1. Introduction
Polyhydroxyalkanoates (PHAs), are biopolymers synthesized naturally by a wide variety of bacteria. These are especially interesting since their characteristics, such as melting point, tensile strength, and elongation, are similar to the physical properties of the materials conventional petroleum derivatives [1]. In addition, these polymers are completely biodegradable, possess thermoplastic and biocompatible properties that allow their use in the pharmaceutical medical area, in the manufacture of suture threads, fabrics for the treatment of wounds, membranes for the development of tissues, fixed prostheses, and implants, capsules for the release of medications, among others. [2,3]. Despite their similarities with plastics of petrochemical origin, their environmental advantages, and their wide field of application, their use is hindered by their high production costs. These prevent them from being economically competitive, reaching values between 2.0 - 5.0 €/kg when the estimated cost of polymers derived from petroleum is less than 1.0 €/kg [4,5].
In the production of PHAs, large amounts of raw material are required, mainly conventional sugars such as glucose, which together with other components required for the culture medium, represent between 30-50% of the total production cost. [6-8]. This has limited the development of these bioplastics, but at the same time, has driven the search for alternative economic substrates to obtain PHA [9,10].
From this perspective, the bitter cassava of the variety Copiblanca represents an excellent alternative, because of the tuber’s high yield per hectare (30 tons / year), its tolerance to droughts and degraded soils. In addition, because it is a cassava for industrial use, it does not put at risk the food security of the populations that depend on cassava as food [11]. In Colombia, this cassava has been used in recent years as a raw material for its use as a growing medium in biotechnological processes. This flour has a high content of carbohydrates (> 80%), which are obtained after a simple enzymatic treatment and which become the carbon source for microbial cultures [12].
Currently, the most studied microorganism in the production of PHAs is the commercial bacterial strain Ralstonia eutropha H16. This type of bacteria is easy to cultivate, grows rapidly in simple sugars such as glucose, is not pathogenic for humans and due its high capacity of accumulation of this polymer (up to 90% dry weight). This has allowed its consideration as a comparative model for the search of new microbial strains with potential for PHAs production [13]. In Colombia, there have been reports of bacterial isolates producing PHAs [9,14-18], however, there is no reference to native strains having a competitive PHAs production potential in comparison to commercial strains. The present research was carried out with the purpose of contributing with alternatives for the production of these biopolymers, by selecting and evaluating the PHAs production potential of a native isolate of Colombian soil, against the referenced strain R. eutropha H16, using glucose syrup obtained by hydrolysis from cassava flour of an non-edible variety, as an alternative substrate for the crop.
2. Materials and methods
2.1. Microorganisms
Four bacterial isolates obtained in a previous study of the Biotransformation Group of the School of Microbiology of the University of Antioquia were selected. The isolates were obtained from soil samples of banana, cassava and tomato crops. The PHAs production potential was previously identified through the qualitative characterization of the polymer with two lipophilic dyes, Sudan-B black, and Nile-A red, and by gas chromatography [19]. The microorganisms were designated as HY-26, SB-34, SY-54, and ST-60. The referenced strain R. eutropha H16 was purchased through the CES University. The microorganisms were kept at -20 ° C in trypticase soy medium (TSB) with 30% glycerol for preservation [20].
2.2. Culture medium
The culture medium used was the MSM medium, (Mineral Salts Medium) already referenced and reported in relation to the growth and production of PHAs [21]. As a carbon source, a glucose-rich cassava flour hydrolyzate of the Copiblanca variety from the Urabá sub-region of Antioquia was used. The method described by Castaño et. al., which suggests the employment of the enzyme complex STARGENTM 001, was used to obtain the cassava flour hydrolyzate[12]. The other components of the culture medium were purchased through Merck Colombia S.A. The culture medium was prepared by adding the corresponding amount of each salt solution per 20 g / L of sugar (glucose), provided by the cassava flour hydrolyzate: Na2HPO4.7H2O, 6.7 g/L; KH2PO4, 1.5 g/L; (NH4)2SO4, 1.0 g/L; MgSO4.7H2O, 0.2 g/L; iron and ammonium citrate , 60 mg/L; CaCl2.2H2O, 10 mg/L; 1 mL of trace elements (0.3 g/L of H3BO3; 0.2 g/L of CoCl2.6H2O; 0.1 g/L of ZnSO4.7H2O; 30 mg/L of MnCl2.4H2O; 30 mg/L of NaMoO4.2H2O; 20 mg/L of NiCl2.6H2O; 10 mg/L of CuSO4.5H2O). The salts were prepared separately in sterile concentrated solutions.
2.3. Culture conditions
The cultures were prepared in a 500 mL Erlenmeyer flask with a gauze cap to favor the transfer of gases. It was incubated at 30 ° C with constant agitation at 150 rpm, initial pH of 7.0 and a culture volume of 100 mL. After 24 h of cultivation, a substrate pulse (cassava flour hydrolyzate) corresponding to 10 g / L of sugars was added, to ensure the carbon source excess and induce the accumulation of the polymer. The cultures were monitored by taking samples every 4 hours for 3 days, to evaluate the growth and the intracellular accumulation of the polymer. The samples were kept refrigerated at 4 ° C until analysis.
2.4. Determination of growth, quantification of biomass and kinetic parameters
The cultures were monitored by optical density (OD600nm), using a THERMO SPECTRONIC model GENESYS 10 spectrophotometer.
The quantification of biomass was performed by gravimetric method. A sample volume of 5 mL was taken for this, which was filtered with 0.45 μm cellulose nitrate filters. The filtered samples were dried in an oven at 60°C for 24 h. They were later transferred to a desiccator until reaching a constant weight. They were weighed on an analytical balance and the biomass concentration obtained was determined in g/L.
The methodology described by Godia and López, 1998 was used to determine kinetic parameters, specific growth rate (μ), duplication time (td) and yields (YX/S, YP/S, YP/X), [22].
2.5. Determination of sugars
Substrate (glucose) consumption was determined through high-performance liquid chromatography (HPLC), using an Agilent Technologies 1200 chromatograph, model 61362A, with an Aminex HPX-87H ion exchange separation column, 300 x 7.8 mm. The samples were centrifuged at 7000 rpm for 5 min and the supernatant was taken and diluted in the mobile phase. Each diluted sample was filtered through 0.2 μm regenerated cellulose filters and injected under the following running conditions: 20 μl sample volume, 0.6 mL / min flow, 35°C column temperature and 12 min runtime.
2.6. Relative accumulation of the polymer
The relative intracellular formation of the polymer was determined by means of lipophilic staining with Sudan B black colorant [23]. Briefly, the samples were centrifuged, and the pellet was resuspended in 0.9% saline, a bacterial smear was prepared on a slide and allowed to dry at room temperature. Subsequently, Sudan B black colorant was added until the sample was covered and left to rest for 15 min. The excess dye was removed with absorbent paper and decolorized with xylene (reactive grade) for 10 s. The excess solvent was removed with absorbent paper and covered with safranin solution for 10 s. The samples were washed with distilled water and allowed to dry at room temperature for later revision under a microscope. The presence of black granules inside the cells indicated the possible formation and detection of PHAs. The relative accumulation of the polymer was then estimated from average values between the ratio of the area occupied by the granule, with respect to the total area of the cell.
2.7. Extraction of the polymer
an acid digestion of the non-polymeric cellular material was performed to recover the polymer [24]. The sample was centrifuged at 5000 rpm for 12 min. The pellet was washed with distilled water and subsequently, the biomass was diluted in 200 mL of distilled water and the suspension was sterilized at 121°C and 1 atm for 30 min. The suspension was again centrifuged at the above conditions and the pellet obtained was suspended in a solution of 0.1 M H2SO4. This solution was heated at 90°C for 2 h and, after cooling to room temperature, the pH was adjusted to 10 with 5 N NaOH. Repeated washings were performed with distilled water to remove excess acid. The biomass pellets were suspended in a 2:1 solution (distilled water: 6%v/v hypochlorite), allowed to decolorize for 2 h at room temperature and again, centrifuged at the same conditions. Finally, the polymer obtained was washed with distilled water, dried in an oven (65°C) and taken to a desiccator until a constant weight was obtained.
2.8. Analysis of the polymer by FTIR
Samples of the extracted biopolymer were analyzed using the Fourier transform infrared spectroscopy (FTIR) technique, together with a Perkin-Elmer Spectrum BX infrared spectrophotometer and an ATR module from 4000 to 400 cm-1. For the identification of the characteristic functional groups of biopolymers produced by bacteria such as R. eutropha, a standard of Sigma-Aldrich brand polyhydroxobutyrate (PHB) with 99% purity was used as a control sample.
2.9. Molecular identification of the isolate
The isolate was identified by sequencing a 1400 bp product of the 16S rDNA gene amplified by PCR with the primers: 27F 5’-AGAGTTTGATCCTGGCTCAG-3’ and 1401 5’-CGGTGTGTACAAGAAGACCC- 3’ [25]. The PCR products were purified with the QIAquick PCR purification Microcentrifuge QIAGEN kit and sent for sequencing to the Human Genome Studies Center of the University of Sao Paulo (Brazil) (USP). The equipment used was the ABI 3730 DNA Analyzer (Applied Biosystems, USA). The obtained sequences were edited through the Bioedit 6.06 program looking for a consensus sequence. The identity of the consensus sequence was confirmed by comparison with the molecular databases using BLASTN GenBank and Silva high quality ribosomal RNA databases (http://www.arb-silva.de/).
All cultures were performed in triplicate and analyzed statistically with the STATGRAPHICS Centurion XVI software.
3. Results
3.1. Evaluation of the growth of bacterial isolates
Fig. 1 shows the growth curves of the strains evaluated together with the referenced strain R. eutropha H16. It was observed that ST-60 and SB-34 isolates grew in a similar way to the commercial strain in the first hours of the culture. However, after 12 h, the ST-60 isolate´s growth slowed down, reaching a maximum of 6.38 OD, while the SB-34 isolate and R. eutropha kept growing, the latter, up to the 24th h, reaching a maximum of 8.5 OD, while SB-34 continued in the exponential phase up to the 50th h of culture with 10.81 OD, showing greater growth. The isolates HY-26 and SY-54 showed a longer adaptation time, and growth was observed only after 24 h of culture. HY-26, SY-54, and ST-60 reached approximately 6 OD units at the end of the culture. Such densities, together with that obtained by R. eutropha H16, were lower than the obtained with the SB-34 isolate. Statistical analyzes showed that this difference is statistically significant (p <0.005).
3.2. Evaluation of the accumulation of PHAs granules
Several authors have used Sudan B black differential lipophilic staining as a strategy to demonstrate the presence of PHAs biopolymers in bacteria [17,18,26]. The accumulation of polymer in four isolates and the control strain R. eutropha H16 was evidenced after such staining (Fig. 2). The results showed that, although isolates HY-26, SY-54, and ST-60 accumulated high percentages of the polymer with respect to the total area of the cell, the SB-34 isolate was similar to the strain referenced in both morphology and accumulation capacity of the biopolymer, reaching estimated values of qualitative accumulation close to 90%.
3.3. Comparison of culture kinetics of SB-34 and R. eutropha H16.
The growth capacity and substrate consumption of SB-34 and R. eutropha H16 bacterial isolates were compared. The results obtained showed that in the first 24 h of culture, both microorganisms were similar in both growth kinetics and substrate consumption, and reached biomass concentrations close to 4.0 g / L and an approximate consumption of sugar 8.0 g/L (Fig. 3). After performing the substrate pulse at the 24th h, R. eutropha H16 reached the stationary phase, contrary to SB-34, which continued in the growth phase until the 50th h.
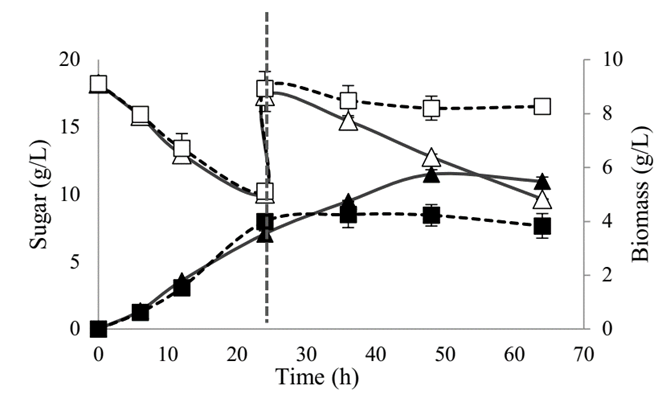
Source: The authors
Figure 3 Growth curve and glucose consumption of SB-34 and R. eutropha H16. Substrate and
biomass of SB-34;
substrate and
biomass of R. eutropha H16. (---) Substrate pulse.
It is noteworthy that, in both cultures, the substrate was not consumed totally during the total time of experimentation; the substrate consumption by SB-34 after the carbon source pulse was added, remained constant and was similar to the initial, contrary to what was evidenced with Ralstonia, which showed no consumption.
Two hypotheses could explain these results. The first one is associated with differences in the nutritional requirements of both microorganisms, since the number of available nutrients (N, P, S, Mg, etc.) for both cultures was the same and was limited from the beginning, which would indicate that the greater growth presented by this isolate, was possibly due to a lower nutritional requirement, in comparison with the reference strain. The second hypothesis is related to the oxygen requirements for respiration. As reported, in the production of PHAs from aerobic microorganisms, one of the strategies used for the culture preparation consists of using reactors with aeration and control systems that maintain the oxygen supply between 30-50% saturation of the medium; this guarantees adequate growth and substrate consumption [27]. In our trials, the cultures were performed at the Erlenmeyer level with an airhead in a ratio of 1: 5 (medium volume / air volume) and were kept in constant agitation. However, as the cell density in the cultures were increasing, these could have been limited due to higher oxygen requirements and lower availability [28,29], which could explain the fact that the substrate was not consumed in its entirety in either culture. However, the higher growth and substrate consumption evidenced by SB-34 could be proof of a greater capacity for growth under conditions of lower oxygen availability. This would represent favorable economic advantages for a subsequent scaling process since a lower supply of oxygen and nutrients would be required and therefore, the costs of PHAs production would decrease. This reduction, together with increasing growth times, would favor the process. However, additional tests and studies will be required to verify these hypotheses.
3.4. Evaluation of parameters
In order to compare the efficiency in the culture of both microorganisms, different parameters of importance were estimated (Table 1). When analyzing the kinetic parameters μ and td, the results obtained are coherent with previous reports about R. eutropha growing in sugars (glucose and fructose) as a carbon source, which indicates specific growth rates between 0.2 - 0.6 h-1 [30,31], and although no statistically significant differences are shown in the specific growth rate and generation times between SB-34 and the commercial strain, the highest growth and substrate consumption achieved by SB-34 represent an advantage for the cultivation and production of PHAs, compared to the referenced strain, when cassava flour hydrolysates are used for the culture medium as carbon source.
Table 1 Kinetic parameters calculated for the bacterial isolate SB-34 and the reference strain R. eutropha H16.
Parameter | Microorganism | ANOVA | |
---|---|---|---|
SB-34 | R. eutropha H16 | Value-P | |
X (g/L) | 5.49 ± 0.21 | 3.83 ± 0.45 | 0.006* |
P (g/L) | 3.23 ± 0.21 | 2.42 ± 0.23 | 0.015* |
td (h) | 1.36 ± 0.03 | 1.39 ± 0.05 | 0.310 |
µ (h-1) | 0.51 ± 0.01 | 0.50 ± 0.02 | 0.311 |
YX/S (g/g) | 0.38 ± 0.02 | 0.41 ± 0.05 | 0.103 |
YP/S (g/g) | 0.22 ± 0.03 | 0.26 ± 0.04 | 0.134 |
YP/X (g/g) | 0.59 ± 0.04 | 0.63 ± 0.08 | 0.655 |
Prod (g/L*h) | 0.05 ± 0.003 | 0.04 ± 0.002 | 0.013* |
µ = specific growth rate; td = cell duplication time; X = biomass; P = polymer; YX/S = biomass yield per unit of substrate; YP/S = product yield per unit of substrate; YP/X = product yield per unit of biomass; Prod = polymer productivity. * Values that show statistically significant differences.
Source: The authors
In relation to the final biomass produced, statistically significant differences were obtained between the microorganisms. The SB-34 isolate reached a biomass concentration of 5.49 ± 0.21 g/L, exceeding by 30% the one obtained by the referenced strain (3.83 ± 0.45 g/L). This aspect is of great importance and makes this isolate possess a desirable characteristic to produce biopolymers, since the formation of the PHAs is intracellular and, therefore, the production is directly related to the biomass obtained.
In terms of polymer production (P), the results show that SB-34 produced significantly more PHAs compared to R. eutropha (3.23 ± 0.21 g/L and 2.42 ± 0.23 g/L, respectively). These results are consistent with the biomass obtained with both microorganisms, since, as explained above, there is direct dependence between the amount of polymer produced with the biomass formed. In this sense, strain SB-34 has a high potential for the production of this biopolymer.
In yields, no statistically significant differences were observed. The values of YX/S, YP/S, and YP/X obtained with both microorganisms were similar, which could indicate that they have the same kinetic behavior regarding substrate conversion efficiency in the formation of biomass and polymers, reaching about 60% of the polymer content relative to the dry weight.
Although there are no differences in the yields obtained in this study, the greater potential of the SB-34 isolate for PHAs production, is an important aspect worth highlighting. Although higher productivities are reported in the literature ((3 g / Lh) [11,32], a 25% increase in polymer productivity was obtained under the conditions evaluated, compared to that achieved with the commercial strain.
3.5. Relative accumulation of the polymer
Fig. 4 compares the morphology and intracellular accumulation of PHAs granules for both microorganisms. It was observed that the accumulation was progressive from the beginning of the culture, increasing in size in accordance with the increase in cell size.
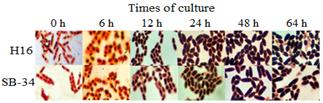
Source: The authors
Figure 4 Intracellular accumulation of PHAs granules in SB-34 and R. eutropha H16 over time. The presence of the polymer was evaluated by staining with Sudan B black at time intervals for 64 hours. The internal areas stained black correspond to presumptive PHAs granules.
An atypical formation of the polymer was observed in both microorganisms, since, as reported, the formation of the polymer is mainly associated with the stationary phase of growth [33-36] and the experimental results showed that its formation occurs from the beginning of the culture. From the physiological point of view, this could be related to the oxygen limitation to which the culture could have been subjected during the time of experimentation. It is known that, in facultative anaerobic organisms, as it is the case of R. eutropha, the lack or limitation of oxygen generates an accumulation of NADH in the cytoplasm, which inhibits the enzymes citrate synthase and isocitrate dehydrogenase, preventing acetyl-CoA from entering the tricarboxylic acid cycle. [37]. This causes the metabolism of the microorganism to be directed towards alternative routes to maintain the redox balance NADH / NAD+, such as anaerobic respiration, fermentation or any other route that allows the maintenance of this balance, as it is the case of the route of formation of PHAs. And although in this route the electron donor is NADPH, it has been reported that in R. eutropha, the enzyme acetoacetyl-CoA reductase involved in this PHAs synthesis route, which uses NADPH as an electron donor in the reduction of acetoacetyl-CoA to 3-hydroxybutyryl-CoA, is not dependent on NADPH and can use NADH to carry out this reduction. [38,39]. This can induce the early formation of the polymer in media with oxygen limitation, thus favoring the deviation of acetyl-CoA towards the PHAs synthesis pathway. The above could not only indicate the presence of this same non-specific NADPH enzyme in the SB-34 isolate but also that, under conditions of oxygen limitation, the production of PHAs by this bacterium would be favored.
3.6. Analysis of the polymer by FTIR
When comparing the FTIR spectra of the polymers obtained with SB-34 and with R. eutropha against the standard sample of PHB (Fig. 5), a correlation of 99.3% was observed between the absorption bands corresponding to the characteristic functional groups of the PHB. Peaks of the functional groups C = O and CH3, respectively, are formed in the region 1740 cm-1 and 2970 cm-1 of the spectrum, which is characteristic of the polyhydroxybutyrate monomer (PHB) [40, 41].
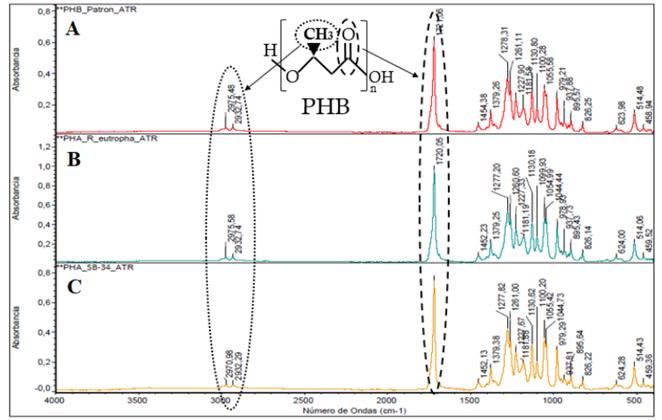
Source: The authors
Figure 5 Fourier transform infrared (FTIR) spectra of the polymers. Sample pattern of PHB (A); polymer obtained with R. eutropha H16 (B) and with SB-34 (C). The bands corresponding to the functional groups C = O (1740 cm-1) and CH3 (2970 cm-1) characteristic of the polyhydroxybutyrate monomer (PHB) are indicated.
According to the reports, the PHB-type polymer is highly crystalline (> 50%) and has an approximate glass transition and fusion temperature of 180°C and 4°C, respectively. It has some mechanical properties comparable to degradable synthetic polyesters such as PLA [42, 43]. Additionally, this type of biopolymer is an attractive material for tissue engineering, due to its inherent biocompatibility. It has been shown to improve the proliferation of different cell types and since the PHB is susceptible to various processing methods, it can be mixed with another type of biocompatible molecules such as the mineral hydroxyapatite to create films, fibrous mats or non-crystalline chains that can be adapted to specific uses. [44-46].
3.7. Molecular identification of the SB-34 isolate
The sequence analysis indicated that the SB-34 isolate possesses a 96% identity with bacteria of the genus Burkolderia sp. This genus belongs to the phylum β-proteobacteria that are abundant in the soil and in aquatic environments, and some species are characterized by being phytopathogenic. The first work that describes the production of PHAs by bacteria of this genus w supports the production capacity of PHB and PHB-co-HV (copolymer) from fructose and propionic acid as carbon sources [21], however an ability to use a wide variety of carbon sources to produce these types of polymers has also been reported [47]. Additionally, this finding correlates with the metabolic capacity described in Burkholderia and Ralstonia in PHAs synthesis, since the PHAs synthase enzyme of these microorganisms -PHAs synthase type I- has an affinity for 3-hydroxyalkanoate-CoA short chain thioesters, and the main type of polymer produced is PHB [48-50].
4. Conclusions
The methodology applied allowed the selection and identification of a native bacterial isolate, with the capacity to produce PHAs, with better physiological characteristics in terms of cell growth, substrate consumption, biopolymer production, and productivity, than the reference strain R. eutropha H16.
This makes of Burkholderia sp. an isolate with potential for the production of PHAs, in alternative media based on cassava flour hydrolysates as a substrate and with lower nutritional and oxygen requirements.
In addition, the polymer produced by the SB-34 isolate corresponds to a PHB, whose physical-chemical properties and potential use in the industry are worth noting.