1. Introduction
It is well known that capsicum yield is very susceptible to water deficit, especially at flowering and fruit development stages. The lack of water during flowering promotes abortion of flowers and fruits, whereas at the beginning of fructification it could lead to the appearance of fruits with apical rot. On the other hand, the excess of water favors the rotting of the stem and roots and also stimulates the abortion of flowers [1,2]. Thus, controlled and optimized irrigation in the capsicum crop is essential for a proper growth and yield, besides reducing the risk of losing all the investments made.
The irrigation depth strategy is a preponderant factor for the activity success. Control and quantification methods of irrigation depths can be classified into three categories: monitoring of climatic or atmospheric conditions, soil moisture and water in plants [3].
The tensiometer is the most commonly used device for soil irrigation management. It determines the soil water matric potential in order to establish the water replacement. However, to guarantee an adequate irrigation management, it is necessary to know the soil water retention curve [2].
The use of electrometric equipment, such as soil moisture sensors, have gained space due to their greater versatility to obtain accurate soil moisture information. Nevertheless, to ensure the precision of the sensor readings it is recommended to calibrate it according to soil type [4].
The quantification of water vapor flow to the atmosphere from wet surfaces in combination with plant transpiration (evapotranspiration) represents the water requirement of the crop. The most used direct method in the management of water resources is the lysimetry technique, which determines the reference evapotranspiration (ETo) through the variation of mass balance or water volume [5].
On the other hand, indirect methods to determine reference evapotranspiration are classified into two groups: mathematical models and evaporimeter tubes or tanks. [6] argue that, for ETo estimation, the Piché evaporimeter has advantages over evaporimeter tanks due to its lower cost, easy installation and handling in a protected environment. Among the equations developed for estimating ETo, the Hargreaves-Samani method is characterized by its simplicity, based on maximum, minimum and average ambient temperatures and extraterrestrial solar radiation [7].
The most commonly used methods are those based on atmospheric characteristics, because they are obtained quickly and easily, but these methods are less efficient when compared to the soil-based management. On the other hand, soil-based management is less accepted by irrigators, as it is more meticulous and difficult to manage irrigation [3].
Considering the need for rational techniques to determine the precise irrigation depth for an adequate water management in irrigated agriculture, this research study intends to evaluate the growth, biomass accumulation in the aerial part and fruit production of the capsicum Rúbia R. cultivated in protected environment as a function of different methods used for irrigation scheduling in drip irrigation systems.
2. Materials and methods
The experiment was carried out at the Federal Rural University of Pernambuco (UFRPE) in the city of Recife, State of Pernambuco - Brazil, where the climate is tropical humid (Ams') according to Köppen’s classification. The experiment was carried out in a protected environment (greenhouse) of the Department of Agricultural Engineering (DEAGRI), whose geographical coordinates are 8° 01’ 07" south latitude and 34° 56’ 53" west longitude, 6.5 m above the sea level.
The soil used is classified as Latossolo Amarelo distrocoeso típico (Oxisol) whose chemical and physical characterization is presented in Table 1, performed according to the methodology of [8]. The soil was collected in the 0-30 cm layer, dried, pounded to break up clods and sieved through a 4.75 mm mesh. Pots with capacity of 10.0 liters were used to store 7.22 kg of soil mass in a usable volume of 5.3 liters, in order to maintain the same soil density found in the field, 1450 kg m-3. After that, the soil was saturated by capillarity for 2 hours and, subsequently, excess water was drained by gravity. When the drainage ceased, and it was considered that the soil had reached a moisture content equivalent to field capacity, the seedlings were transplanted.
Table 1. Chemical and physical composition of the Latossolo Amarelo distrocoeso típico (Oxisol).
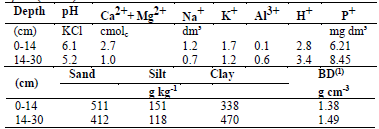
(1) Bulk Density;
Source: The Authors.
Fertilization was carried out following the recommendation of the Instituto Agronômico de Pernambuco (IPA) for the capsicum crop [9]. Basal application was equivalent to 30 kg ha-1 of nitrogen and 120 kg ha-1 of P2O5. In the top-dressing fertilization, doses equivalent to 120 kg ha-1 of nitrogen and 40 kg ha-1 of potassium were split into three portions and applied at 25, 45 and 60 days after transplanting (DAT).
The seedlings of capsicum (Rúbia R. - mean cycle of 120 days) were transplanted to the pots at 35 days after sowing, when they had four true leaves. During the first 15 days after transplanting, the plants were kept under an acclimatization regime. After this period, the differentiation of the treatments with different irrigation depths was started. Throughout each planting row, a training system with one stem per plant and supported by polypropylene ribbon was installed to assist the vertical growth of the plants. The system was composed of wooden stakes spaced by 9 meters and nylon ribbons every 0.2 meters of height above the pot. The control of pests and diseases was carried out by adopting a preventive management, with the application of pesticides recommended for the crop.
The experimental design was completely randomized, in a 5 x 2 factorial scheme, with eight replications, totaling 80 experimental plots. The treatments consisted of the five irrigation scheduling techniques tested to define water depth, which were applied at two irrigation frequencies. Three methods were by climate monitoring: (small weighing lysimeter (LS), Hargreaves-Samani equation (HS), Piché evaporimeter (EP), and the other two were by soil moisture monitoring: tensiometer (TS) and soil moisture sensor EC-5 (SU).
Irrigation frequencies were carried out in two configurations: F1 - full water depth application at 8:00 a.m. (continuous) and F2 split water depth application, half applied at 8:00 a.m. and the other half at 4:30 p.m. (alternating frequency). A small lysimeter system was installed to directly determine reference evapotranspiration (ETo), composed of four pots with soil under the same conditions as those with sweet capsicum, but these were cultivated with Bahia grass (Paspalum notatum), maintaining the height of 12 cm and completely covering the soil, without water stress [10].
For the estimation of reference evapotranspiration (ETo) by the method of [11], only values of minimum, average and maximum ambient and solar radiation were required. Thus, the DHT22 sensor was installed inside the protected environment to monitor temperature and relative humidity. In addition, extraterrestrial solar radiation was obtained via the table.
In order to determine the ETo with Piché evaporimeter (EP), the methodology of [12] was used, which according to [13] has the advantage of not requiring measurements of wind speed and saturation deficit, since this instrument estimates the evaporating power. The device was installed 1.5 meters above ground level in the center of the greenhouse.
The EC-5 soil moisture sensor was installed in six pots at 12 cm depth, as recommended by the manufacturer, and the readings were daily taken at 8:00 a.m. Irrigation management with soil moisture sensors was performed based on soil water availability, in order to allow the plant to consume from 55% to 100% of the water available in the soil. Thus, the established critical soil moisture was 0.20 m3 m-3 and the water replacements were calculated to raise the soil moisture to the field capacity (0.38 m3 m-3). As the EC-5 sensor determines the soil water content indirectly, it is essential to perform its calibration according to the soil monitored. For this reason, the calibration equation developed by [15] was used for this type of soil.
In the same way, tensiometers were installed in six other pots. The irrigations were carried out based on the average value of the matric potential indicated by those six tensiometers, installed at 15 cm depth. The readings were performed daily at 8:00 a.m., by means of an analog tension meter with sensitivity of 10 kPa. At the moment of experiment installation and pot preparation, the volumetric soil moisture contents at the tensions of 6, 9, 12, 15 and 20 kPa were determined. An experiment conducted by [16] with the capsicum crop in protected environment established that the critical water tension in the soil for this crop is 15 kPa. Hence, irrigations were performed whenever this potential was indicated by the tensiometers. The biometric variables of stem diameter (SD) and plant height (PH) were evaluated every 10 days, from 25 days after transplanting (DAT), totaling eight evaluations during the whole crop cycle. PH measurements were made with a graduated ruler, measuring the distance between the base of the stem and the highest leaf of the plant, in cm; while the SD variable was measured using a 0.01-mm-precision digital caliper placed at the base of the stem, approximately 2 cm from the ground.
Leaf area (LA), total leaf area (LAt) and leaf area index (LAI) were estimated according to the methodology proposed by [17]. LA values were estimated after the experiment, measuring the length and width of three leaves, randomly selected, first at the top, second in the middle and the third leaf at the base of the plant, determining the means of leaf lengths and widths. Then, LAt was obtained by multiplying the leaf area by the total number of leaves per plant. Finally, LAI was estimated by dividing the leaf area estimated by the area of soil covered by the plants.
The absolute growth rate (AGR) and relative growth rate (RGR) values were estimated for an interval of 80 days, with the first biometric evaluation performed at 15 DAT and another measurement after 95 DAT of the capsicum crop cycle. RGR and AGR were estimated for the PH and SD variables, according to equations 10 and 11 proposed by [18].
The biometric values of fruits, absolute and relative growth rates, fresh and dry shoot biomass, and the total productivity of the capsicum Rúbia R were submitted to analysis of variance at 1 and 5% probability levels, by the F test, for the sources of variation: different irrigation depths and application frequencies. Then, the significant data by F test were submitted to the comparison of means by the Tukey test at 5% probability level.
3. Results and discussion
Most of the biometric variables were influenced by the irrigation scheduling techniques. However, they were not influenced by irrigation frequencies and factor interaction. Leaf area index (LAI) and stem diameter (SD) were significantly influenced (p <0.01) by the different irrigation scheduling techniques, while plant height (PH) did not differ significantly. [19,20] also found no significant difference in the height of capsicum plants under different irrigation depths.
It is observed that the plant height along the cycle life of the studied variety of capsicum showed standard growth (Fig. 1). Thus, the variations in irrigation scheduling techniques did not cause significant variations in plant height, but stem diameter showed significant variations. This result is due to the variation of the replacement depth, which leads to changes in sap flow, so there is greater sensitivity in this variable [21]. The evolution of plant height growth (PH) as a function of the days after transplanting (DAT) and the different irrigation strategies shows, in general, that plants had similar growth in all treatments throughout the experiment (Fig. 1).
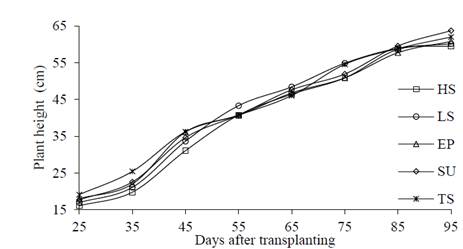
Source: The Authors.
Figure 1. Plant height (PH) of capsicum Rúbia, due to the different irrigation controls and their temporal evolution during the study. HS - Hargreaves-Samani, LS - Weighing Lysimeter, EP - Piché Evaporimeter, SU - Soil moisture Sensor and TS - Tensiometer.
The mean values of leaf area index (LAI) as a function of the different irrigation strategies were submitted to the Tukey test at 5% probability level, which showed higher values obtained with Piché evaporimeter, soil moisture sensor and tensiometer, 1.58, 1.61, 1.67 m2 m-2, respectively (Table 2). These methods provided larger irrigation depths for the capsicum crop throughout the cycle, a condition that contributed to higher LAI values. [6] also found an LAI up to 1.5 m2 m-2 for the protected-environment cultivation of capsicum.
Table 2. Average values of leaf area index (LAI) and stem diameter (SD) as a function of the different irrigation depths defined by the Hargreaves-Samani equation, weighing lysimeter, Piché evaporimeter, soil moisture sensor and tensiometer.
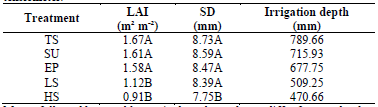
Means followed by equal letters in the column do not differ from each other by the Tukey test at 5% probability level.
HS - Hargreaves-Samani, LS - Weighing Lysimeter, EP - Piché Evaporimeter, SU - Soil moisture Sensor and TS - Tensiometer
Source: The Authors.
The lowest values of LAI were found with LS and the Hargreaves-Samani equation (HS), which differed significantly from the other treatments when Tukey test was applied at 5% probability level (Table 2). This result is due to the fact that LS and HS methods provide the lowest volumes of water applied during the crop cycle, consequently limiting leaf growth. According to [17], plants under lower water availability have reduced leaf area, which is the first line of defense under water restriction conditions.
According to [22], leaf growth depends mainly on cell division and expansion, whose inhibition causes a slow rate of leaf growth at the early in its development; cellular expansion is regulated by the turgor pressure of the cells, so it will only occur with adequate water availability, a fact that justifies a lower LAI in plants under lower volume of water applied.
The mean values of stem diameter (SD) were submitted to the Tukey test, at 5% probability level (Table 2), and it is observed that the techniques of the small system of lysimetry, Piché evaporimeter, tensiometer and soil moisture sensor led to the highest values of 8.39 8.47, 8.59 and 8.74 mm, respectively, which differed significantly from the other treatments. [19, 23] evaluated the effect of different irrigation depths for capsicum and verified that stem diameter is not influenced by the different irrigation depths.
However, the value of SD obtained in plants submitted to Hargreaves-Samani treatment (7.75 mm) differed statistically from those of the other treatments (Table 2). This result is due to the HS treatment, which provided a lower volume of water for the plants, reducing their leaf area and consequently their stem diameter. Leaf area represents the light gathering area for photosynthesis, as a result, is very important to stem growth [24]. Stem diameter (SD) as a function of the days after transplanting (DAT) and different irrigation strategies is shown in Fig. 2. It is possible to observe that during the experiment the HS treatment caused the smallest stem diameters in comparison to the other methods.
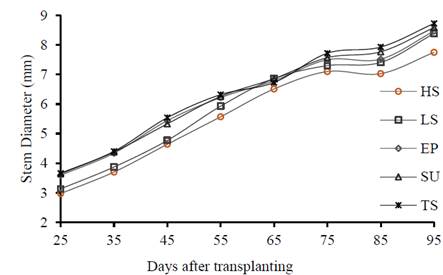
Source: The Authors.
Figure 2. Stem diameter (SD) of capsicum Rúbia as a function of the days after transplanting (DAT) and different irrigation strategies. HS - Hargreaves-Samani, LS - Weighing Lysimeter, EP - Piché Evaporimeter, SU - Soil moisture Sensor and TS - Tensiometer.
Variations in irrigation scheduling techniques caused significant effects on the absolute growth rate of stem diameter (AGRD) and relative growth rate of stem diameter (RGRD). However, there were no differences in the absolute growth rate of plant height (AGRH) and relative growth rate of plant height (RGRH).
AGRD and RGRD were significantly affected only by the Hargreaves-Samani method according to the Tukey test at 5% probability level (Table 3). This fact occurred because it was the method that provided smaller irrigation depths for the capsicum plants compared to the others, which contributed to the smaller leaf area. [24] stated that the relative growth rate is associated with the rate of net assimilation, for relating the photosynthetic rate to the amount of leaf area available for light interception.
Table 3. Mean absolute growth rate of stem diameter (AGRD) and relative growth rate stem diameter (RGRD) as a function of the different irrigation depths defined by the Hargreaves-Samani equation, weighing lysimeter, Piché evaporimeter, soil moisture sensor and tensiometer.
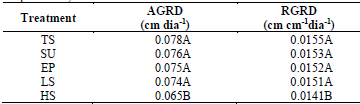
Means followed by equal letters in the column do not differ from each other by the Tukey test at 5% probability level.
HS - Hargreaves-Samani, LS - Weighing Lysimeter, EP - Piché Evaporimeter, SU - Soil moisture Sensor and TS - Tensiometer.
Source: The Authors.
Based on the results of the analysis of variance, it was observed that the fresh biomass (FB) and dry biomass (DB) of the aerial part of capsicum plants were significantly influenced by the single factors irrigation depths and frequencies at 1% probability level by the test F. However, the interaction between the factors did not have a significant effect on these variables.
Comparing the mean values of shoot fresh biomass (FB) and shoot dry biomass (DB) by Tukey test, at 5% probability level, it was observed that the treatments of the tensiometer (TS), soil moisture sensor (SU) and Piché evaporimeter (EP) led to the highest values of these parameters (Table 4).
Table 4. Shoot fesh biomass (FB) and shoot dry biomass (DB) of capsicum plants under different irrigation depths.
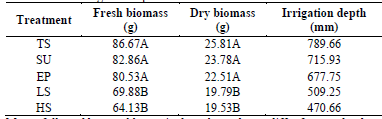
Means followed by equal letters in the column do not differ from each other by the Tukey test at 5% probability level.
HS - Hargreaves-Samani, LS - Weighing Lysimeter, EP - Piché Evaporimeter, SU - Soil Moisture Sensor and TS - Tensiometer.
Source: The Authors.
The values of DB and FB obtained by Hargreaves-Samani (HS) and lysimetry (LS) methods differed statistically from the others by the Tukey test at 5% probability level (Table 4). This fact is probably due to the lower volume of water applied in the plants in comparison to the other methods. Water availability to the plant can change the assimilate partition between the roots and aerial part, which can cause effects of reduction in biomass accumulation in its aerial part [25].
The variables of fresh biomass (FB) and dry biomass (DB) of the aerial part of capsicum plants were influenced by the different frequencies of irrigation by the F test at 1% probability level. The alternating frequency (F2) caused higher values of FB and DB, promoting increments of 12.34 and 22.30% in FB and DB, respectively, compared to F1 (Table 5). Irrigation frequency F2 (alternating frequency) established a nearly constant water regime in the root zone and ensured that plants grew under proper soil water conditions for optimum FB and DB.
Table 5. Fresh biomass and dry biomass of capsicum according to the different irrigation frequencies.

F1 - Continuous frequency, F2 - Alternating frequency
Source: The Authors.
[26] also verified that greater availability of water in the soil favors biomass accumulation in the capsicum crop. Moreover, [22] argue that the low availability of water may interfere with the photosynthetic activity, reducing the growth and, consequently, the biomass accumulation of the plants.
The yield variable was significantly influenced (p <0.01) by the different irrigation scheduling techniques, although the interaction between scheduling techniques and irrigation frequencies did not influence it. The average yield was 11.67 t ha-1, a value close to that predicted by the Instituto Agronômico de Pernambuco for sweet capsicum crop [9] for studied region.
Capsicum yields showed a direct response to the total irrigation applied during whole crop cycle. So, treatments with irrigation management based on soil moisture sensor (SU) and tensiometer (TS), which applied more water (Table 4) had the highest yields, 14.55 and 13.32 t ha-1, respectively (Fig. 3). This result can be justified by the greater water supply to the crop during the whole cycle, contributing to increasing the production of photoassimilates distributed to the parts of the plant and mainly to both formation and growth of the fruits.
The treatments LS, Piché evaporimeter (EP) and tensiometer (TS) did not differ statistically, with yields of 11.23, 12.32 and 13.30 t ha-1, respectively. The lowest productivity was obtained with the Hargreaves-Samani method, differing from the other treatments by the Tukey test, at 5% probability level (Fig. 3). This result is due to the fact that the HS method estimated a lower volume of water for plants, which resulted in a low leaf area index, consequently limiting the growth and development of the fruits.
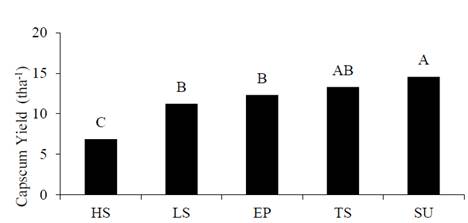
Source: The Authors
Figure 3. Capsicum yield (t ha-1) as a function of different irrigation strategies. HS - Hargreaves-Samani, LS - Weighing Lysimeter, EP - Piché Evaporimeter, SU - Soil moisture Sensor and TS - Tensiometer
Capsicum yield was significantly influenced by the irrigation frequency (Table 6). The treatment of higher frequency (F2) showed higher average productivity (12.65 t ha-1), differing statistically from F1 (continuous irrigation frequency, 10.69 t ha-1). In F2, lower quantities of water were applied in each irrigation pulse, which contributed to maintaining the matric head in the soil within a narrow range of values and soil moisture with steady state resulting in a more stable level of soil moisture compared to the F1 irrigation frequency. So, it kept irrigation threshold constant while promoting more favorable conditions for the development of the crop [27].
Table 6. Yield (t ha-1) of capsicum according to the different irrigation frequencies.

Means followed by equal letters do not differ from one another by the Tukey test at 5% probability level.
F1 - Continuous frequency, F2 - Alternating frequency
Source: The Authors.
The higher irrigation frequency promoted an increase of 18.34% in total capsicum productivity. Corroborating our results, studies indicate that increasing irrigation frequency results in higher productivity values in crops such as tomato and sweet capsicum [28,29].
Many studies claimed that crop yield can be increased by improving irrigation scheduling, applying adequate timing and amounts of water over the growing season of crops. In this study, it was observed that irrigation scheduling based on Hargreaves-Samani equation is not suitable to manage irrigation of capsicum crops in greenhouse on the Northeastern coast of Brazil, as it underestimates the amounts of water to be applied. Also, higher irrigation frequency is more appropriate to obtain higher yields since it promoted better soil water conditions for plant development.
4. Conclusions
Capsicum growth and yield were affected by the irrigation scheduling techniques. Treatments with irrigation managed based on soil sensors (soil moisture sensor and tensiometer) led to greater leaf area index, stem diameter, accumulations of fresh and dry biomass, and yields.
Irrigation frequency also affect capsicum yield. Alternating irrigation frequency contributed to higher productivity and greater accumulation of shoot biomass.