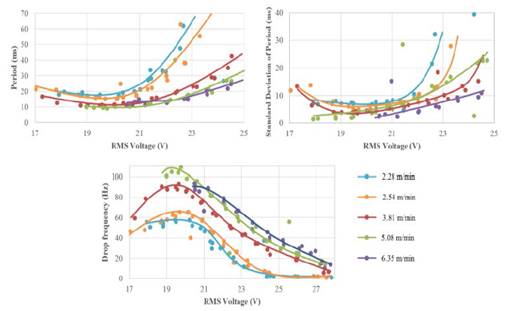
Source: Authors’ own work.
Figure 8 Average short-circuiting period (A), standard deviation of period (B) and drop frequency (C) for some WFS Vs RMS Voltage settings.
For higher WFS, the voltage value required to obtain the maximum frequency is near the area of arc interruptions and no stubbing area is present. Therefore, when the arc re-ignition problem arises, a slight increase in voltage should produce a favorable solution.
Fig. 8(B) shows drop Detachment Frequency Vs. RMS Voltage. Typical bell shaped curves, as those presented by Houldcroft [17], were only achieved with low wire feed speeds (2.28, 2.54, and 3.81 m/min, i.e. 90, 100 and 150 ipm-). At these wire feed rates, maximum stability (maximum drop detachment frequency) was found around 20 volts. Nevertheless, for higher rates, maximum stability is achieved by reducing voltage, but not as much as to cause stubbing.
A simplified short circuiting frequencies map is shown in Fig. 9. It is formed by isopleths drawn on a Voltage vs. Current coordinate system. It was constructed by tracing lines through points of equal frequency and defining areas where a given detachment frequency can be located. Boundaries between the short circuit transfer mode and other modes were also traced. A black solid line indicates electrode stubbing and the arc interruption limit, beyond which arc cannot be established due to excessively low voltage.
4. Applications of the short circuit frequency map
In the industry, it is very common to use the two following sets of parameters to qualify welding procedures for GMAW-S with ER70S-6 of Ø0.045” and 75%Ar-25% CO2 shielding gas: (1) 190 amperes and 24 volts and (2) 170 amperes and 19 volts. These two sets are taken as examples to explain the way in which the short circuit frequency map can be used in connection with the requirements of AWS D1.1:2015 to define limits for welding procedures specifications (WPSs). Points A and B in Fig. 10 represent 190 amperes and 24 volts and 170 amperes and 19 volts, respectively. Shaded squares were drawn to represent the current and voltage variation ranges where depositing of production welds are allowed with the same procedure qualification record (PQR), in accordance with the limitations stated in AWS D1.1:2015 Structural Welding Code-Steel [2]. These limitations are established for GMAW and are also applicable to welding in short circuit transfer mode (GMAW-S). In this particular case, the current and voltage can change ±10% and ±7%, respectively, from the values recorded during welding the qualification coupon. For example, for the procedure qualification record corresponding to the case A, the code would allow to make welds between 171 and 209 A and between 22.3 and 25.7 V (shaded in green); for point B, the code would allow to make welds between 144 and 176 A and between 18.4 and 20.3V (shaded in blue).
However, in the case of point A, the square includes several metal transfer modes (Short circuit and SC/Globular) and this would be a change in an essential variable for the GMAW process according to Table 4.5 of the code (Variable 14). For point B, the window includes the interruption arc area where the quality of the beads is not consistent and it is possible to find stubbing, lack of fusion and arc interruptions, which make the process difficult to operate by welders. Suitable operation parameters may be found for the definitive WPS drawing the windows allowed by the welding code in the short circuit frequency map and taking in consideration the boundaries where a change in the MTM may occur or where a poor arc stability compromise the quality of the production welds. Engineers or inspectors should consider them so that suitable parameters are achieved during the development of their WPSs, which must meet both the Code and the technical feasibility to produce good welds.
5. Conclusions
A short circuit frequency map for ER70S-6 steel wire of Ø1.14 mm using 75%Ar-25% CO2 shielding gas was constructed based on voltage and current signals. Said map proved to be a tool that offers a graphic representation to identify the stability areas for the GMAW-S process and boundaries with different transfer modes.
High short circuit frequencies (>50Hz) are recommended during welding when high quality is required. A broad range of parameters in the short circuit frequency map can be used to achieve these frequencies.
With low WFS (2.28 and 2.54 m/min), the highest recorded short circuiting frequencies were 58 and 65.5 Hz at 120A/19.4V and 118A/19.7V, respectively. These parameters also exhibit the minimum standard deviation of the short-circuiting period for each WFS, which is consistent with the studied literature.
With higher WFS (3.81-6.35 in/min), the maximum short circuit frequency is located near the instability limit. Therefore, a little voltage reduction may cause instability during the weld and, as a result, care should be taken when such parameters are being used.
Weld beads with good surface appearance were obtained with short circuit and spray MTM, even with short circuit-spray. Globular welds exhibit spatter. Welds in unstable areas with low voltages are very poor in quality and surface appearance.