1. Introduction
Many plants and their biomass are currently being investigated due to their antioxidant properties and their ability to scavenge free radicals, which have been involved in the development of a number of disorders including cancer, neurodegeneration, and inflammation. As a result, studies into antioxidants have been conducted to prevent and treat such diseases and meet the increasing demand for natural antioxidants and food preservatives [1,2]. For example, chlorogenic acid (CGA, CAS: 327-97-9), an ester formed by caffeic and L-quinic acids, is a widely distributed polyphenol in upper plants [3,4] and a high value-added phytochemical used by the pharmaceutical and food industry due to its biological activity and functionality as antioxidant, antiviral, and hepatoprotective, among others [3], [5].In addition, studies have determined that it possesses anti-inflammatory, bactericidal, and antitumor properties [6,7]
A major source of CGA is green coffee beans. However, some of the byproducts of the coffee industry also have an interesting CGA content, in a range between 1.3 and 3.5%P/P, such as coffee husk, silver skin, spent coffee, and coffee pulp [5]. The latter is considered a raw material, because it is generated in high quantities, as well as agroindustrial waste; therefore, CGA extraction processes using this second-generation biomass would not compete with the human coffee consumption [8]. Moreover, the pulp is the first byproduct of the wet process of the cherry coffee, during the sub-process called pulping [5]. Such residual biomass represents about 29% in dry basis weight of the cherry [9,10], that is, approximately 43.58% of the weight of the fresh fruit [11]. This means that, for every ton of pulped coffee, there would be approximately two tons of pulp [9].
The aim of the present work is to stablish a methodology to identify the adequate solvents, or mixes of them, to extract any phytochemical or organic complex compound in a study case in which the extraction of chlorogenic acid (CGA) from coffee pulp is performed using numerical selection methods (i.e., Hansen solubility parameters and Ra number) and decision-making tools (i.e., health, environmental, sustainability, safety and economic criteria). Additionally, this work considers a residual biomass revaluation and reincorporation in the value chain because the only way to take advantage of coffee pulp is through a composting process to convert it into low-quality manure. Nevertheless, in many cases the process is not carried out using technical methods and adequate infrastructure, which causes negative environmental impacts on all the water bodies near the coffee processing facilities [9,12].
2. CGA solid-liquid extraction process
Chlorogenic acid (CGA), as well as some alkaloids and vegetable oils, are commonly extracted using organic solvents (i.e., chloroform, dichloromethane, etc.); however, such solvents could be toxic, harmful to the environment, and difficult to handle [13]. For those reasons, other techniques, such as supercritical fluid extraction, have been implemented in spite of their requirements of large investments in equipment and facilities for their industrial operation. Hence, supercritical fluid extraction lacks simplicity at that scale [14], [15]. In addition, this technique depends to a great extent on the solubility of the volatile substances in the supercritical fluid. CO2, a nonpolar solvent with low affinity with polar substances, is commonly used. Therefore, the solubility of the substances in the CO2 fluid, decreases with the increase in the number of polar functional groups (i.e., hydroxyl, carboxyl, amino, and nitro), whereby the solubility of the chlorogenic acid molecules tends to be low [13]. As a result, the most viable and efficient method for the extraction of CGA is a leaching or solvent extraction process [5] using solvents related to such active principle.
In a solvent extraction process, the main task is to find the most suitable solvent to extract the phytochemicals of interest from the raw vegetable material [16-18]. However, during the early stages of the extraction process, experiment-based methods are usually employed to select the best performing solvent from a preselected shortlist [19], which implies spending, reagents, time, human resources, and installed capacity, among other resources.
Several studies into solvent extraction selection apply the concept of green chemistry, which is based on 12 principles presented as guidelines to design chemical products and processes. Green chemistry aims to reduce or eliminate the use of substances that can be harmful and dangerous for the environment and human health, as well as to decrease energy consumption in order to have renewable feedstocks [20-22].
To reduce the volume of required solvents in any extraction, advanced equipment, devices, and techniques can be applied, such as supercritical fluid extraction and microwave-assisted extraction. Perhaps, these techniques require advanced and costly equipment [15]. Furthermore, the extraction yield not only depends on the extraction method but also on the solvent’s physical-chemical behavior, which changes when polarity, pH, temperature, extraction time, or sample composition vary [23,24].
Therefore, this paper explains a valuable methodology as a contribution to the development of the solvent selection guides because it considers, simultaneously, numerical, technical, risk, and feasibility analyses.
3. Methodology
In this work, a solubility study was performed based on data reported on common solvents as: water, methanol, ethanol, n-propanol, acetone, t-Butyl alcohol, ethyl acetate, acetic acid, benzyl alcohol, phenol, benzene, hexane, toluene, d-limonene, mineral oil, and pine oil. Additionally, such numerical results were used to describe the difference in CGA solubilizing capability of the non-polar and polar solvents using Hansen solubility parameter (HSP) and Ra criteria.
Fig. 1 below illustrates the methodology step by step and how Hansen solubility parameters, Ra criteria, sustainability, risk, and economic criteria should be used to select suitable solvents for the solid-liquid extraction process of a phytochemical from a vegetal matrix.
To design a green and sustainable solvent extraction process, additional criteria, besides the solubility analysis, should be considered for solvent selection, e.g., environmental impact, health, safety, life cycle assessment (LCA), ecological footprint, etc. [16,21,22]. Nevertheless, until recently, scant attention has been paid to the development of comprehensive solvent selection guides [16,25].
The methodology is described below, step by step, in more detail, from the numerical determination of the molecule’s physical-chemical properties, to the integration of the solubility and risk analyses and the sustainability assessment based on the economic evaluation. Additionally, a solvent mixing technique and environmental and feasibility evaluations are included and detailed in this paper.
3.1. Group contribution method
In group contribution methods, the physicochemical property of a component is a function of structurally-dependent parameters, which are determined as a function of the frequency and contribution of the groups in the studied molecule [26-28]. The range of application and reliability of these methods depends on the definition of the groups used to represent the chemical structure of the pure component and the model to calculate the properties, among other factors [26,29].
The group contribution method selected in this study was that proposed by Hukkerikar [26] and Marrero and Gani [27] because it considers three orders of characterization. The first one is about each functional group in the molecule; the second and third orders are about how the groups interact with each other, which helps to achieve accuracy in the property estimation [26,27]. The predictive mathematical model proposed in the references above is where f(X) is a function of property X and may contain additional adjustable model parameters (universal constants), depending on the physicochemical property estimation; Ci denotes the contribution of the first-order group of type-i that occurs Ni times; Dj, the contribution of the second-order group of type-j that occurs Mj times; and Ek, the contribution of the third-order group of type-k that has Ok occurrences in a component. w and z take a value of 1 if the molecule has representation in the second order and third order, respectively, and 0 if they do not have such representation.
Hansen solubility parameters are important tools to determine the right solvent to extract CGA or other phytochemical compounds without the need to spend any reagent or energy resources doing unnecessary impractical experimental work at the laboratory [16,30-32] or analyzing the substitution of the petroleum based solvents with a greener solvent for extraction processes [16,33]. Therefore, HSPs are widely used to predict the solubility of many industrial products such as polymers, bio-polymers, drugs, pigments, dyes, and some biological materials in different types of solvents [15,16].
Several papers and studies have incorporated Hansen Solubility Parameters (HSPs) to stablish the solute-solvent behavior. Saini and Keum [34] used such statistical thermodynamic approach to perform a substitution of the n-hexane in the extraction of carotenoids from dehydrated carrots. Houssam et al. [15] took advantage of them to support the development of a liquid-liquid extraction method for resveratrol from cell culture media. Sánchez et al. [16] used them to reduce the list of candidate solvents for pressurized liquid extraction (PLE) of fucoxanthin from P. tricornutum. In turn, Park et al. [35] used HSPs as necessary data to calculate the equilibrium solubility of ascorbic acid, citric acid, butylated hydroxytoluene (BHT), caffeic acid, epicatechin, and epigallocatechin gallate in several organic functional solvents. However, they did not consider a solvent mixture evaluation in their studies as Gantiva et al. [36] did to predict the solubility of naproxen in ethanol-water mixtures. Martínez [37] evaluated the solubility of acetaminophen in propylene glycol and water mixtures. Notwithstanding, these last studies are focused on pharmacological applications, not the development of solvent extraction procedures.
Additionally, HSPs have been shown to be an effective and practical way to understand issues related to the solubility and influence of different types of intermolecular interactions present in the dissolution process, particularly solute-solvent interactions [25,35]. These parameters indicate weak inter-molecular interactions or dispersions (δd), polar interactions (δp), and hydrogen bonds (δh). Since CGA is an unconventional molecule, a group contribution method is required to numerically estimate its physical and chemical properties, in this case, its HSPs. The methodology used here was presented by Hukkerikar et al. [26] and Marrero and Gani [27]. They introduced a guide to characterize a molecule through its functional groups because their multilevel estimation method does not need substantial computer resources and it allows more accurate and reliable estimations of a wide range of chemical substances, including large and complex chemical compounds such as biochemicals and unconventional solvents, among others [26,27].
3.2. Hansen Solubility Parameters (HSPs)
Solutes and solvents with similar HSPs display affinity, i.e., when there is similarity between the terms, dissolution will occur [35,38-40]. The following expression can be used to mathematically explain the previous statement:
where Ra [MPa1/2] is the distance, in the three-dimensional space, of the solubility parameter between the solute and the solvent; 1 denotes each HSP of the solvent and 2, the target phytochemical or organic complex compound whose affinity will be determined. If Ra approaches or tends to zero, a greater affinity and degree of solubilization between the solute and the solvent under analysis can be achieved [31,32,39]. The melting point of pure CGA is used in this work to measure the degree of accuracy of the property prediction model because such data was determined through experimental work and reported in several databases, i.e. in [41].
3.3. Solvent mixture evaluation
A solvent mixture can display more affinity with the chemical target than a pure solvent and can enhance the extraction yields [23]. To complete the analysis, a solvent mixture is assessed at several ratios of two of the best pure solvents. Such assessment serves to evidence if, compared to pure compounds, the mixture shows improvements in its solubilizing properties, risk and sustainability indicators, and feasibility of the CGA extraction process.
Eq. (3) is used to calculate the Hansen solubility parameters (δpolar, δdispersion and δH2 bonds) of the mixture of two or more solvents.
where δmixture is the Hansen parameter of the mixture; δ, the value of the Hansen parameter k of component i; and ν, the volumetric fraction of solvent i in the mixture.
3.4. Decision and management criteria as a tool in a solvent selection method
This information is a valuable input for the solvent selection methods because few papers include the solubility analysis of several complex solutes, the assessment of solvent mixtures, and, at the same time, other indicators and decision tools that can enhance and support solvent selection processes. Furthermore, it can be used to apply management, business, environmental, and other decision criteria to ensure that the selected solvent will be sustainable and environmentally friendly, regardless of its use.
To perform an efficient extraction with a minimum volume of solvent or solvent mix, the latter should satisfy certain requirements, including a great affinity with the solute, ease of recovery of the desired solute from the bulk, chemical handling, and potential harmfulness [42]. Therefore, the decision and management criteria use sustainability analyses, risk assessments, and economic valuations as tools. The selection criteria are detailed below.
3.4.1. Sustainability analysis
Another environmental and more quantitatively accurate indicator is the carbon footprint, which is related to the measurement of the emission of Green House Gases (GHGs) and considers a life cycle assessment to quantify all the inputs or output flows of materials and energy. In that way, it can determine the environmental impacts of a product, which are defined as equivalent KgCO2 [43-46]. The solvents with the lowest carbon footprint are the most sustainable and, therefore, they must be selected if an environmentally-friendly and sustainable extraction process is the goal.
Table 1 shows the carbon footprint values per Kg and per L of the advisable solvents and other solvents that require dangerous handling. This study does not consider a solvent recovery process; therefore, the numerical impact factor is that of a virgin solvent in all the cases.
If a procedure to recycle solvents is implemented, it could reduce the carbon footprint and greenhouse gas emissions by 92% compared to the average emissions associated with the production of virgin solvent [47]. Therefore, it is a good GHG mitigation alternative and a sustainable proactive strategy.
3.4.2. Risk Assessment (RA)
In this work, risk was assessed following CHEM21’s selection guide for typical and less-common solvents used in the industry. Such guide was written based on a solvent use survey, mainly of pharmaceutical industries. To rank the solvents, we propose a set of Safety, Health and Environmental criteria aligned with the Global Harmonized System (GHS) and European regulations. Our methodology is based on a simple combination of those parameters, and it assigns an overall preliminary ranking to any solvent [48]. RA is complemented with an LCA analysis for sustainable solvent selection, which becomes a technical decision criterion and a tool to support the environmental management system (EMS) and occupational health and safety system inside a laboratory before working with chemical products or any solvent. Furthermore, it can be compared with non-environmental criteria, such as cost-benefit, specific energy consumption, and material performance [49].
Additionally, RA can be used to define a solvent selection method motivated by hazard reduction and gaining an understanding of the systemic environmental consequences of a product, process, or service [42], [49]. This is a valuable contribution because some of the first solvent selection tools were directed at solvents as cleaning agents and they did not consider important issues in the pharmaceutical industry such as process safety [21].
3.4.3. Economic evaluation
This criterion considers the commercial price of the solvents per liter, which is available from several companies that sell chemicals around the world. In this case, the data were taken from Merck-Millipore (http://www.merckmillipore.com/CO/es); with that information, we conducted a comparative study to identify the cheapest solvents and mixtures.
This criterion was applied to the solvents that fulfilled the previous requirements and are advisable for phytochemical extraction purposes. Fig. 2 shows the price per liter of different solvents (in US Dollars) that are conventionally used at the industrial scale. The economic data was used to compare the solvents and identify the cheapest option.
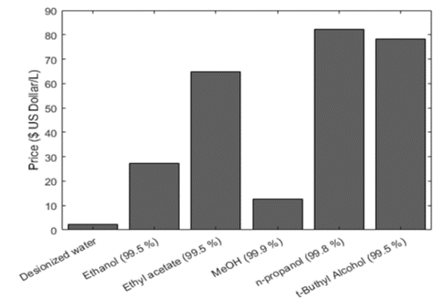
Source: Adapted from [50].
Figure 2 Price per liter of different advisable solvents and methanol (in US Dollars).
3.4.4. Quantitative evaluation of the non - solubility criteria of the mixture
It is necessary to consider a numerical way to evaluate the mixture applying the criteria mentioned above. Eq. (4) is used to quantitatively assess the risk and sustainability of any solvent mixture in order to establish its economic feasibility.
Where Imixture,𝑗 is the criteria value of the mixture; F, the value of the sub-criteria parameter k of component i (safety, human health and environmental risk, carbon footprint and sale price); and ν, the volume fraction of solvent i in the mixture.
4. Results and discussion
4.1. Physical-chemical characterization of CGA
CGA chemical structure is illustrated in Fig. 3. As can be seen from the molecular structure of CGA, this is a highly polar molecule due to the presence of hydroxyl groups (-OH) and the carboxyl group (-COOH) in it; therefore, this molecule is very soluble in water [35]. However, a solvent or solvent mixture with better CGA solubilizing properties than water could be found through a numerical analysis.
Tables 2 and 3 present the characterization of ACG by functional groups. To validate the data obtained for the characterization and properties of the molecule, its melting point was calculated and compared with the actual value in the literature. This information was obtained following the methodology proposed by Hukkerikar et al. [26] and Marrero and Gani [27]. This was a good approximation to its real behavior because the actual melting point of CGA and such point calculated through numerical methods are very similar; the comparative error between both was 2.68%, which is lower than 5%, the value recommended in the literature [26,27].
Fig. 4 shows the HSPs of CGA and the polar solvents, whose Ra are below that of water since CGA is reported to be very soluble in this inorganic and very polar liquid. The Ra number of water and CGA is far from zero because the HSPs of these compounds are different. Although Eq. 1 will never equal zero, experimental works have reported a high solubility of CGA in water [35,51]. Therefore, water was used as base solvent, and Ra values of water below this parameter will be a good numeric indicator of the solvent’s capacity to solubilize CGA. Although acetone is a non-polar solvent, it is found together with polar solvents because the Ra value of this organic compound is lower than that of water. Additionally, Fig. 5 shows the HSPs and Ra of CGA and non-polar solvents commonly used in the industry to extract essential oils and non-polar organic compounds.
As can be seen in Fig. 5, the non-polar solvents that have been reported to be unable to solubilize CGA due to their polarity behavior show a Ra number very far from zero, but, in all cases, above that of water.
A comparison between the Hansen Solubility Parameters of CGA and those of the polar and non-polar solvents reveals a trend: the non-polar solvents do not have the capacity to solubilize GCA because their polar HSPs equal zero and their H2-bonds HSPs are very low. Therefore, this data is evidence that polar molecules display an affinity with solvents, which have polar and H2-bonds similar to them, and these HSPs values should be different from zero. The above is the expected behavior, and it validates the HSP methodology adopted in this study. Adequate solvents to extract CGA are small molecules that present low or null steric hindrances, polar functional groups in their chemical structure, and high potentials to form H2-bonds.
4.2. Risk assessment of pure solvents
Table 5 shows the risk analysis of solvents with the highest affinity with CGA and their classification. In addition, CHEM21’s selection guide of classical and less-classical solvents was used to identify the safest and most advisable solvents.
Table 5 Risk assessment and classification. Assessment: 1-3, advisable; 4-6, problematic; and > 7, dangerous.
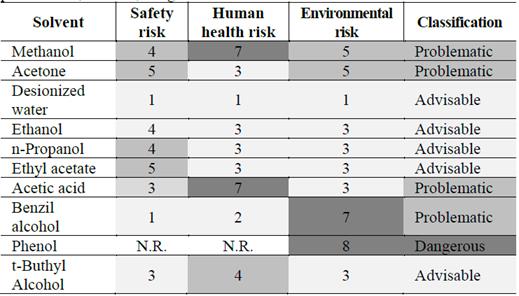
Source: Adapted from [49].
According to the steps and data included in the solvent selection guide, advisable solvents are those whose assessment is under 3. Deionized water, ethanol, propanol, ethyl acetate, and t-Butyl alcohol fulfilled that condition. The other solvents will not be considered pure or at high concentrations because their usage would be dangerous and risky for human health and the environment.
4.3. Sustainability analysis of pure solvents
As can be seen form Table 1, methanol and deionized water presented the lowest carbon footprint values. However, methanol was classified as a problematic solvent because of its high risk to human health due to its toxicity and dangerous handling [48]; moreover, it is forbidden for cosmetic applications according to Annex III/52 of CosIng, the database of ingredients and raw materials used by the cosmetic industry in the European Union.
In Table 5, acetone, benzyl alcohol, and acetic acid are classified as problematic solvents, and phenol is considered a dangerous chemical. Therefore, the non-solubility criteria were only applied to the mixtures of advisable solvents and non-risky mixtures.
4.4. Solubility and affinity analysis of solvent mixtures and CGA
Fig. 7 shows the Ra number of CGA and several solvent mixtures. It should be noted that the HSPs of water in this mixture were taken from [39,40] and the case Water with Organic Liquid.
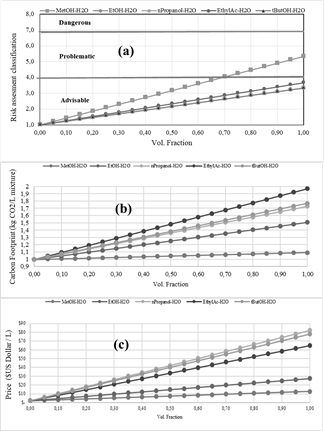
Source: The Authors.
Figure 7 Solvent selection. Non-solubility criteria results; (a) Risk Assessment classification of several aqueous solutions; (b) carbon footprint (KgCO2/L mixture) of several aqueous solutions; (c) price of several aqueous solutions ($ US Dollar /L).
Based on Fig. 6 and the Ra definition, it can be inferred that the minimum value in the curve is the best mixture ratio because, at that point, the Ra number is at its lowest value and closest to zero. The mixtures to solubilize CGA that meet that condition are ethyl acetate, methanol, ethanol, and t-Butyl alcohol, all of them in aqueous solutions, because such mixtures show a minimum value in the Ra curves, near 0.05.
However, when the target chemicals are inside a biomass and water is used as co-solvent in the extraction process, its quantity in the mixture should be considered because it can promote fermentation processes and microorganism growth due to issues related to the appropriate storage or handling of the samples [52]. Therefore, when water is a co-solvent, volume fractions around 0.4 or less are recommended so that the next phytochemical separation and purification stage is not overcomplicated and/or the biodegradation of valuable chemicals is avoided.
Thus, the mixtures that show a high performance and affinity with CGA at volume fractions greater than 0.4 are EtOH-Water and MeOH-Water. The latter is the best option based on Ra criteria, but it was discarded due to its classification in CHEM21’s guide. Nonetheless, a mixture analysis of the advisable pure solvents was conducted.
4.5. Non-solubility criteria for solvent mixture selection
Fig. 7 shows the results of the non-solubility criteria. All the evaluations studied several mixtures of advisable solvents and compositions that have good affinity with CGA.
Based on CHEM21’s Risk Assessment guide and the results of the solvent mixtures in Fig. 7 (a), all the solvents mixed with water evaluated here are advisable at any volume fraction, except for methanol because such alcohol turns problematic at concentrations higher than 0.7. Hence, if methanol is employed, the advisable fraction in an aqueous solution must be below 0.7. Likewise, all the alcohols studied here are adequate since they are not dangerous mixed with water at any volume fraction, except for methanol, which becomes problematic at a certain concentration.
Based on Fig. 7 (b), the mixture with the lowest CF indicator at all proportions of solvents was MeOH-H2O, always below 1.1 KgCO2 per mixture L. Nevertheless, in the Risk Assessment, MeOH-H2O is a problematic solvent at volume fractions higher than 0.7. As in the Risk Assessment, all the alcohol mixtures presented a low Carbon Footprint compared to ethyl acetate-water solutions.
Fig. 7 (c) shows the solvent mixtures that would guarantee an economically feasible operation: methanol-water and ethanol - water. This is because, at all the proportions, these aqueous solutions stay under $30 (US Dollars), while the rest of the advisable solvents reach such value at volume fractions between 0.3 and 0.4, which represents a problem since biomass biodegradation processes can occur at those fractions.
Another point related to hidden costs is azeotrope formation because it requires a high amount of energy resources and expenses and a novel separation technology [53], [54] which could affect the benefit-cost of subsequent phytochemical separation and purification processes.
5. Conclusions
Determining the HSPs and Ra number of any chemical is a numerical way to save time and material resources in a laboratory because they can be used to identify the adequate solvents to perform a solid-liquid extraction process without spending excessive reagents. Therefore, the methodology proposed here to select solvents and/or their mixes to extract CGA applies several principles of green chemistry related to the rational, safe, and sustainable use of solvents involved in extraction processes. For that reason, such methodology is not limited to the selection of solvents for CGA extraction processes, and it can be used to find the correct extraction solvent or mixture of solvents for the solid-liquid extraction process of any complex organic or phytochemical compound, even if not enough experimental data are available. This is because said approach considers a physical-chemical property estimation based on an accurate group contribution method with three orders for a detailed chemical structure characterization.
If the economic factor is not considered in the same proportion as HSPs, Ra, safety, human health, environmental, and sustainability criteria (as Carbon Footprint), deionized water, ethanol, ethyl acetate, n-propanol, t-Butyl alcohol, and their mixtures would be adequate solvents, taking into account that the highest affinity with CGA is achieved when deionized water works as a co-solvent in the mixture.
In accordance with the Risk Assessment, methanol and water mixtures are not recommended at volume fractions greater than 0.7 because they are classified as problematic, and, for that reason, the operational risk and final disposal cost could be increased. Therefore, ethanol-water and methanol-water are the only mixtures that meet, at the same time, technical, economic, environmental, and low-risk criteria. Nonetheless, the implementation of methanol-water mixtures is limited by its composition, risk classification, and the fact that it has not been accepted as a solvent to manufacture products for human consumption.