1. Introduction
In terms of the generation of wood volume, forest plantations can be more productive than forests. Plantations not only ensure higher production but also a decrease in deforestation. Consequently, If there are strategies that lead to higher wood quality as well as higher volumes that increase the yield in the sawmill, an added value will be generated that can increase the commercialization of the product [1].
The mechanical properties of the trunk of homogeneous trees can vary significantly, even though they have been classified visually [2]. This is of great relevance because the different characteristics of the wood are those that will determine the resource use and profitability in forest plantations [3]. The concept of "tree shape" involves aspects such as the curvature or geometry of the different sections of the tree. Shape is directly related to optimal tree growth, which is the main objective in any commercial forest plantation [4]. Also, growth can be determined as an increase of biomass either of a living organism or of a community, and in the case of forest species this growth is related to the trunk, which is the part of the tree usable for wood production and is expressed as volume [5]. In real terms, a direct measure that can be used to estimate growth is the amount of biomass possessed by the tree. Being able to establish maximum use of the trees is fundamental to the calculation of this biomass, where it is essential to make morphological and structural analysis of the trees. Among the ecophysiological variables for performing such analysis of alometric indicators of plants are involved such as density, wood volume, number of branches, slenderness, total height and height of the trunk [6]. In order to determine the biomass of the tree trunks in this research the volume was taken as a variable indicator that can be related to the physiological processes of the plant such as their use of elements (C and N) and greater or lesser production of branches and roots.
Usually in tropical forest inventories it is common that only the diameter and commercial height are included to characterize tree productivity, but in many cases this commercial height may not reflect tree productivity [5]. Considering these sources of error, it seems necessary to develop indices involving different variables [6]. The measurement of the bark is of importance because it allows us to obtain the volume of wood of the tree. The total or harvestable standing volume of a tree (m3) in terms of biomass is used as a calculation of productivity in forest agroecosystems. This variable can be estimated through different tree types, incorporating in the statistical or biometric model variables such as the basal area, the height, and specific parameters related to the species [7]. In the case of study, the height, base, and upper radii of the shaft, used as a volume calculation of a truncated cone. For these reasons, in order to establish a more precise analysis of the productivity and the shape of the trees, the variables associated with the volume of usable wood were used. This was not only useful as a predictor of productivity but also as an indicator variable that could be related to physiological processes such as the fate of elements in the trunk (C and N) and the greater or lesser production of branches and roots of the plant.
Small changes in environmental factors (climatic and edaphological) can also generate changes in the growth of trees, which are related to limiting soil factors for the growth of forest species, such as humidity and the availability of nutrients for the plant. In addition, texture, porosity, percentage of organic matter and apparent density determine the translocation of nutrients towards the tree's trunk [8]. In the case of A. mangium, if the intake of elements such as C, N and P is made more efficient, the biomass could be increased and therefor the trunks will attain greater robustness and volume for commercialization [9,10].
Indeed, the fate of the nutrients is implanted in the harvested products (food or wood). This is how agriculture works as an open system, where natural systems are modified to produce food and fibres that are exported out of ecosystems, i.e. destined for the market. [11] argues that from the generation of products from different crops, one can see the flow of nutrients from the soil to these products. Unlike natural ecosystems, agroecosystems cannot be self-sufficient in nutrients, thus they require external nutrients that are necessary to compensate for the outputs used for crop production. However, there are alternatives to mitigate the introduction of external inputs that supply the nutrients in agroecosystems. Among these are the use of residues and their inclusion in the soil after their stabilization for the contribution or maintenance of nutrients.
So, it is necessary to search not only for solutions that provide nutrients for the soil, but also materials that contribute to the improvement of soil structure, maintaining the soil in the different soil phases, preventing leaching and supporting greater assimilation by the plant. Biochar has a large specific surface area, a large number of functional groups and high porosity, and these could be an alternative for the retention of nutrients in the soil [12]. One of the fundamental variables in the differentiation of the different types of biochar is the raw material from which they come.
Pyrolyzed plant materials containing large cells in their tissues can generate biochar with considerable amounts of macropores. When applied to soils, these types of biochars can improve soil drainage and capture large molecules [13]. The authors [14] that biochars from wood contain considerable amounts of NO3; the authors demonstrate that the dominant form of N (NO3) is physically trapped within the porous structure of the biochars, before being captured by chemical biochars strategies.
Another factor related to the chemistry of biochar that could help maintain nutrients in the soil is the increase in CIC. This increase occurs when the material is introduced into the soil and comes in contact with water and oxygen, which increase the number of functional groups that will interact with the different nutrients [15]. The authors [17] suggests that these oxygen-containing groups in biochar are responsible for Cu sorption. Similarly, the authors [18] establish that soil Cu is captured by organic ligands of biochar produced from manure. Similarly, important nutrients such as NH4 can be sorted from the colloidal portion of the soil by the biochar. The authors [19,20] observed that NH4 sorption on biochar focuses mainly on ion exchange, coulomb forces, and associations of ammonia functional groups.
In order to model the volume variables and study their relationship to the levels of biochar and synthetic fertilizer, the response surface methodology (MSR) was used in the current research. This consists of a series of techniques that allow the researcher to examine one or more responses in the form of the surfaces, to find the effect that the deliberate variation of quantitative factors has on the response [21] and to find, if possible, the optimum for the combination of levels of factors that yield a maximum or minimum response. In this case, the central composite design with a star portion (central, factorial and axial portions) allows adjusting second degree surfaces, using not only the upper and lower levels of each factor associated with fertilization, but also allows the incorporation of other treatments that facilitate the estimation of second degree terms, therefore achieving more precision in the estimation of wood volume.
The results show that with a lower incorporation of organic fertilizer 15-15-15 of only 84.4 g per plant instead of 100 g (conventional at this stage of production and for this species), complemented with biochar at a dose of 63.1 ton-ha-1, generates an optimal volume of 8566.9 cm3, resulting in significant values for practically all the effects (simple and quadratic) in the linear model explaining the wood volume. These results may encourage the use of biochar accompanied by a reduction in the use of synthetic fertilizers, which is convenient not only for the crop but for the management itself that can be given to wood residues in the management of agricultural production.
2. Materials and methods
We carried out this study in a plantation of the company Cooperación Verde, in their nursery of A. mangium in the township of Planas, municipality of Puerto Gaitán in the department of Meta (Colombia) (coordinates between 3°05' and 4°08' latitude North, and between 71°05' and 72°30' latitude West). The area has an average annual temperature of 30 °C and a total annual rainfall of around 2.300 mm with a bimodal rainfall pattern. The soils that dominate the region are oxisoles and ultisoles and specifically in Planas the soil is the typic troporthent, characterized by shallow soils and low in bases.
For evaluations of the wood volume, estimates were carried out using the volume equation of a truncated cone, using height, the radii of the lower base, and the upper base of the trunk. Measurements were carried out with caliper for the radii and tape measure for the height. This approach was confirmed with a destructive pilot sampling that allowed us to discard the use of the form factor and to use the convenience of the truncated cone instead of the oblique one, since it is the most similar three-dimensional geometric shape in practice for the age of the plantation. For the process of optimizing the volume of Acacia mangium wood, two applications of fertilizer were applied to the soil each at two concentrations, adjusting a second order model for the central composite design with star (orthogonal). In the model two treatment levels were used, namely 40 and 80 ton-ha-1 of biochar and 50 and 100 g per synthetic fertilizer plant 15-15-15. The evaluation biochar, (A. mangium waste biochar) came from thinning residues and pruning from the A. mangium plantation and heating under slow pyrolysis for 14 hours at temperatures between 350 and 400 °C using a pyrolytic furnace located at the plantation. This biochar was produced according to the methodology of [22]. Levels of biochar derive from the research of [23] that estimates an average of 50 ton- ha-1 for an application of this material resulting in an 18-28% increase in the yield of crops on a global scale. Irrigation was not used due to the type of crop and the region where it is established. The seedlings planted in these lots came from seeds collected from a single tree. These seeds were used to establish a nursery three months after planting; seedlings were chosen from heights of 20 to 25 cm and with a diameter at neck height of 5 to 8 mm with as much homogeneity as possible.
The design generated from the central point 60 ton-ha-1 of biochar and 75 g of triple 15 fertilizer per plant with 12 replicates, the four combinations of the factorial portion with 3 replicates each and the four axial points as shown in figure 1. In total 9 treatments of the two factors and 32 runs of the response surface design were generated. All analyses of this model were made with R software.
3. Results and discussion
Fig. 1 shows the points of the response surface design in real and coded variables as well as the optimal point of the estimated response for the combination of 40 and 80 ton- ha-1 for biochar and 50 and 100 g per silver of triple fifteen. The square represents the factorial part, the rhombus the axial part and in the center of the rhombus the central point. The optimum for a volume of 8566.9 cm3 of wood from the analyzed trees of A. mangium in the experimental plantation for one year was reached with an application of 63.1 ton- ha-1 of biochar and 84.4 g per plant for synthetic fertilizer.
Fig. 2 shows the surface generated from the design points that appear as marks on the second degree surface.
Table 1. Analysis of variance for the surface response (central compound design with star points).
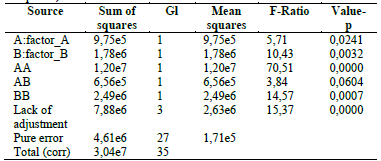
Source: The Authors.
The horizontal plane shows the factors associated with biochar (BAM) and synthetic fertilizer (FS) in coded variables. The percentage value of the latter is due to the fact that levels based on 100 g per plant are used in the conventional dosage usually applied. The response variable (volume of wood in cm3) appears in the height coordinates of the surface. The contours projected on the plane of the factors show an optimal (maximum) within the experimentation region, which falls in the volume region between 8100 and 8700 cm3.
In Fig. 3 only the contour lines are extracted to highlight the optimal value of the response. Table 1 shows the analysis of variance of the second-order model; in addition, the effect associated with the lack of adjustment is populated. In this case, practically all the simple, mixed and quadratic effects were significant or nearly significant (interaction of the two main factors). The mismatch test that is designed to determine whether the selected model is suitable for describing the observed data or whether another type of model should be used shows the appropriateness of the model in terms of its fit.
For other fitness and assumption statistics, the R2 statistic indicates that the adjusted model explains 58.96 % of the variability in volume. The adjusted R2 statistic, which is more suitable for comparing models with different numbers of independent variables was 52.12%. As regards serial self-relation, no statistical evidence of non-compliance with no autocorrelation in waste was presented (p=0.89) (Table 2).
The following eq. (1) is the equation of the adjusted second degree model, where the values of the variables are specified in their original units, where Y ̂ represents the estimated volume, BAM represents the biochar dose and FS the synthetic fertilizer dose.
Table 3 shows the optimal (maximum) for the BAM and FS factors together with the points of the coded variables together with the levels in coded variables where the factors oscillated. The optimal volume was 8566.9 cm3 when using BAM at a level of 63.1 ton- ha-1 and FS at 84.3 g per plant.
A percentage increase was found at the optimal point compared to the control (T1) of 511.52% and 49% with T3 treatment (with 100% FS, conventionally used in the plantation) (Table 3).
In this research it was not only established that after the application of the values found at the optimal point (BAM at a level of 63,1 ton- ha-1 and the FS in 84,3 g per plant), the maximum volume was reached for the tree trunks for the age of the plantation under the established conditions of the study, but also a decrease was obtained in the application of FS, which leads to a decrease of economic and environmental costs in the agroecosystem under study. The use of BAM would also achieve a use of plantation waste that could positively increase the different properties of the soil, that is reflected in higher biomass of the plantation trees and lower waste accumulation.
In general, terms it can be stated that in the last decades different investigations have shown that the application of organic amendments based on organic residues increases the productivity of various crops. The change in soil factors such as increased pH, CIC, nutrient retention and water retention, promoted by the inclusion of biochar, could have a greater impact on the plants. Particularly the contribution of ashes with the application of biochar to the soil could be key to the contribution of available nutrients if we take into account the immediate availability of salts found on the surface of the biochar [24,25]. This "short-term" entry of available nutrients into the soil could be reflected in increased plant productivity [26]. Biochar can provide nutrients to the soil and thus influence plant nutrient uptake and, also, this alters the competitive capacity of certain plant species. In particular, legumes benefit from the addition of biochar [27]. For example, the addition of biochar from residues of A mangium to different red clover and Trifolium pratense crops resulted in an almost three-fold increase in the proportion and biomass of legumes measured after their growing season [28].
The amount of biochar applied to the soil is a determining factor in increasing crop production. In the last decades biochar has been applied in a wide range of application rates ranging from 1 to more than 150 ton- ha-1 for different crops. [23] Wolf et al. (2013) have established by meta-analysis that a large scale application level of 50 ton-ha-1 of biochar would generate an average increase in the production of different crops of 18 %. The authors [29] propose more positive scenarios and they put this increase at 28%. Although in the present study higher levels of biochar application were found at optimal levels, the increase in production was found to be 2.5 times higher compared to research by [23].
4. Conclusions
The use of biochar together with a reduction in the application of synthetic fertilizer 15-15 usually applied in plantations of A. mangium resulted in doses of 100 g per plant under established conditions of the current research. This resulted in an adjustment of the response surface, so that the estimate the maximum volume of wood in a crop of one year of age turned out to be approximately 8570 cm3. This volume was reached with a biochar application of 63.1 ton- ha-1 and a reduction of around 16 grams per plant of 15-15-15, and this will surely be associated with a reduction of production costs. This implies the application of the new residual products of the plantations themselves resulting in lower cost. Also, if biochar is a factor associated with an increase in the volume of wood, its use will guarantee a lower accumulation of plantation residues, which will additionally be reflected in an additional reduction in management costs of these same forest plantation residues.