1. Introduction
Sustainable development is defined as development that ensures the needs of the present without compromising the ability of future generations to face their own needs [1]. This implies an adequate planning regarding the use of resources in the short, medium and long term, and requires an analysis of the threats and opportunities of human beings acting on their environment. Sustainable development seeks a balance between economic growth, social progress and ecological balance [2], this is known as the three pillars on which sustainability is based and contributes to an increase in the welfare and progress of present and future generations [2,3].
Sustainable companies, which use the World Business Council for Sustainable Development as a global regulatory body, are important players in the search for a sustainable world as they care able to develop cleaner production processes that have a positive impact on the environment. A sustainable business creates economic, environmental and social value in the short and long term, thus contributing to the increasing welfare and progress of present and future generations [4].
This article aims to build a model that allows the integration of different elements that interact within the organization and operation of a company, incorporating elements of business sustainability, all with an emphasis on the production process. The main variables and their interrelationships will be identified, allowing decision making that favorably influences the development of the environment and improves the sustainability conditions of the business.
Considering the model was structured in a global manner and under the system dynamics approach, its description and analysis is the basis for its application in other companies of lesser or greater complexity. Moreover, it integrates components of sustainable development with the manufacturing process and the quantitative model.
The model’s construction is based on the characterization of aspects related to sustainable development and business sustainability, while the modeling blueprints with respect to business sustainability and the fundamentals of system dynamics. It continues with the identification of variables and their relationships through the causal diagram, the explanation of the model, and its composition and interrelations based on the structure and functioning of a manufacturing company which served as a reference for data collection and modeling. Finally, the analysis and results of the simulation and the evaluation of the model are laid out.
2. Business sustainability and modeling
The objective of a sustainable business is the creation of value [5] which can be achieved through the company's own commercial activity, a decrease in the consumption of electricty, water and other raw materials, the use of new technologies, and product innovation; all of which generate greater profitability and impact on the needs of consumers measured through indicators. These are defined as aspects of interest for the company and are analyzed based upon general guidelines for corporate sustainability [6].
Corporate sustainability includes three dimensions known as The Triple Bottom Line: social progress, economic growth and ecological balance [3]. The balance of these three dimensions seeks the long term profitability and permanence of companies. The sustainable company does not only partially compensate for the negative effects of its actions, and further integrates within its strategies the three aforementioned parameters [7]. Regarding the modeling of business sustainability, partial analyzes can be found where modeling is carried out from the production process’s perspective, certain parts of the business, and problematic situations regarding business dynamics. However, components of sustainable development are not integrated within the manufacturing process and the mathematical model [8-11].
Forrester, the creator of System Dynamics, lays out in his book Industrial Dynamics several examples for application in a company, including: model of the production-distribution system; the dynamic characteristics of a client-producer- employment system; and industrial dynamics in business [8]. Later, in the 90s, [9] he proposed and updated the concepts of system dynamics and incorporated mathematical elements that are typical of dynamic systems. In addition, the book explains characteristic behaviors in the different systems studied called archetypes. These serve as a reference to identify, design and analyze models.
A methodological approach for organizational modeling under a system dynamics approach is presented in [10], laying out a structured development of company modeling. Conversely, the use of case studies for the understanding of the concepts and methodology of system dynamics is used by [12]. An integrated sustainability model that includes the management, measurement and social responsibility of an organization is presented in [13]; concepts of sustainable development are raised in the general context and in the business world, integrating the dimensions of sustainability (social, economic and environmental) and incorporating product management as a key component of the model.
Other management models applied to sustainability are outlined in [14]; that proposes sustainable management as an integrating activity in the company. The self-management model [15] explains that workers take a very active role in the company and become its owners. This model conceptually describes the organization and its regular functioning, without establishing numerical or mathematical type relationships. In the mining sector, the incorporation of elements of sustainability has also been proposed as a strategy to increase productivity and meet the technical, normative and social responsibility requirements for this industry [16,17].
In [18], a study is presented on multilevel distribution chains where system dynamics are used to perform a multi-product modeling. Whereas in [19], some models are proposed for business management settings which make use of system dynamics. The textile industry has also been subject to modeling from perspective of system dynamics [20] through a model that is based on the management of production. The CEO of the company DPS Telecom develops a process of modeling his company that is carried out under the methodology of system dynamics [21]. It starts with a simple model until a complex modeling of the organization is achieved and decisions can be taken. The Viable System Model presents the approach of a business model integrating business sustainability, based on the guidelines of the ISO 26000 [22].
System dynamics modeling has applications in different areas [23], therefore, this article links it as the methodological component for the approach and construction of the business sustainability model: the modeling is done from a structure and behavior viewpoint. The structure is analyzed from its composing elements, its relationships and characteristics. Behavior is studied from the evolution of the variables through time. Through simulation medium and long-term behaviors can be estimated and decisions made based on the changes recorded by simulated variables. [24-27]
3. The model
The construction of the model is based on the system dynamics through which complex systems are modeled, requiring an analysis of their components and a global understanding of their operation. [6,11-13,18,20,21,28-34]. System dynamics can be used to model complex systems from the most diverse fields: from engineering to social and economic sciences [32-36]. System dynamics is considered to be a scientific tool for the construction of system models which can be simulated with computers [6].
3.1. Theoretical foundation
System dynamics starts with the definition of the problem, characterization of the system to be modeled, identification of the variables, the construction of the causal diagram, the Forrester diagram, model verification, simulation, and finally, the analysis of results. The causal diagram allows the recognition of dynamic system structure by defining relationships between the variables. The Forrester diagram is a formalization of the causal diagram and shows the relationships between the state, flow, and auxiliary variables [9-11]. The model obtained is considered a dynamic system and this, in turn, is defined as the mathematical object constituted by the space of states and rules which determine its evolution. [9-11]. The space of state refers to the space of the dimensions formed by the state variables.
State variables, also known as level variables, characterize the system at a given time or state. They represent accumulations and inventories, and also reflect a delay in the flow of material or information. They are important in identifying the evolution of the system. The state variables are linked by at least one variable of flow that can be input or output. These determine the increase or decrease of the state variable.
Auxiliary variables are intermediate variables which affect the flow variables or other auxiliary variables. They can also be related to state variables and constants, such as rates. The parameters are constant values or of very slow variation, they are related to auxiliary variables and flow variables, but they are not influenced by other values or variables. During the analysis of the model, the parameters are varied in order to evaluate the behavior of the model. The model is verified based on the coherence of the results obtained against the simulated real system and the expected behaviors.
3.2. Description of the model
The construction of the model is based on the structure and functioning of a Colombian manufacturing company, which is taken as a reference. The causal diagram was constructed based on a general identification of variables and relationships, (Fig. 1). In this diagram, the following points are highlighted: customers taken as a starting point for the dynamics of the model. The industry component which corresponds to the production system of the company: production, inventory and dispatch of finished products. The environmental component includes energy consumption and the generation of solid waste taking into account that they are two of the main indicators for the company under study. The social component refers to the variation of jobs based on production levels, training, and productivity.
From the causal diagram, the variables and parameters were adjusted, specified and classified. The model (Forrester Diagram) was built, comprising of two sub-models: The manufacturing model and the sustainability model. The model was made up of four (4) state variables (four-dimensional model), seven (7) flow variables, twenty-three (23) auxiliary variables and twenty-seven (27) parameters. Two negative feedback cycles were identified: one related to the energy saving program and the other was related to human talent management.
3.2.1. The manufacturing module
The manufacturing module has the aim to model the production process from the intake of raw materials to the preparation and dispatch of the finished products (Fig. 2). The company performs production scheduling based on the demand (production orders) and the levels of losses that occur in the manufacturing process. Regarding the raw materials inventory, the interest is focused on those products that are supplied by international suppliers and whose stock in the warehouse must guarantee three months of production. This inventory is adjusted based on a standard reference value established by the company.
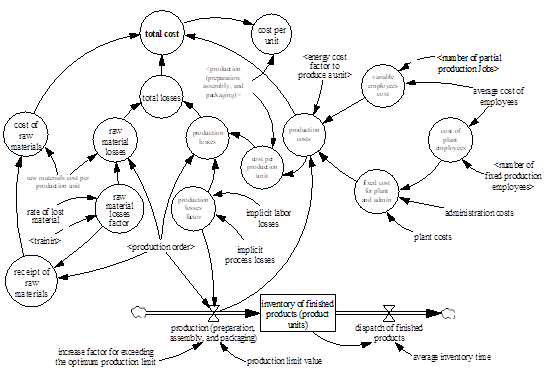
Source: The authors
Figure 2 Representation of the manufacturing module including technical and economic aspects and the productive process of the business
The production (preparation, assembly and packaging) is impacted by production or material losses, and by the levels of equipment operation capacity or worker productivity, this in turn is reflected in defective material parts or units. The manufactured products go to the inventory of finished products. There is a maximum storage capacity, a maximum time of permanence, and a dispatch volume of products which determines the dynamics of said inventory.
The operating costs of the company are differentiated by the areas of interest identified in the structure of the proposed model. The income is given by sales of products and green income, which corresponds to the total income. The profit is equal to the total income minus the total costs.
3.2.2. The sustainability module
In the sustainability module, everything related to solid industrial waste, energy consumption and human talent management is integrated. Each of the sub-modules are displayed independently. Fig. 3 shows the structure of energy consumption in the company, this is an important aspect in the sustainable development of a company.
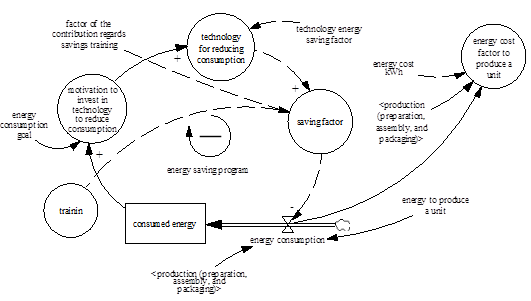
Source: The authors
Figure 3 Sustainability module from the point of view of the energy saving component
The energy saving factor is based on a maximum energy consumption goal. When reaching this goal, saving actions should be strengthened, especially with the employment of technology to reduce consumption. The monthly energy consumption is given by the factor of energy consumption per product manufactured, multiplied by the quantity of products manufactured in the same time period. This energy consumption depends, not only on production levels, but also on the implementation of energy saving strategies. The expertise and level of training of operators is another factor which contributes to the reduction of energy consumption levels.
To be more precise, the company establishes training and training programs according to the number of contracted operators. The greater the number of contracted workers, the greater the emphasis placed on human talent training and management programs (Fig. 4).
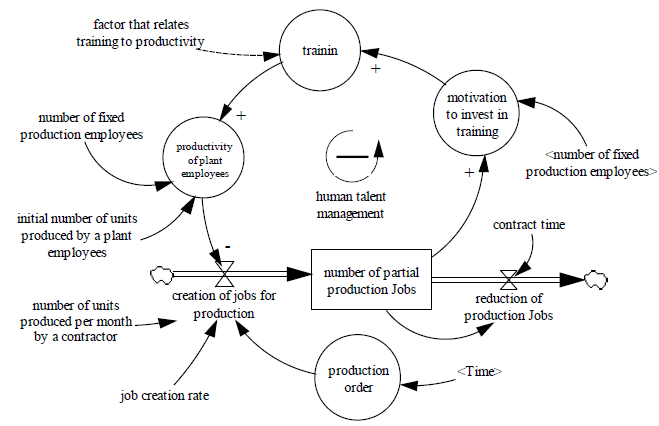
Source: The authors
Figure 4 Sustainability module from the human talent management component perspective
With fixed workers, also known as plant workers, certain levels of productivity can be achieved. When a production order exceeds these levels, temporary workers must be hired, while the needs of production volumes are met. The number of workers to hire is given by the difference between the level of productivity and the production order, divided by the number of units that a contractor can produce.
Solid waste (Fig. 5) refers to “Scrap”, industrial solid waste such as sheet metal.
4. Results and analysis
Based on the records of monthly production, it was found that the production orders were adjusted to a normal distribution with an average and deviation of approximately 39,000 and 8400 units respectively, with minimum reference values of 20,000 and a maximum of 65,000. With reference to the above information, the orders were generated randomly (Fig. 6) following a normal distribution. It was observed that the minimum value generated is 20,868 and the maximum value 58,913. To this end, a process of orders was simulated, coherent with a realsitic context of a company woth a 48- onth outlook.
The production order determines the levels of receipt of raw materials factoring in the losses of raw materials, which, in turn, is defined by the rate of material loss and the levels of personnel training. This means that there is a difference between the production orders and the receipt of materials. This difference also means that production levels are lower than the order, leaving products pending for the next production order. To correct this situation, it can be established that the production orders include, from the beginning, the additional percentage of raw materials necessary to satisfy the volumes of products requested. On the other hand, production is also affected by the size of the production order. This is due to the fact that there is a production limit value (PLV), in this case of 35,000 monthly units that, when exceeded, the effectiveness of control over the quality levels of some products is lost. Therefore, the efficiency of the productive process is also affected. The production loss factor keeps increasing until it doubles.
With respect to the inventory of finished products (Fig. 7), it is observed that these are accumulated despite the fact that there is a constant dynamic of products being dispatched.
The accumulation of finished products is the result of several aspects, including: the policy on product consignment for some clients that are influential and have high levels of contracts with the company, and the impossibility of quickly dispatching all products to customers which affects demand in the long term.
The products which are in the inventory of finished products are sent to customers based on the maximum time of permanence in the inventory, which is 3 months. The dispatching of finished products (Fig. 8) has a consistent behavior with the inventory of finished products, since the shipments depend essentially on the quantity of products that are in the inventory, the time of permanence, and the client’s requirements.
With regards to energy consumption, this depends on the levels of production and energy consumption per unit. However, it can be modified through training processes where operators can upgrade their work and make better use of their time, which contributes to energy saving. In the same way, the use of technology is an alternative that can contribute to cost reduction through a more efficient use of energy. While measures are not implemented in the energy saving factor of technology (equal to 0), this will accumulate over time without variations in the slope (Fig. 9).
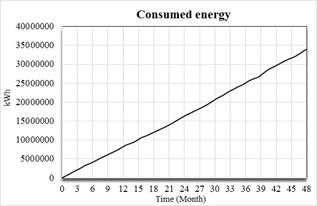
Source: The authors
Figure 9 Energy consumed when the energy saving factor of the technology is equal to 0.
When a consumption goal of 6 million kwh (the reference value of the company from which the energy saving technology is applied) and a saving factor of 0.1 (Fig. 10) are established, it is observed that the slope changes to a lower value compared to what is observed in Fig. 9.
When establishing a technology saving factor equal to 0.3 (Fig. 11), it is observed that the slope changes to a lower value after period 12. With a technological energy saving factor of 0.5 (Fig. 12), the value of the slope decreases from period 9, an impact that will be reflected over time.
When evaluating the energy cost factor of producing a unit in front of the variations that occur in the technology energy saving factor, rational changes are visualized in Figs. 13, 14, 15 and 16. In the first case (Fig. 13), When the technology energy saving factor is zero, the energy cost factor of producing a unit decreases a little at the beginning ($20); later, from period 3 it maintains a marginal decreasing trend with minimal variations. In the second case, when this factor is 0.01 (Fig. 14), similar to the previous case (Fig. 13), the energy cost factor of producing a unit decreases a little at the beginning ($20) and then after period 9 an additional decrease is observed, of $80 per unit regarding its energy cost. When the savings are 0.3 (Fig. 15) and 0.5 (Fig. 16), the energy costs of per unit of manufactured product from period 9 decrease by $1,800 and $3,000, respectively.
These variations in the energy cost factor of producing a unit can be taken by the company as a basis for its energy saving decisions.
Evaluating the behavior of waste (Fig. 17), it can be observed that variations are presented that are coherent with the quantity of units that contain each of the production orders. In addition, as time passes there is a slight tendency of growth.
This increase is due, in some cases, to the greater generation of waste caused by increases in production levels, inefficiencies in the production process, efficiency losses in the use of some waste, or the generation of lower quality waste, limting its use. However, the percentages of waste generation are below 1%. Furthermore, the use of waste also has a slight upward trend, which may be due to a more efficient process or the generation of greater volumes of waste.
Another interesting item to evaluate is the green income (Fig. 18), it is evident that the monthly income of this is estimated at $10,670,000. However, the company can strengthen its policies and actions to use this waste in a better way, taking into account that the recovery rate is at 0.7.
5. Conclusions
A systemic vision of the company allows for an understanding of the systemic behavior of the organization’s key aspects, for example, how its decisions can impact on other components within the organization, especially those that are subtle and not easily perceived. Furthermore, the effects can accumulate over time and when they are detected it may be too late to take action to correct the problem.
A four-dimensional model was built for a company, based on the fundamental characteristics of the production process, incorporating elements of business sustainability (environmental, economic and social dimensions) and quantitative modeling. The model was integrated by two large modules which were designated as the manufacturing module and the sustainability module. The first covered the physical and economic component of the production process and the second was divided into sub-modules related to energy consumption, green income, and human talent management. Reference was made to environmental, economic and social indicators, where at least one indicator of the triad was incorporated. Based on the model, some variables of interest were analyzed, such as the storage of finished products, the energy consumed, and the generation of solid waste.
The company incorporates the just-in-time concept into its management, however, when evaluating its policy regarding the handling of inventories, in particular of finished products, as it was found that they accumulate slowly over time. This requires the company to make an assessment of storage costs and opportunity costs against the optimal quantities of inventory that could actually be maintained to ensure the continuity of the production process. Similarly, redefining their policies regarding the consignment of products for their main customers will streamline the movement of these stored products.
Another interesting aspect was related to energy consumption. While measures are not implemented in the technology energy saving factor (equal to 0), this will accumulate over time in direct proportion to the levels of production and inversely to the levels of efficiency and productivity. When a technology energy saving factor equal to 0.3 was established, it was observed that the slope changes to a lower value after period 12. With a technology saving factor of 0.5, the value of the slope decreased from period 9 onwards. Regarding the cost of energy per unit of production, it remained constant when the technology energy saving factor is zero. When the savings were 0.3 and 0.5, the energy costs per unit of manufactured product decreased by $1,600 and $3,000, respectively, from period 9.
With regards to waste generation, in this case, of solid waste; it was determined that its growth is mostly due to increases in production levels, inefficiencies in the production process, efficiency losses in the use of some waste, and the generation of lower quality waste with a limited use.
Finally, since it was structured under a global and systematic scheme of operation and business organization, the developed model and its corresponding methodology forms a basis for its application to other companies of lesser or greater complexity.