1. Introduction
In 2014, Colombia planted 230303 hectares of sugarcane and harvested 24 million tons of cane [1]. Crop residues are estimated at approximately 50% and consist mainly of leaves and top cane [2], which decompose in the field. Half of them could be used in various industrial alternatives (cogeneration, biofuels) and the other half to restore the organic layer of the soil. But the principal application could be its valorization towards the production of second generation ethanol, integrated to the first generation ethanol. Very few studies have been done on this material. The cost of producing alcohol from lignocellulosic material is relatively high, and the focus is now on the low yields and high costs of the hydrolysis process. Different investigations have been carried out to identify the economic and technological limitations that are generated in the use of enzymes for the hydrolysis of agricultural residues [3-6]). Efforts have been made to improve the hydrolysis of lignocellulosic materials, among them pretreatments, the search for enzymes based on mixtures of enzymatic activities and the loading of enzymes; which remains a challenge since the structural and chemical characteristics make the enzymatic hydrolysis [7,8]. The saccharification process of lignocellulosic material can be accomplished by a variety of pretreatments (thermal, acidic, alkaline and enzymatic). Enzymatic pretreatment is one of the methods that offers several advantages, such as high specificity, milder reaction conditions, high yield and less formation of undesirable products [9-11]. Many pretreatment processes have been suggested for the efficient saccharification of these materials [12-17] to separate the recalcitrant material from the lignocellulosic matrix. At a cost of US $ 0.38 / 100 FPU, generating cellulases and hemicellulases by solid state fermentation, according to NREL (National Renewable Energy Laboratory), is lower than the production by in situ submerged culture. These costs can reach up to 20% of the costs of ethanol production. On the one hand, the costs of commercial enzymes, which is around US $ 16/100 FPU, is prohibitive for this process [18]. On the other hand, finding the mixture of enzymatic activities that produces an enzyme capable of converting the components of the lignocellulosic materials into fermentable sugars at a suitable enzyme/substrate ratio that allows an economically viable hydrolysis process, is a challenge for the industry.
For efficient hydrolysis of sugarcane harvest residues to simple sugars, it is necessary to develop a synergistic combination of enzymes capable of hydrolysing cellulose and hemicellulose [19]. For this reason, this article aims to show the role that pretreatment (changes in the structure of the material) takes in the enzymatic hydrolysis of sugarcane harvest residues, mainly using the leaves and top canes, as a fundamental step in obtaining simple sugars (of six and five carbons) as a basis for the production of bioethanol.
2. Methodology
2.1. Enzymatic activity in commercial enzymes
The expressed cellulolytic activities were measured as FPUs (filter paper units) [20]. The hemicellulase activity was the sum of the xylanase [21], galactosidase, mananase, rhamnosidase [22] activities, based on 11 commercial enzymes. From the enzymatic spectrum found in each commercial enzyme, a combination was developed, with the help of Microsoft Excel® Solver software, to prepare five cocktails with different cellulolytic activities. These five cocktails were coded E1, E2, E3, E4, and E5. The effect of each enzymatic cocktail on the different substrates was evaluated with the saccharification index [23] and the Global Hydrolysis Index (GHI) [24].
2.2. Preparation of substrates
The leaves and top cane of sugarcane crop residues used in this study came from varieties CC 8475, CC 8592 and were supplied by CENICAÑA. These residues were used a few days after being collected. The first substrate (S1) corresponded to the control, which was not subjected to any treatment. Substrates S2 to S8 were pretreated as described in Table 1. The moisture content was determined until weight was constant at 105°C, Kappa Number by Tappi Standard T 236-cm-8 and cellulose, hemicellulose, lignin, extractive and ash content were determined by the Van Soest method [25].
2.3. Enzyme evaluation
From the mixtures of enzymatic activities obtained from the commercial enzymes, reaction progress curves were collected at different enzyme/substrate (E/S) ratios (0.05, 0.1, 0.2, and 0.3) for all pretreated substrates. The saccharification was carried out in acetate buffer solutions at pH 4.8 with a solid/liquid ratio of 6:100 at a temperature of 40 °C. Samples were taken at reaction times of 0, 2, 6, 9, 24 and 28 hours, from which reducing sugars were determined by the DNS method [30]. To have a more significant spectrum, which allows visualizing the interactions of the enzymatic mixtures and the different substrates, an analysis of the interactions between enzymes and substrates performed through an analysis of fuzzy systems, using the diffuse logic tool of MATLAB® R20009 software. In the development of this research, the following were assigned as input variables to the diffuse system: percentage cellulose composition (35 - 82%), hemicellulose (10-38%), lignin content (0.5 -12%) and enzyme activities such as filter paper units (1-40 FPU/ml), hemicellulase activity (10-140 IU/ml) and beta-glucosidase activity (100-2500 pNPG U/ml). The Global Hydrolysis Index (GHI), in ranging between 0 and 112, was established as the output variable.
3. Results and discussion
The cellulolytic and hemicellulosic activities of commercial enzymes are shown in Figs. 1 and 2. Accelerase® 1500, accelerate® XC, and celluclast ® enzymes have high values (FPU / ml). It was also observed that enzymes described as cellulolytic have high levels of hemicellulosic activity.
The composition of the different substrates used in the evaluation of the enzymes is shown in Table 2. Enzymatic cocktails showed cellulase activity between 0.073 FPU / ml and 37 FPU / ml, hemicellulase activity from 14 IU / ml to 129.4 IU / ml and a β-glucosidase activity of 183 pNPG / ml to 2498 pNPG / ml. These results allowed us to obtain enzyme cocktails with a wide range of enzymatic activities (See Table 3).
Table 2.

Compositional analysis (percentage) of the substrates of pre-treated materials from sugarcane harvest residues.
Source: The Authors.
Table 3. Spectrum of activities of enzyme mixtures obtained through mixtures of commercial enzymes

Hemicellulase* Global UI/ml: The sum of xylanase, galactosidase, mannanase and rhamnosidase activities was designated global hemicellulase activity; (2) Cellulase FPU/ml; (3) Endoglucanase (CMC/ml); (4) Exoglucanase UI/ml; (5) β Glucosidase (pNPG U/ml; (6) Xylanase (UI/m); (7) Galactosidase (UI/ml); (8) Manananase (UI/ml); and (9) Ramnosidase (UI/ml).
Source: The Authors.
According to Patel, et al., 2015 and Silverstein, et al., 2007 [31-32], the composition of enzymes is a crucial factor that affects the successful application of enzymes in bio-refineries. Effective bioconversion of lignocellulosic material into fermentable sugars requires a balanced composition of cellulolytic and hemicellulolytic enzymes.
It is a well-known fact that the production of cellulases and hemicellulases is significantly affected by culture conditions as well as the inducing substrate. Researchers suggested that the set of enzymes produced by microorganisms during growth of lignocellulosic biomass is dependent on the substrate used. In addition, saccharification efficiency of enzyme complexes is at a maximum when these enzymes are produced on the same lignocellulosic materials in fermentation [33-35].
The global hydrolysis Index (GHI) shows the interaction of the enzyme mixture and the production of fermentable sugars. Fig. 3, shows that the E5 enzyme has its highest GHI when substrates S3, S4, S5, S6, S7 and S8 are used. Otherwise, the maximum occurred when using substrates high in hemicellulose and lignin content (S1, S2). It is possible that this difference in GHI is due to the presence of recalcitrant material that did not separate during pretreatment.
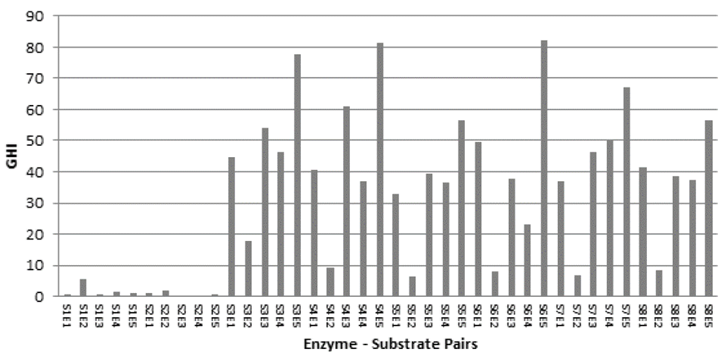
Source: The Authors.
Figure 3 Global Hydrolysis Index (GHI) for the different enzyme-substrate pairs.
Enzyme preparation E2 has low activity in filter paper units (FPU / ml) and relatively low GHIs. Otherwise, enzymes with average FPU / ml values and with balanced cellulose and hemicellulase activities (E3, E4, and E5) produced a higher IGH. The compositions of designed enzyme mixtures resulting from this study depended on a wide variety of factors such as utilized enzymes, type of biomass and type of pretreatment, and highlighting the importance of customizing enzyme mixtures for individual processes [5].
To complement, it was necessary to implement a procedure to determine pairs of enzyme-substrate relationships with similar characteristics, through the conglomeration method of the nearest neighbour, taking the metric distance (square Euclidean) as a parameter.
Fig. 4 shows three conglomerates, in one of which the E5 enzyme mixture is grouped with substrates S3, S4, and S6, which are the enzyme-substrate pairs that have higher GHI. A second group is represented mainly by substrates that have high Kappa numbers (high lignin content), such as S1 and S2, which have a low GHI. A third group of intermediate GHIs mainly involves the E1 enzyme mixture with all the substrates, except S1 and S2. The E1 enzyme mixture had the highest activity expressed in FPU / ml, and the E2 enzyme mixture had the lowest value of filter FPU/ml found in the group with the lowest GHI. These results indicate the importance of pretreatment in the enzymatic hydrolysis of lignocellulosic material (leaves and the top cane of sugarcane harvest residues).
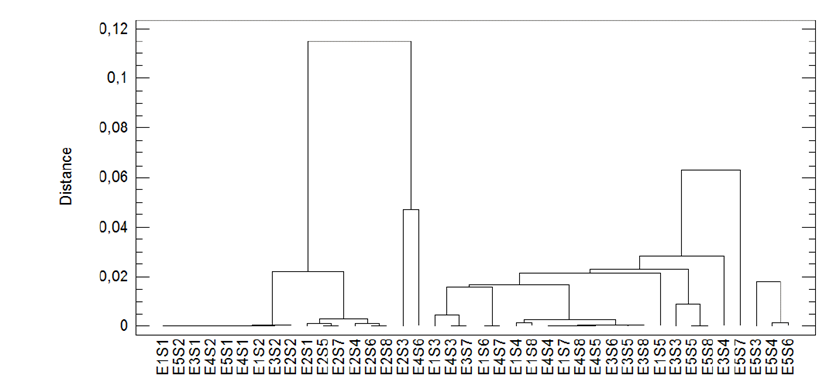
Source: The Authors.
Figure 4. Dendrogram of the global hydrolysis index of the closest distance for different enzyme-substrate pairs.
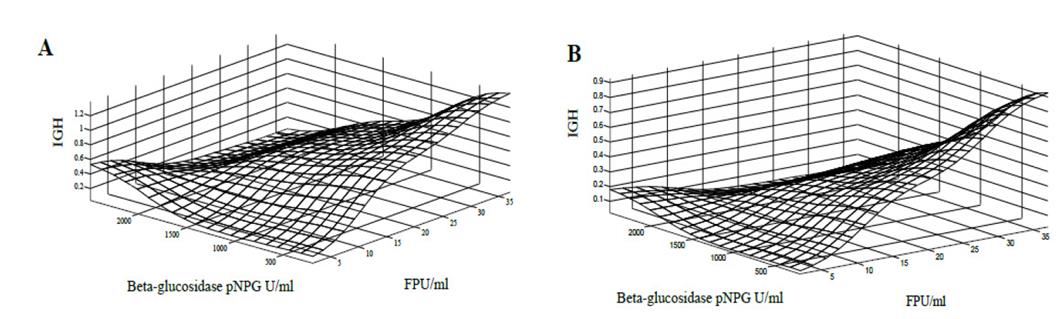
Source: The Authors.
Figure 5. Response surface of the GHI against cellulitic activity (FPU / ml) and beta-glucosidase activity (pNPG U / ml), for substrates S1 (A) and S2 (B) with a hemicellulase activity of 60 IU / ml.
Behaviour of the GHI for substrates S3, S5, S6 and S7 was characterized by different types of pretreatment and originating substrates with different levels of cellulose, hemicellulose and lignin content for enzymatic hydrolysis (Fig. 6). In general, these substrates, after enzymatic hydrolysis, produced a high GHI. Substrates S3 and S6, which contain low residual lignin content, presented with a higher GHI. In all these pretreated substrates, a response surface ceiling is observed for beta-glucosidase activity values less than 500 pNPG / ml and filter paper units greater than 20 FPU / ml.
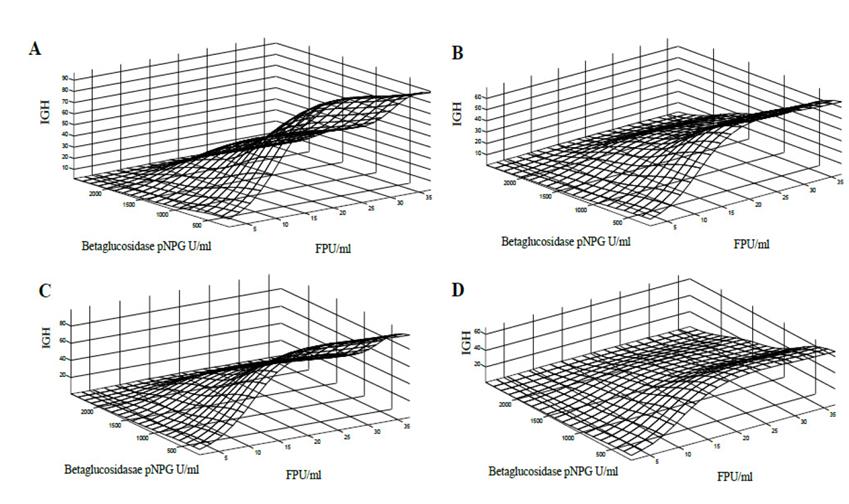
Source: The Authors.
Figure 6. Response surface of the GHI against the cellulitic activity FPU / ml and beta-glucosidase activity pNPG U / ml, for the substrate S3 (A), S5 (B), S6 (C) and S8 (D) with a hemicellulase activity 60 IU / ml.
These results are an indicator of the synergism presented by the different activities of the enzymes, and show that it is not an excess of activity which determines the hydrolysis of the lignocellulosic material [36]. In addition, it is essential to observe how the lignin content and the type of pretreatment influence the activities of the enzymatic mixtures, favouring the pretreated substrates with lower lignin content. These conclusions are supported by research carried out by [37-39]. They also reinforce a claim regarding the effect of lignin content and pretreatment on the hydrolysis rate, confirming that the aim of the pretreatment of lignocellulosic materials is to try to disorganize the crystalline structure of macro and micro fibres to release the polymeric chains of cellulose and hemicellulose, modifying the pores in the material to allow the enzymes to penetrate inside the fibres and make the enzymatic hydrolysis easier [39-41]. It is essential to visualize that the response surfaces reach the maximum high values of cellulose activity (FPU / ml), indicating that excess of this activity does not improve the hydrolysis. The same occurs with beta-glucosidase activity (pNPG U / ml). For substrates S3, S4, S5, S6, S7 and S8, the overall hydrolysis index is favourable for beta-glucosidase activity of less than 500 pNPG U / ml and cellulolytic activity between 20 and 30 FPU / ml. It is necessary to clarify that these simulations are specific for residues of the sugarcane harvest (leaves and top cane).
Conclusions
Pre-treatment is an essential factor that must be taken into account in the hydrolytic processes of lignocellulosic materials (sugarcane residues). Depending on the type of pretreatment, the attack of the enzymes on the lignocellulosic matrix can be facilitated or hindered. The interaction of physical and chemical pretreatments in lignocellulosic materials produces changes in the structure of the lignocellulosic matrix, promoting enzymatic hydrolysis. In this study, the use of a pretreatment with enzymes was not efficient. The lignin content has an adverse effect on the hydrolysis of the substrates for different enzymes, which leads to the conclusion that lignin intervenes as an inhibitor in the enzymatic hydrolysis of sugarcane residues. The simulation in fuzzy logic presented regions of maximum global hydrolysis index for sugarcane harvest residues, which are between 20-30 FPU / ml, and values lower than 500 pNPG U / ml for beta-glucosidase activity and between 50 and 70 IU / ml for hemicellulase activity. These results indicate the importance of beta-glucosidase in the enzymatic hydrolysis of lignocellulosic substrates and that large amounts of cellulolytic enzymes are not necessary. Enzymes that have an appropriate distribution of enzymatic activities according to their composition have a synergistic action of enzymatic activities that is reflected in a better rate of hydrolysis, as is the case of the enzymatic mixture E5 and, to a certain extent, E1 and E2. The highest global index of hydrolysis was revealed with the E5-S6 enzyme-substrate pair, in which the substrate was pretreated with an organo-solvent process and the enzyme mixture had an FPU content of 27.53.