1. Introduction
To achieve large-scale, low-cost biotech production of second-generation bioethanol, it is necessary to look for substrates that are economical, abundant, easy to collect and transport, do not compete with food safety policies, are environmentally safe and are appealing for use in the energy industry. One of the substrates that meets these characteristics are lignocellulosic materials that come from sugarcane, which is a large-scale crop primarily harvested in Brazil. In Colombia, mechanical green harvesting produces between 35 and 93 tons/ha of waste, manual burning produces between 19 and 59 tons/ha, and 81% of this residue is made up of leaves and tops. According to these data, an average of 9 million tons of this material, a resource rich in fermentable sugars that has not been valued, can be generated per year [1]. In general, the residues of the three varieties of sugarcane that are most commonly cultivated in the country (CC 8592, CC 8475, V 7151) contain an average of 42% cellulose, 26% hemicellulose, 20% lignin and 3% ash [2,3]. From these polysaccharides, hemicelluloses have recently been extracted and characterized to find new applications. They have been used for synthesis of cationic polymers, hydrogels, ester derivatives and thermoplastics; for production of the sweetener xylitol and furfural [4]; and in applications such as adhesives, thickeners, stabilizers, film-formers, emulsifiers and even decorative paints [5].
Hemicellulose is operationally defined as the cell wall polysaccharides of plants that is not soluble in hot water or obtainable via chelating agents but is solubilized by alkalis in aqueous media [6]. Chemically, it represents a class of heterogeneous polymers primarily containing pentoses (β-D-xylose, α-L-arabinose), hexoses (β-D-mannose, β-D-glucose, α-D-galactose) and/or uronic acids (α-D-glucuronic acid, α-D-4-O-methylgalacturonic acid and α-D-galacturonic acid) [7]. These monomeric units are rearranged in other more complex forms that depend on the phylogeny of the plant, including glucans, xyloglucans, xylans, arabinoxylans, mannans, and glucogalactomannans with different degrees of substitution. Grasses of the Poaceae family, such as sugarcane, have a main (1→4)-β-D-xylopyranosyl chain with different branching patterns consisting of lateral mono-, di-, and trisaccharide chains composed of xylopyranosyl, arabinophuranosyl and/or galactopyranosyl units [8]. The hemicelluloses extracted from sugarcane bagasse with 0.5% H2O2 at pH 11.5 are primarily composed of a main xylose waste chain with links of the β-(1→4) type and with branches mainly comprising arabinofuranosyl and 4-O-methylglucopyranosyl units [9].
The process of hemicellulose decomposition to its monomeric form has not yet reached a commercially attractive level, in part because of the difficulty in extracting the hemicellulose from the lignin network of the plant wall due to the strong ester and ether bonds between lignin and carbohydrates, the numerous hydrogen bonds between the polysaccharides [10] and the difficulty of co-fermenting sugars of five and six carbons with conventional microorganisms.
Currently, the information on sugarcane hemicelluloses as a substrate for biofuel generation is limited to the polymers present in bagasse, to such a degree that information on other potentially useful parts of the plant, such as the leaves and tops, is insufficient [2]. Prior to this work, a study was carried out using different extraction methods to separate hemicelluloses from sugarcane residues. It was found that after eliminating chlorophyll, waxes, pectins and other compounds soluble in dilute acid, the application of chlorite with NaBH4 managed to recover between 0.20 and 0.41 g hemicelluloses/g dry residue in the three varieties studied [3, 11]. Because the structure of hemicellulose often varies with the methodologies used for isolation [12], it is necessary to characterize them. For this reason, this work focused on determination of the structure of hemicellulose extracted from the leaves and tops of three varieties (CC 8475, CC 8592 and V 7151) of the sugarcane Saccharum officinarum L. most frequently cultivated in Colombia and on determination of their neutral sugar content. This is in virtue of deciding on the most appropriate processes it must be submitted to for commercial use.
2. Materials and methods
2.1. Samples
Samples of crop residues (leaves and tops) from the sugarcane varieties CC 8475, CC 8592 (CC: Cenicaña-Colombia) and V 7151 (V: Venezuelan variety) were collected from the soil after mechanical green harvesting. The residues of the CC 8592 and V 7151 varieties were collected few days after harvesting of the sugarcane, while the residues of the CC 8475 variety were collected 30 days after harvesting. Each sample was mechanically homogenized according to variety, washed with distilled water at 30 °C, dried in an oven at 45 °C to a constant weight, ground and sieved. Characterization was performed with residues that had a particle size ranging between +20 (0.85 mm) and -30 (0.6 mm).
2.2. Extraction of hemicelluloses
For extraction of hemicelluloses, the procedure developed by Höije, et al. [13] and modified by González-Córdoba A. [11] was used. Total removal of liposoluble compounds, such as waxes and pigments from the cell wall, was carried out on each of the residues of the three sugarcane varieties (10 g) with a chloroform/methanol mixture (2:1 v/v) in a Soxhlet extractor at a temperature of 65 °C for 6 h until the solution became colorless. After the liposoluble components were extracted from the insoluble residue, the pectins were removed. This extraction was performed with a 0.05 M HCl solution (25 mL/1 g sample) in a Soxhlet extractor at room temperature. The solution was recirculated with a peristaltic pump. Subsequently, the dewaxed sample was delignified with a solution of NaClO2 (3.52 g/10 g sample, dissolved in 200 mL of solution) at pH 4 (adjusted with glacial acetic acid) for 2 h at 75 ºC with constant agitation. The sample was then treated with 18% NaOH and 0.26 M NaBH4 for 18 h at room temperature, followed by final extraction with 24% KOH and 0.26 M NaBH4 to extract the hemicelluloses.
2.3. Purification of hemicelluloses
Each alkaline solution obtained from the previous stage was slowly brought to a pH between 5.5-6.5 using 6 M HCl. The hemicelluloses were precipitated via evaporation concentration to obtain hemicellulose A and with ethanol to obtain hemicellulose B. For that purpose, once neutralized, the solutions were concentrated by rotary evaporation at 45 ºC to a volume between 100 and 300 mL, after which the concentrated mixtures were filtered. The filtered solids were washed several times with 0.05% HCl and allowed to dry to constant weight. After the collected hemicellulose fractions were weighed, moisture was determined according to the NREL/TP-510-42621.34 norm protocol, and the fractions were macerated and stored in covered test tubes for further analysis.
Then, the supernatant solution left over from the previous stage was rotary evaporated at 40 ºC to a volume of approximately 30 mL. Once this volume was reached, three to four volumes of 96% ethanol were added to each solution, and hemicellulose B was precipitated along with the remaining salt. This process was carried out several times until the ethanol was colorless to avoid contamination of the hemicellulose precipitate with lignin, which was dissolved in the solvent. Next, the precipitate was redissolved by heating in deionized water. The salts in this solution were removed via dialysis using sequential tubing of two different diameters: first, Spectra/Por 5 made of regenerated cellulose with a molecular weight limit (MWCO) from 12000 to 14000 Daltons, a flat width of 65 mm and a diameter of 41 mm; and second, Spectra/Por 1 with a MWCO from 6000 to 8000 Daltons, a flat width of 40 mm and a diameter of 25 mm. The salt was collected in 2-4 L of deionized water (18.2 MΩ) (Bamstead EASY deionizer) for a period of three days [13]. After dialysis, the hemicellulose solutions were dried to constant weight at a temperature of 45 °C. All hemicellulosic solids obtained immediately after being weighed were measured for moisture according to the NREL/TP-510-42621.34 protocol. They were then macerated and stored in test tubes with lids for further analysis.
2.4. FTIR analysis
Fourier transform infrared (FTIR) spectra of the extracted fractions of hemicellulose (soluble and ethanol precipitated) were obtained with a Shimadzu Affinity IR spectrophotometer in a range of 400-4000 cm-1 and with a resolution of 2 nm using a KBr disk containing a 1% finely ground sample.
2.5. 1 H and 13 C NMR analyses
1H and 13C NMR spectra of the hemicellulosic samples were recorded on a Bruker 400 NMR spectrometer UltraShield™ at 400.02 and 100.60 MHz after 15000 scans, respectively. For the preparation, 200 mg of sample was dissolved in 1.0 mL of DMSO-d6. Tetramethylsilane (TMS) was used as the internal standard. The analysis temperature was 297 K.
2.6. Thermal analysis
Thermogravimetric analysis (TGA) and analysis of hemicellulose samples via differential scanning calorimetry (DSC) were performed on a simultaneous SDT Q600 V20.5 Build 15 analyzer. Each sample was heated from room temperature to 500 °C at a rate of 2 °C/min. The analysis was performed under an inert atmosphere with a nitrogen flow of 100 cm3/min. A sample of 100 mg was weighed. TGA measured mass differences, while DSC detected energy differences. The thermograms provided information regarding the decomposition mechanisms of the polymer preparations, and the horizontal regions of the preparations corresponded to the temperature intervals in which the indicated compounds are stable.
2.7. HPLC analysis of the isolated hemicelluloses
Samples of the sugarcane residues and the hemicelluloses isolated from them were analyzed for xylose, arabinose, mannose, galactose and glucose according to the methodology proposed by the NREL Chemical Analysis and Testing Standard Procedures (TP-510-42618, 21). The samples were treated with H2SO4 for 1 h at 30 °C in a water bath, diluted up to 4% with deionized water and autoclaved at 121 °C for 1 hour. After each sample was cooled, it was neutralized with CaCO3, and centrifuged at 6,000 rpm for 15 minutes, and the supernatant was filtered through GV Durapore PVDF filters with 0.22-µm pores and a diameter of 47 mm. The monomeric sugars in the samples and standards were quantitatively determined using an HPLC system (Shimadzu, Prominence LC-20AD UFLC) equipped with a Bio-Rad Aminex HPX-87P column, a Shimadzu RID-10A refractive index detector and a Prominence SIL-20A HT UFLC autosampler. Water was used as the mobile phase at a flow rate of 0.6 mL/min and a temperature of 80 °C for 25 minutes. The sugar concentration was calculated using the LC solution software. The same H2SO4 hydrolysis procedure described above was applied to the standards to correct for the amount of sugars in the samples that could be converted to furfural. The analyses were performed in triplicate.
3. Results and discussion
3.1. FTIR spectra
3.1.1. Compounds extracted with hydrochloric acid
The amount of material extracted from each variety with hydrochloric acid was between 1 and 5% of the total residue. Data from FTIR spectroscopy analysis indicated that 0.05 M HCl extracted most of the pectin and some polysaccharides from the lateral chains grafted to pectin (Fig. 1). A shoulder between 3000 and 3400 cm-1 was observed corresponding to the presence of the hydroxyl group (OH-) of carbohydrates and absorbed water, which gives a strong signal at 1652 cm-1 [14]. The 2921 cm-1 band is a C-H stretch vibration of the methyl group [15]. A 1731 cm-1 band, shaped like a small shoulder, was present in the spectra of samples taken from the three varieties and represents the acetyl and uronic groups and the ferulic esters of the polysaccharides [9]. The presence of this band indicates that there was no rupture of the ester bonds or of the acetyl and uronic groups due to the gentle extraction conditions. The presence of a very small fraction of solubilized and precipitated lignin together with pectin was more noticeable in the CC 8592 variety and was recognized by a small peak that appeared at 1521 cm-1 that indicates the lignin-hemicellulose association [4]. Similarly, small peaks between 1248 and 1384 cm-1 corresponding to the C-H and C-O deformation bands of the hemicellulose were observed for the extracts from the three varieties.
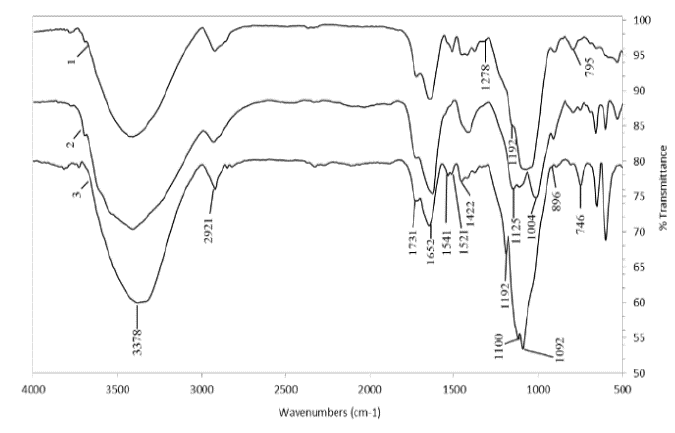
Source: The Authors.
Figure 1. FTIR spectra of pectins isolated with 0.05 M HCl solution. CC 8592 (1), CC 8475 (2) and V 7151 (3).
The stress signal of the C=O group at 1422 cm-1 is characteristic of uronic acid. The shoulder that appeared in the spectra in the region between 1200 and 850 cm-1 (Fig. 1) is characteristic of a large number of pectic compounds, including their side chains [16]. Specifically, a band for pectic substances was present between 1100 and 1015 cm-1 for extracts from the residues of the three varieties. In the CC 8475 variety, the pectin band was observed at 1004 cm-1, while in the CC 8592 and V 7151 varieties, a very strong band at 1100 cm-1 was observed, which is consistent with the results obtained by Kačuráková et al. [17]. The band at 896 cm-1 found in the spectra of the three varieties corresponds to the frequency of the C-1 group or ring frequency, which is characteristic of β-glucosidic bonds between sugar units [8,18].
3.1.2. Hemicelluloses A and B extracted with NaOH and KOH
The material extracted with NaOH and KOH from the residues of the three varieties and that was precipitated after being concentrated via rotary evaporation was given the name hemicellulose A. Hemicellulose B was extracted from the same material by subsequent precipitation with ethanol. FTIR analysis of these hemicelluloses (Figs. 2-5) showed that they have the same shoulder between 3435 cm-1 and 3450 cm-1 corresponding to the OH- group. The stress vibrations of C-H gave a signal between 2919-2926 cm-1, while those of deformation were found at 1450-1455, 1374-1424 and 1247-1253 cm-1 [19]. These signs of deformation were more intense in hemicellulose B than in hemicellulose A.
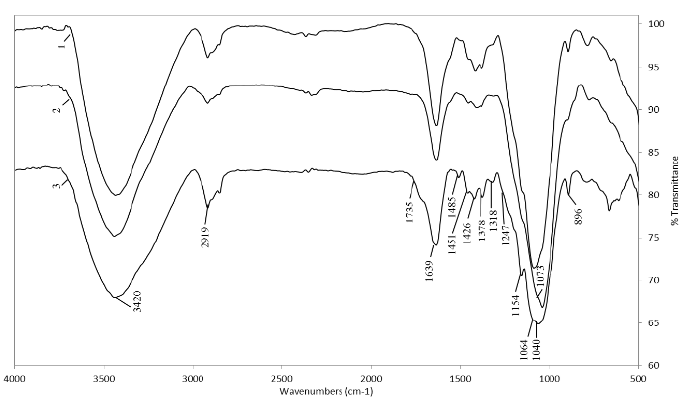
Source: The Authors.
Figure 2. FTIR spectra of hemicellulose A isolated with 18% NaOH solution containing 0.26 M NaBH4. CC 8592 (1), CC 8475 (2) and V 7151 (3).
In all the spectra, the water absorbed in the hemicelluloses presented a band between 1631 and 1642 cm-1. The shoulder between 1720 and 1745 cm-1 was very small in most extracts, indicating a weak presence of acetyl and uronic groups, typical of pectins [9], which are characterized by the stretching vibration of the C=O bond in the methyl esterified carboxyl group or in the protonated carboxylic acid [20].
Each type of hemicellulosic polysaccharide has maximum band peaks in the region between 1200 and 1000 cm-1 [17,21]. However, no sharp bands were observed in this region, which made interpretation difficult, possibly because vibrations of the ring overlap with vibrations of the C-OH side groups and of the C-O-C glycosidic bonds of the different sugars. The anomeric region between 955 and 800 cm-1 was also complicated due to overlap of the bands but can provide additional information supplementary to the region between 1200 and 1000 cm-1. In most of the spectra, there was a well-defined sharp band between 896 and 899 cm-1, which is characteristic of the β-glycosidic links between sugar units [17].
A pattern of similar bands was observed for the spectra of hemicelluloses A (Figs. 2 and. 3) and B (Figs. 4 and 5) extracted with NaOH and KOH. However, some variations in the composition of the sugars in the three varieties were perceived.
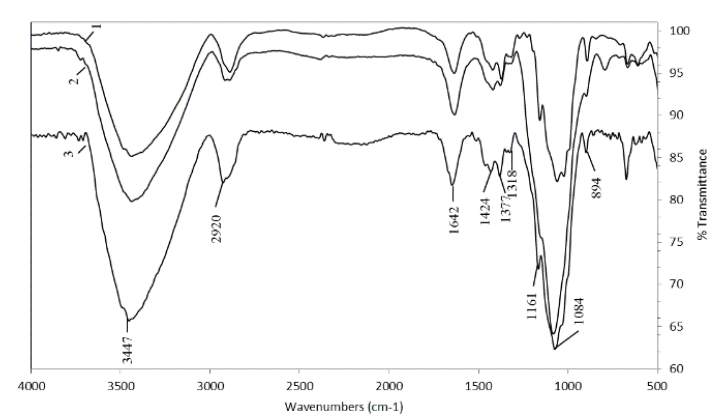
Source: The authors.
Figure 3. FTIR spectra of hemicellulose A isolated with 24% KOH solution containing 0.26 M NaBH4. CC 8592 (1), CC 8475 (2) and V 7151 (3).
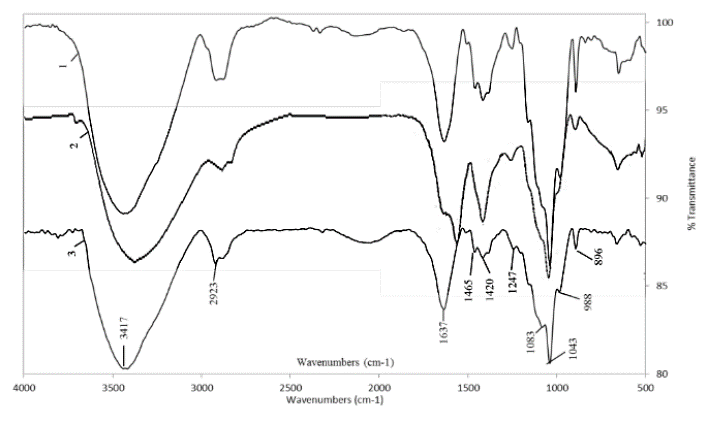
Source: The Authors.
Figure 4. FTIR spectra of hemicellulose B isolated with 18% NaOH solution containing 0.26 M NaBH4. CC 8592 (1), CC 8475 (2) and V 7151 (3).
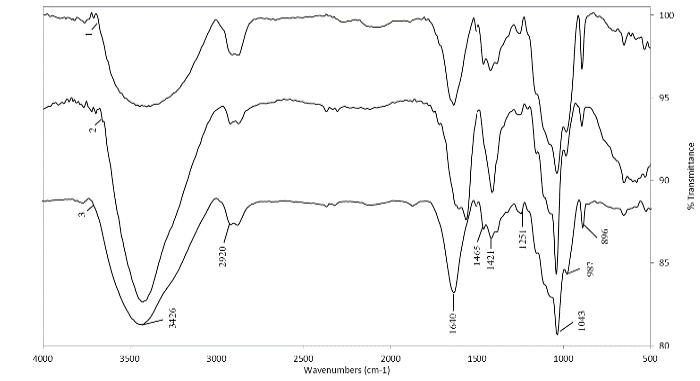
Source: The Authors.
Figure 5. FTIR spectra of hemicellulose B isolated with 24% KOH solution containing 0.26 M NaBH4. CC 8592 (1), CC 8475 (2) and V 7151 (3).
As shown in Fig. 2, in hemicellulose A extracted from the three varieties of sugarcane with NaOH, a 1064 cm-1 band appeared that was assigned to the mannose units of glucomannans [17]. The band at approximately 1044 cm-1 assigned to the stretching vibration of the C-O and C-C bonds and to the contributions of the glycosidic bond (C-O-C) in the hemicelluloses is a typical signal for xylans [21]. The sharp band near 898 cm-1 corresponds to the frequency of a C1 group or ring, which is attributed to the glycosidic β-(1→4) link between the units of xylose and hemicelluloses.
In addition, the presence of lateral arabinogalactan chains was observed based on the small shoulders found at 1154 and 1073 cm-1 [17]. The absorption at 1247 cm-1 is due to some acetyl groups that remained attached to the hemicellulose, particularly in the hemicellulose A fraction [22]. In hemicellulose A extracted with KOH (Fig. 3), the presence of arabinogalactans and glucomannans was observed, primarily based on the bands found at 1060-1079 cm-1 and 1144-1157-1162 cm-1. The extraction of an appreciable amount of cellulose from the CC 8592 variety residue was also evidenced by the bands that appeared at 1162-1121 cm-1 [17].
Hemicellulose B extracted with NaOH and KOH (Fig. 4. and Fig. 5.) from the residues of the three varieties had a more homogeneous spectrum. In all of them, it was common to find an intense band at 1044 cm-1 and 1075-1078 cm-1 and smaller bands between 983-992 cm-1 and 1149-1153-1160 cm-1, typical of xyloglucans [17]. This polysaccharide is the most commonly found polysaccharide in grasses and straws, such as sugarcane leaves and tops [7,23], and is the main component of the hemicellulose in the primary non-lignified cell wall of dicotyledonous plants, constituting between 20 and 30% of their biomass [24]. It has also been found as the most abundant hemicellulose in sugarcane bagasse [9] and as hemicellulose B extracted from different varieties of wheat [18]. The band at 897 cm-1 found in the spectra of the three varieties corresponds to the frequency of group C-1 or ring frequency, which is characteristic of β-glucosidic bonds between sugar units [8, 18].
The small band between 1507-1523 cm-1 associated with lignin and present in most of the spectra indicates that the hemicelluloses were recovered with good purity, especially the hemicelluloses A, which confirms that chlorite is a good delignifying agent for residues of the three varieties. However, it was observed that most of the residual lignin was precipitated by ethanol together with hemicellulose B, which was deduced not only by the higher intensity of the bands in this hemicellulose fraction but also by the darker (brown) color remaining (Fig. 6).
3.2. 1 H and 13 C NMR analyses
The hemicellulose A and B fractions obtained with 18% NaOH and 24% KOH solutions containing borohydride were analyzed via 1H NMR spectroscopy to characterize their structural features. In Figs. 7-12, the spectra of hemicellulose A from the three sugarcane varieties and of hemicellulose B from the CC 8592 variety are presented. The peaks were assigned by comparison with chemical shift data reported in the literature [25-27].
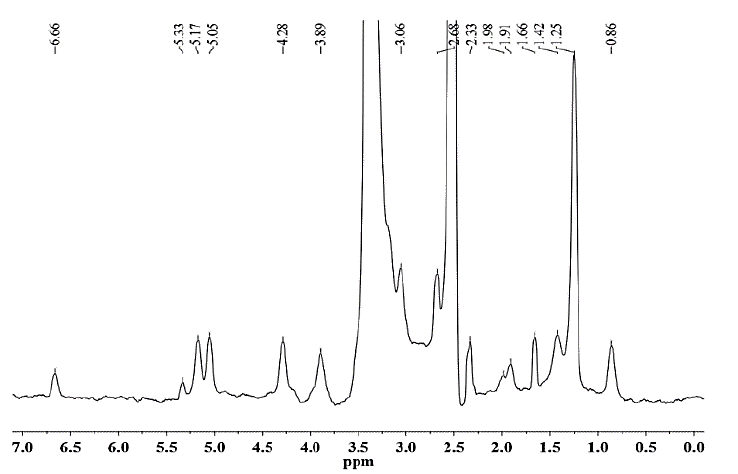
Source: The Authors.
Figure 7. 1H NMR spectrum of hemicellulose A isolated from CC 8592 with 18% NaOH solution containing 0.26 M NaBH4.
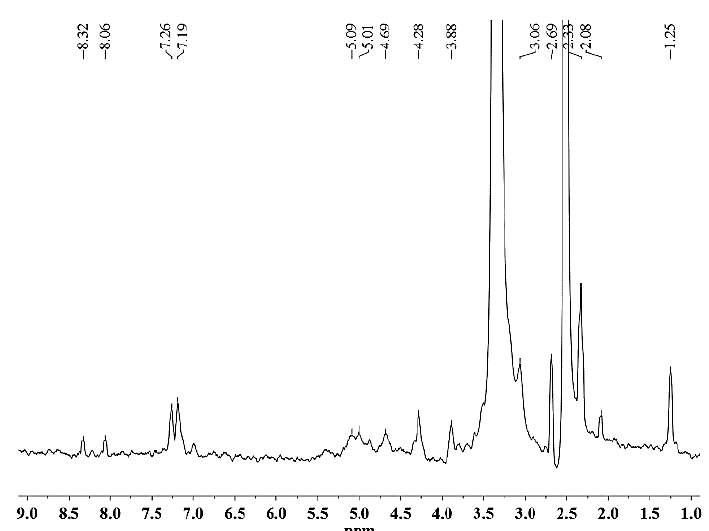
Source: The Authors.
Figure 8. 1H NMR spectrum of hemicellulose A isolated from CC 8592 with 24% KOH solution containing 0.26 M NaBH4 .
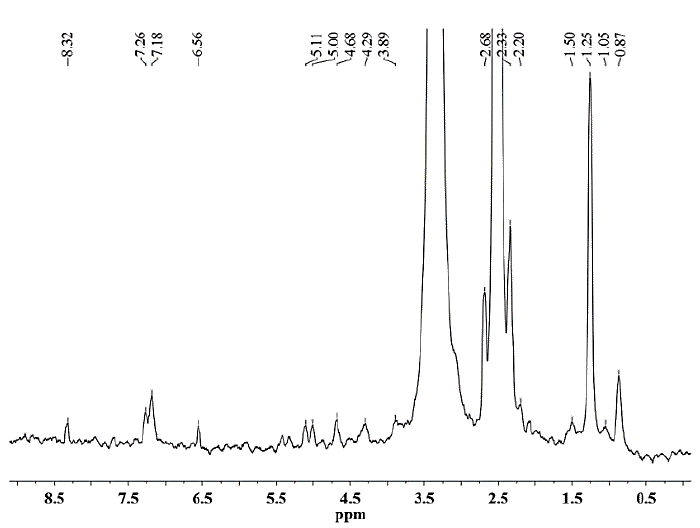
Source: The Authors.
Figure 9. 1H NMR spectrum of hemicellulose A isolated from CC 8475 with 24% KOH solution containing 0.26 M NaBH4.
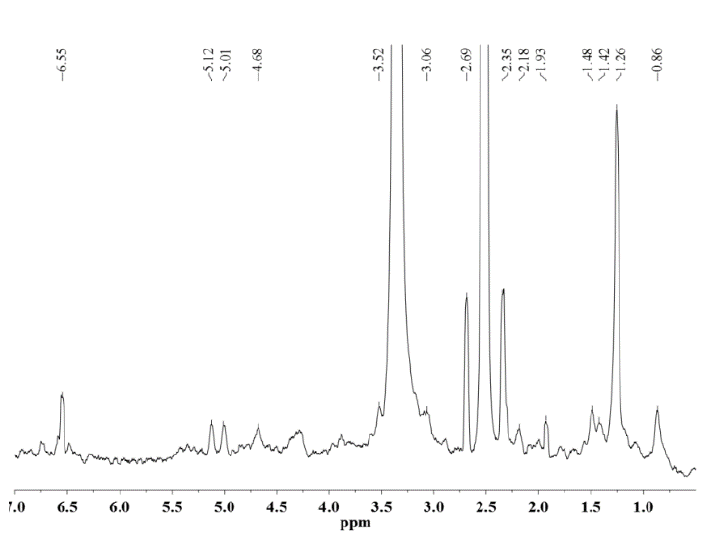
Source: The Authors.
Figure 10. 1H NMR spectrum of hemicellulose A isolated from V 7151 with 24% KOH solution containing 0.26 M NaBH4.
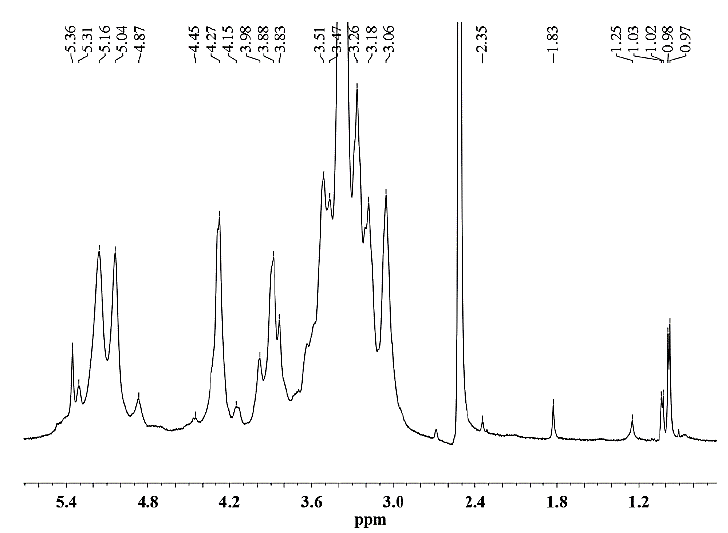
Source: The Authors.
Figure 11. 1H NMR spectrum of hemicellulose B isolated from CC 8592 with 18% NaOH solution containing 0.26 M NaBH4.
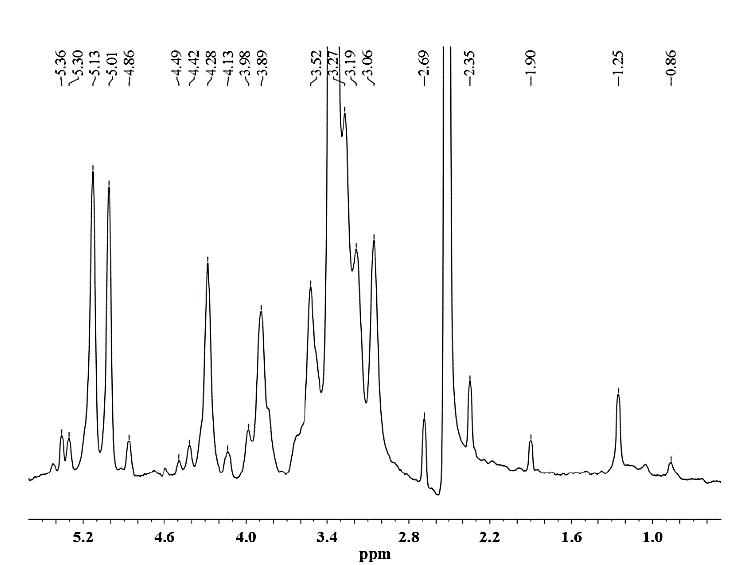
Source: The Authors.
Figure 12. 1H NMR spectrum of hemicellulose B isolated from CC 8592 with 24% KOH solution containing 0.26 M NaBH4.
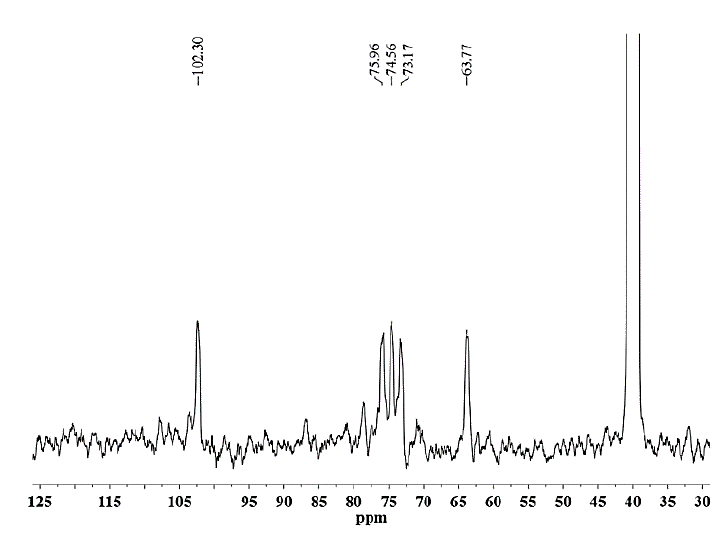
Source: The authors.
Figure 13. 13C NMR spectrum of hemicellulose B extracted from CC 8592 with 18% NaOH solution containing 0.26 M NaBH4.
As seen for hemicellulose B (Fig. 11-12), the signals at δ 3.1-5.4 are due to the presence of protons from arabinose and xylose residues. However, the strong signal at δ 3.33 corresponds to the wastewater present in the DMSO-d6 solvent. The signals at δ 5.36 are due to the anomeric protons of α-D-arabinofuranosyl residues and show substitution of the main chain of xylose at C-2 and C-3. For both hemicelluloses A and B, the chemical shifts of δ 3.1-4.3 correspond to the protons of anhydroxylose units. The signals at δ 4.27/4.28 were assigned to the anomeric protons of the β-D-xylose residues replaced in C-3, taking into account that the region of δ 4.1-4.5 corresponds to the β configuration and the region between δ 4.9-5.6 corresponds to the α configuration. The methyl protons of 4-O-methyl-D-glucuronic acid give weak peaks at 1.0-1.1 ppm [25].
In the spectra of hemicellulose A (Figs. 7-10), well-differentiated signals attributed to the hydrogen H1 linked to the carbon C1 of the glucose (5.0 ppm) and mannose units (5.1 ppm) typical for glucomannans were present [26]. Additionally, the anomeric signal in the 1H NMR spectrum at δ 4.68 was assigned to the (1→4) link of the residue from β-D-mannose [27]. The signals originating from phenolic compounds (6.5 ppm) in the hemicellulose A spectra are due to the presence of small amounts of associated lignin [25] and correspond to the FTIR spectroscopy results.
To confirm the structural features of the hemicellulosic fractions, they were also characterized via 13C NMR spectroscopy. Fig. 13 shows the spectrum for the hemicellulose B fraction precipitated from CC 8592 residues with 24% KOH solution. The spectrum shows five main signals at δ 102.2 (C-1), 75.9 (C-4), 74.7 (C-3), 73.2 (C-2) and 63.3 (C-5), corresponding to the (1→4) link of the β-D-xylose residues [25]. The 1H and 13C NMR results coincide with the FTIR data and confirm the composition and high purity of the hemicelluloses extracted from the sugarcane residues of the three varieties studied.
3.3. Thermal analysis
Thermal analyses provide information about the decomposition reactions that are characteristic of each biopolymer and in some cases can be used for identification purposes. For this reason, TGA and DSC are valuable analytical methods for investigating the physicochemical properties of hemicelluloses.
The TGA curves of hemicellulose A extracted with NaOH from the residues of the three sugarcane varieties showed three phases of weight loss (see Figs. 14-16). In the first phase, the moisture was found to be removed at approximately 100 °C and corresponded to a loss of mass between 5 and 7.5% of the initial value. This retention of water molecules between the branches of hemicellulose is well known and documented [9,17]. Up to 225 °C, some stability of the hemicellulose of the three varieties was observed, very similar to that found in the hemicelluloses isolated from the sugarcane bagasse [9]. In the second stage, there was greater weight loss, and the hemicellulosic fractions began to decompose in the range between 240 and 340 ºC. However, the polymers forming the hemicelluloses of the Colombian varieties CC 8592 and CC 8475 were apparently stable in this range because they lost only 19-16% of their weight, whereas the hemicellulose of the Venezuelan variety V 7151 lost 50% of its weight at 340 °C. The foregoing findings could indicate that the hemicelluloses of the Colombian varieties have a higher molecular weight than those of the Venezuelan variety and of sugarcane bagasse [9], corn stalk, rye straw and rice straw [14]. In the third stage, when the temperature exceeded 400 ºC, weight loss was not evident, with a remnant of 64%, 69% and 40% of solid waste, even at 600 ºC, for the CC 8592, CC 8475 and V 7151 varieties, respectively.
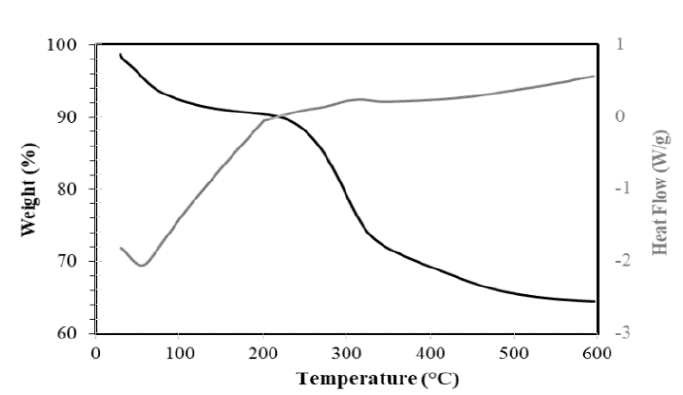
Source: The Authors.
Figure 14. TGA-DSC of hemicellulose A in the CC 8592 variety extracted with NaOH.
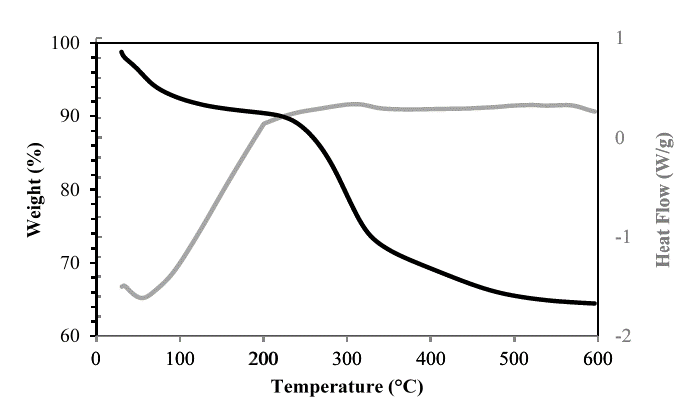
Source: The Authors.
Figure 15. TGA-DSC of hemicellulose A in the CC 8475 variety extracted with NaOH.
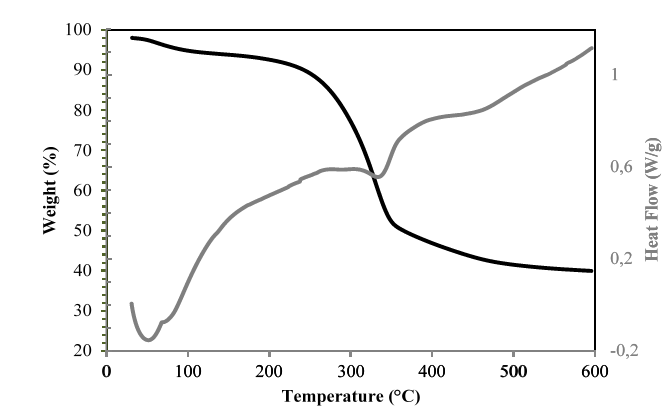
Source: The Authors.
Figure 16. TGA-DSC of hemicellulose A in the V 7151 variety extracted with NaOH.
The DSC curves of the hemicellulosic fractions from CC 8592, CC 8475 and V 7151 showed an endothermic peak at 54, 55 and 52 ºC, respectively, attributed to loss of moisture from the hemicellulose [28]. After reaching 200 ºC, all the hemicellulosic fractions showed a glass transition due to the movements of segments of the polymer chains, increasing their free volume and making them softer. In this process, the hemicelluloses change from their vitreous or slightly ductile form to a viscous fluid or plastic rubber form. The most important factor determining the value of the glass transition temperature is the flexibility of the polymer chain considered in isolation and the interactions between these chains; the chains formed by C-C and C-O bonds are remarkably flexible, and the polymers containing them show relatively low glass transition temperatures due to their amorphous structure. In the end, the DSC curve of each hemicellulosic fraction showed an exothermic peak (at 316 ºC for CC 8592, at 308 ºC for CC 8475 and at 303 ºC for V 7151) related to intramolecular disintegration and polymer decomposition.
3.4. HPLC analysis of monomeric sugars
The composition of monomeric sugars in the raw untreated samples of the CC 8475 and V 7151 varieties was very similar (Fig. 17). Similar results were found by Gomez [2] when analyzing a different batch of sugarcane harvest residues of the three varieties, which were collected at the same time as the harvest was made. In the CC 8592 variety (the most highly cultivated in the Cauca River geographic valley), 50% more xylose and approximately 30% more glucose were obtained than from the other two varieties. However, these results are not conclusive because, in addition to the variety, other factors can have an impact on the compositional difference, such as the agro-ecological zone from which the sample comes, the microenvironmental conditions that affected development, and the time the waste remained in the field, among others.
Analysis of sugars in each fraction showed that the most common sugar in hemicelluloses A and B was glucose and xylose, respectively (Table 1). This indicates that in hemicellulose A, α-glucans were preferentially extracted [29], while xylans were extracted from hemicellulose B, very similar to the FTIR spectroscopy findings.
Table 1. Molar percentage of sugars in the hemicellulose extracted from the residues of the three varieties.
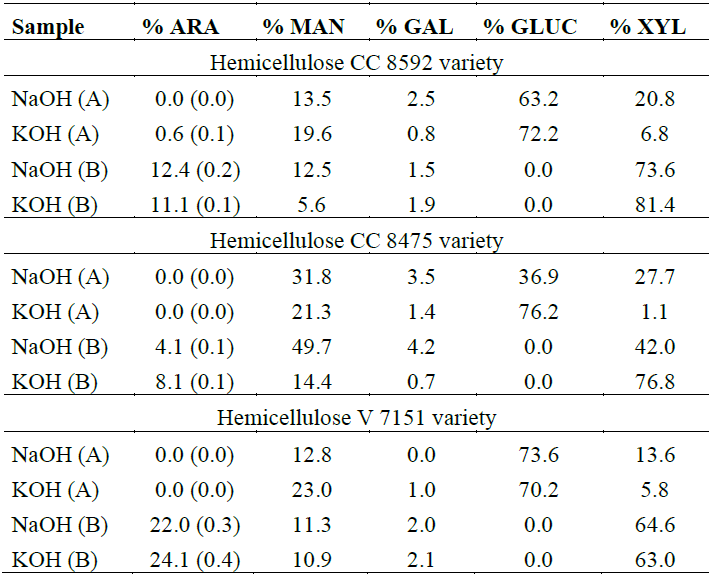
Note: ARA, arabinose; MAN, mannose; GAL, galactose; GLUC, glucose; XYL, xylose. The values in parentheses correspond to the ARA/XYL ratio, typical of arabinoxylans.
Source: The Authors.
In hemicellulose B, the predominant sugar was xylose, followed by arabinose, mannose and galactose, in this order. Altogether, these varieties have a holocellulose content between 60 and 78%, which makes them appealing for bioethanol production. The hemicellulosic sugar content (excluding glucose) was approximately 12%, 17% and 24% for the CC 8472, V 7151 and CC 8592 varieties, respectively. The xylose and arabinose content was comparable to that found in other materials from agro-industrial or agricultural residues, such as corn fiber, rice straw, and even sugarcane bagasse [7]. Particularly, the high xylose content in the residues (8-17%) also makes them promising for production of xylitol, which is used as a sugar substitute.
It was found that the treatment used recovered 83% of the original hemicellulose in the Venezuelan variety, 72% in the CC 8592 variety and only 54% in the CC 8472 variety, on average. In the latter variety, only 42% of xylose was recovered and 69% of glucose. There are many factors that can affect the dissolution of these polysaccharides, including the linear, unbranched or poorly branched structure of the hemicellulose, as well as irregularities in its fine structure, which can be explained by the aggregation tendencies of the macromolecules in solution and the strong intermolecular and interparticle hydrogen bonds formed during the drying process. It is also assumed that these factors that contribute to the formation of aggregates or to the low solubility of polysaccharides also contribute to formation of covalent bonds between these compounds and lignin and other cell wall components via cross-linking through phenolic acids [30], which can be accentuated by greater permanence of the residues in the field after harvest.
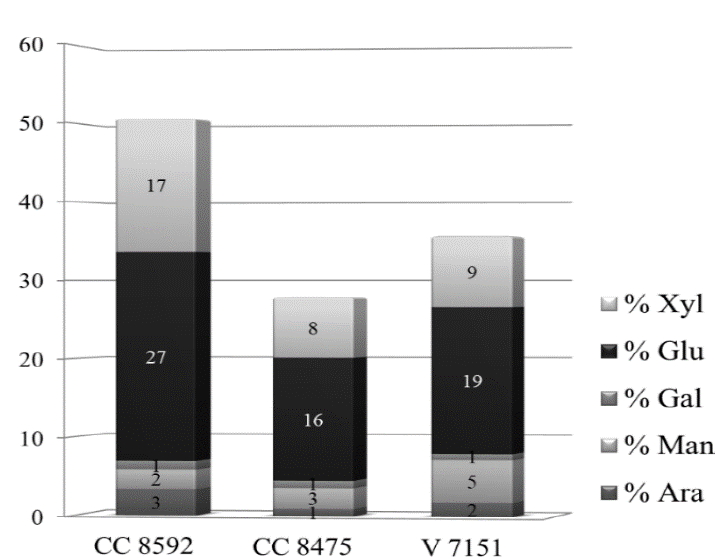
Source: The Authors.
Figure 17. Percentage (w/w) of monomeric sugars in the sugarcane residues of the three untreated varieties.
According to the results presented in Table 1, it can be deduced that within the xylan subclasses, the one that prevails is arabinoxylan (AX) [30], due to the molar ratio of arabinose and xylose, which is approximately 0.1-0.6, as well as the water-soluble AX found in wheat [31]. This range of variation in ratios has also been observed in previous investigations [31-33] and is indicative of the degree of linearity or branching of hemicellulosic polymers. Thus, for the 0.1 ratio, a more linear structure is present, while for the 0.4 ratio, a more branched structure is present, although differences can be found in the distribution of the branches along the main xylan chain [29]. Dervilly-Pinel et al. [32] found that more branched structures degrade to small oligomers more easily than less branched structures. These arabinoxylans can be used in medical applications to lower blood and food glucose levels and to form gels or biofilms [24], and they have also been identified as the main component of sugarcane bagasse [34]. The amounts of mannose and galactose found confirm the presence of arabinogalactans and glucomannans, indicated by the FTIR analysis.
The highest proportion of glucose found in hemicellulose A from the three varieties, in addition to indicating a greater presence of glucomannans, may also derive from cellulose due to the extent of treatment with both 18% NaOH and 24% KOH and/or the oxidation produced by chlorite, which helped in partial depolymerization of the cellulose. This was confirmed by the FTIR analysis, especially of the hemicellulose extracted with KOH from the CC 8592 variety.
4. Conclusions
In sum, 0.05 M HCl primarily extracted pectic substances with side chains of arabinoglucuronoxylan from the CC 8475 and V 7151 varieties. In addition to pectin, arabinoxylan, which is the main hemicellulose from the cell wall of grasses, was extracted from the CC 8592 variety.
By the method described here, hemicellulose A, composed primarily of xylans (arabinoxylan subclass), glucomannans and arabinogalactactans, was extracted from the three varieties of sugarcane and recovered via precipitation during the concentration process through rotary evaporation. Ethanol primarily precipitated type B hemicellulose, which was primarily composed of D-xyloglucans, arabinoxylan type, based on HPLC, FTIR and NMR analyses. According to the TGA analyses, the hemicelluloses of the Colombian varieties were more stable at 340 °C than those of the Venezuelan variety.
In general, the high holocellulose content of more than 60% in the agricultural sugarcane residues demonstrated that they have potential for use in bioethanol production.