1. Introduction
The discovery of colossal magnetoresistance (CMR) in magnetite materials has motivated the study of their remarkable physical properties. For instance, the role that doped holes play in the CMR of magnetite materials is well known. Specifically, for Manganese Oxide and Manganese doped with Cobalt/Nickel, the CMR effect has been explained by means of a double-exchange mechanism for
samples. Nonetheless, there are different points of view as to the formal charge of either cobalt or nickel and the interaction 𝐶o⁄(3𝑑)−𝑂 (2𝑝)−𝑀n(3𝑑). Goodenough [1] has suggested that the charge distribution for the compound distribution in
and
samples should be trivalent either of cobalt or nickel with a low spin state (
for Co3+ and
for Ni
3+) and the resultant ferromagnetic interactions (Ni
3+, Co3+) - 0
2 -Mn
3+ allow a spontaneous magnetization to occur in such compounds. Toulemonde [2] employed X-ray absorption spectroscopy (XAS) at high energies and noticed the formal charge from dopant elements are mostly seen in Ni
2+ and Co
2+, which aligns with the results obtained by Blassel [3] for compounds of
, suggesting that the ion combinations of Co2+ (Ni
2+) and Mn
4+ should be more stable than trivalent ions. Consequently, these authors established that the exhibited ferromagnetic effects of the samples studied are governed by strong interactions between ions of (Ni
2+, Co2+) - 0
2 -Mn
4+. In contrast, it is well known that the matrix compound of LαMn0
3 is antiferromagnetic and it is relatively easy to induce the ferromagnetic effect using cationic substitution [4]. A notable example is the substitution of alkaline earth metal ions such as Cα
2+ or Sr
2+ at the A-site of LαMn0
3, which carries out paramagnetic-ferromagnetic transitions bounded by magnetoresistance effects. Furthermore, from a theoretical perspective, the substitutions at the manganese site are noteworthy owing to the important role that the lattice of manganese-oxygen plays in terms of the physical behavior of such materials. The cationic substitutions could produce certain changes in the physical properties of both conductive ferromagnetic manganites and the insulator compound of ordered charge. The orbital-ordered ferromagnetic state of LαMn0
3, is easily destroyed, which causes ferromagnetism, but this ferromagnetic behavior can take place by means of several mechanisms, depending on the cation and level substitution. The physical properties can be derived from a complex interaction between the exchange interactions, the orbital-ordered state, and Jahn-Teller distortion (JT) [5-13].
To give another example, an increase of either the length or angle of an Mn - 0 - Mn bound tends to trace the charge carrier, and, furthermore, reduces the double exchange interaction, modifying the Curie temperature (T c .) of the magnetite [14].
In terms of biaxial compression, the stress components σ zz , σ xy , and σ yz are equal to zero. Given by the formula ε xx < ε zz < 0 (compressive strain), and where ( is Poisson’s ratio, the strain component along the normal of the thin film can be written as
As an initial approach, assuming that the volume will be conserved, it can be concluded that εxx = -2εxx when V = 0.5. As the majority of materials have values for V that are between 0.3 and 0.5, their biaxial distortion can be given by the equation ε bi = ¼ (2ε zz - εxx εyy) - that translates as tensile strengths of ε bi < 0 and compressive strains of ε bi > 0.
In this paper, we analyze the effects of strain on the magnetite samples
and
at different doping levels.
2. The effects of strain on magnetite
2.1. The effects of strain on Lα 0.67-x Pr x Cα 0.33 Mn0 3 magnetite samples
The magnetite samples were produced using a solid-state reaction method in which high purity Lα2𝑂3, Cα0, 𝑀n2𝑂3, and 𝑃r2𝑂3 dusts were mixed. The resultant materials were mixed and heated at 800 °C for 16 hours, then pulverized and heated again at 850 °C for 32 hours. Subsequently, the obtained material was mixed again and heated at 950 °C for 64 hours. Finally, the samples were encapsulated and heated at 1200°C for 8 hours. These processes were performed at standard conditions of pressure under which different heat treatments and exposition times contributed to the production of high crystalline quality in the samples.
As depicted in Fig. 1, the crystalline structure of the sample was observed and characterized by X-ray spectroscopy, specifically the X-ray Diffraction Technique (XRD), whose data was processed with X’Pert Plus software. Secondary phases were not observed in the collected spectrum using XRD. In this respect, Table 1 shows data referring to the variation of the lattice parameters at different concentrations of Praseodymium Pr. When Pr concentration is increased, the lattice constant parameters gradually decrease. This result could be attributed to the different ionic ratios of Lanthanum and Praseodymium of around 1.15 Å and 1.09 Å respectively. The presence of Pr causes a shift in the peak signal detection that is well-observed at its peak (202) and shifts from 2( = 32.781 and 32.700 (see x-axis in Fig. 1) and shows a full width at half maximum (FWHM) of less than 0.5117, indicating high crystalline quality for bulk samples.
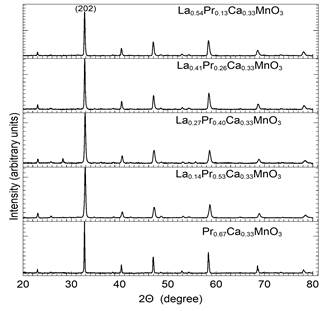
Source: The Authors.
Figure 1 The XRD patterns of the Lα0.67-xPr x Cα0.33Mn03, samples with 0.13 ≤ x ≤ 0.67.
Table 1 Lattice constants and unit cell volume of Lα0.67-xPr x Cα0.33Mn03 samples with 0.13 ≤ x ≤ 0.67.
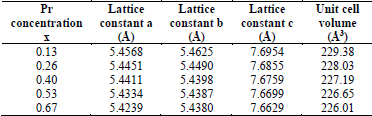
Source: The Authors.
Another important result is depicted in Fig. 2 and shows the behavior of the lattice constant and the unit cell volume which decreases by around 3% due to the smaller ion size of the Praseodymium. Notably, all samples exhibited an orthorhombic structure in pbmn (62) space group.
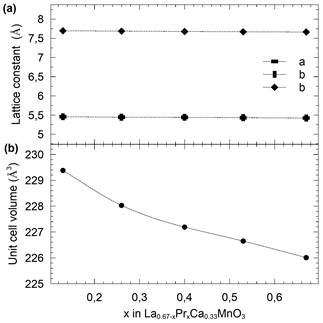
Figure 2 Lattice constants (a) and unit cell volume (b) variation as a function of Pr concentration in Lα0.67-xPr x Cα0.33Mn03 samples. Dashed lines are visual guides.
The substitutions of Pr with an ionic ratio less than that of Lα produced a decrease in Curie temperature T c as a function of the concentration of Praseodymium (see Table 2). Such experimental results are mirrored in other related research [15,16].
Table 2 Biaxial strain, Curie temperature, transition temperature, activation energy and electrical resistivity for Lα0.67-xPr x Cα0.33Mn03 samples with 0.13 ≤ x ≤ 0.67.
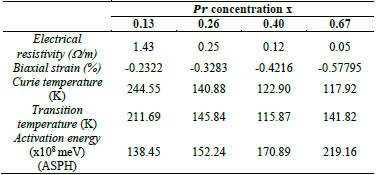
Source: The Authors.
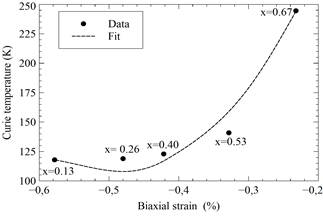
Source: The Authors.
Figure 3 Evaluated biaxial strain versus Curie temperature for different compositions of Lα0.67-xPr x Cα0.33Mn03 samples with 0.13 ≤ x ≤ 0.67. Second degree polynomial (dashed line) fitting the defined evaluated setpoints (dots).
The above result can be understood as follows: on one hand, the substitutions of Lα ions for a smaller ones, like those of Pr reduce the average ionic ratio <r A > which causes a decrease in the electronic coupling matrix element b which describes the electrons hopping among the different sites of Mn. The change in 𝑏 emerges as a consequence of the structural changes that modify Mn - 0 - Mn either through angle or length variations of such bound states [17].
On the other hand, the decrease of the average ratio of A-sites <r A > is related to the decrease in the θ angle of the Mn - 0 - Mn bound due to a rotational action of the octahedrons that reduces the distance between the A-sites. When 𝜃 is decreased, a decrease in b and T c are also observed [17-19].
To clarify the role that the biaxial strain plays, different studies have been performed using ultrathin films and hetero-structures [16]. From these, it is expected that the effects produced by the strain test-induced by the substrate-are different in bulk samples, which influence the relationships between spin, charge, structure, and orbital degree of freedom.
In this context, our calculation of biaxial strain (εbi) produced by the substitution of Lα with Pr in the compound Lα0.67-xPr x Cα0.33Mn03 has been performed assuming, as an initial approach, that the volume of the unit cell is constant. The variations of volume from the unit cell are less than 3% in all cases and were determined by X-ray measurements.
In the case of the mentioned samples, the “tensile” strain effect (εbi < 0) increases with the concentration of Pr. Fig. 3 depicts the behavior of εbi vs. Curie temperature (T c ) and shows the increment in εbi that causes a decrease in T c . An approximated quadratic relation is found in the experimental data (dashed line-fit).
Notably, T c decreases when strain increases and it can therefore be assumed that the biaxial strain increases the Jahn-Teller splitting of the eg levels, which greatly contributes to the determination of the location of electrons. Such results were predicted by Millis and his collaborators [20-22].
The results of the transport mechanism are examined in the context of the data of resistivity obtained at different temperatures T > T c . Thus, T T stands for the temperature of the metal-insulator transition derived from the maximum derivative of dρ/dT. Remarkably, T t is similar to T c and decreases when the biaxial strain is increased. It has been suggested that the rate of temperatures T > T T has a strong effect on polarons [23], in terms of fitting experimental data from transport mechanisms, in which Adiabatic Small Polaron Hopping (ASPH) is considered, and is calculated as follows:
Similarly, Non-adiabatic Small Polaron Hopping (NASPH) is written as
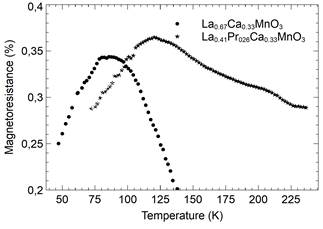
Source: The Authors.
Figure 4 Magnetoresistance as a function of temperature for Lα0.67-xPr x Cα0.33Mn03 and Lα0.41Pr 0.26 Cα0.33Mn03 samples.
where Eα stands for the activation energy and K B the Boltzmann constant. A change of temperature rate is observed that is used for the fitting, which also depends on the value of the biaxial strain. In addition, the resistivity properties of the samples increase with the concentration of Pr, i.e., the increment of ε bi . The activation energies derived from such fittings increase when the biaxial strain is high (see Table 2), which implies a Jahn-Teller-like increment of the lattice distortion in which an increment of Eα would be caused, owing to a greater location of conductive electrons that increase the resistivity and reduce T c .
In contrast, the samples at different rates of biaxial strain when measured for magnetoresistance 𝑀R=[𝑅(𝐻)−𝑅(𝑂)]/𝑅(𝐻) (see Fig. 4) exhibited an increase in MR with a remarkable decrease in T c . A similar trend has been observed in multi-layers tested over different periods and with doped bulk samples, in which a decrement of the difference between the resistivity of the metallic and insulator regimes takes place as the temperature increases. The general observed trend of the MR obeys Eq. (4) [16], as follows,
2.2. The effects of strain on LαMn 1-x Co x 0 3 magnetite samples
The 𝐿α𝑀n1−𝑥𝐴𝑂3 (𝐴=𝐶o,𝑁i) magnetite samples were analyzed by examining strain effects obtained from different substitutions of B-site with magnetite. To correlate the observed effects of the strain tests on the samples, an analysis of their electric and magnetic responses was performed. An analysis of the behavior of the 𝐿α𝑀n1−𝑥Cox𝑂3 sample was performed first and an analysis of the 𝐿α𝑀n1−𝑥Nix𝑂3 sample second.
The 𝐿α𝑀n1−𝑥(𝐶o/𝑁i)𝑥𝑂3 samples were prepared using the sol-gel technique using a solution of lanthanum nitrate 𝐿α(𝑁𝑂3)3 (0.1𝑀),, manganese nitrite 𝑀n(𝑁𝑂3)2 (0.1𝑀), cobalt nitrite 𝐶o(𝑁𝑂3)2 (0.1𝑀), a stoichiometric ratio of nickel nitrate and citric acid 𝐶6𝐻8𝑂7 (0.2𝑀).. The obtained solution was jellified and heated to 100 °C and finally the obtained foam was heated at 600 °C for 4 hours.
Fig. 5 depicts the X-ray spectrum for different concentrations of cobalt where an orthorhombic structure with 62 space group pbmn can be observed. In such samples the chemical composition and morphology were determined by EDX and SEM, respectively. The inhomogeneity of the compositions is lower than 5%.
Furthermore, several measurements of ac-susceptibility were taken using a Lake Shore 7000 susceptometer with a frequency of 20 Hz and a magnetic field strength of 800 A/m, with a range of temperatures between 80° K and 300° K.
The real and imaginary part of the susceptibility as a function of temperature for different concentrations of cobalt is shown in Figs. 7a and 7b. The paramagnetic-ferromagnetic/anti-ferromagnetic transition and Curie temperature (T c ) were obtained using the derivative of dX´/dT. Notably, the temperature T c increases when the concentration of cobalt is increased, which can be related to the increased interaction of double-exchange. The observed peaks of susceptibility suggest that they can be understood as a spin-glass behavior related to competition between the ferromagnetic and anti-ferromagnetic interactions [24].
Table 3 Lattice constants and unit cell volume of the 𝐿α𝑀n1−𝑥Cox𝑂3 samples with x = 0.0 to 0.5.
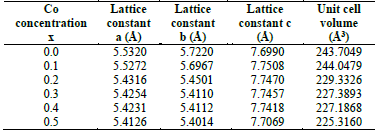
Source: The Authors.
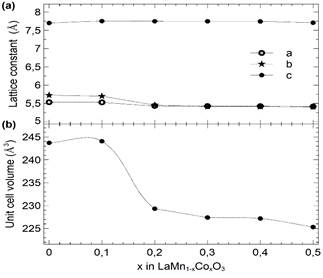
Source: The Authors.
Figure 6 Lattice constants (a) and unit cell volume (b) variation as a function of Co concentration in 𝐿α𝑀n1−𝑥Cox𝑂3 samples. Dashed lines are visual guides.
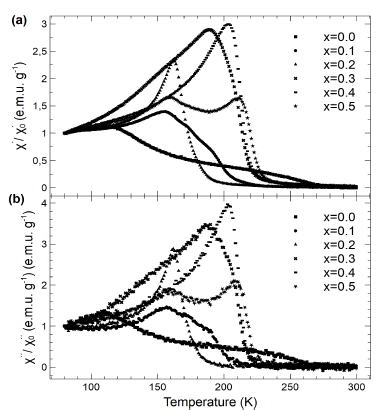
Source: The Authors.
Figure 7 (a) Real (X/X0) and (b) imaginary (X/X0) component of the normalized ac-susceptibility as a function of temperature for different compositions of 𝐿α𝑀n1−𝑥Cox𝑂3 samples (x = 0.0 to 0.5).
Fig. 8 shows the resistivity as a function of temperature for different concentrations of cobalt. A metal-insulator transition can be observed where, at specific temperatures, the change in resistivity is abrupt and is denoted by T t and determined by the maximum derivative of the resistivity vs. temperature (dρ/dT).
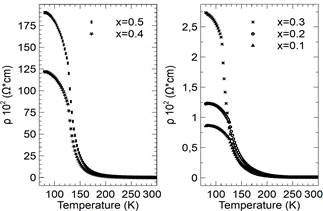
Source: The Authors.
Figure 8 Resistivity (() as a function of temperature for different compositions of 𝐿α𝑀n1−𝑥Cox𝑂3 samples (x = 0.0 to 0.5).
Table 4 lays out the assigned temperature for different levels of samples doped with cobalt. The increase in resistivity is closely related to the observed rise in activation energy and T t , which can be attributed to an increase in electron hopping among the different sites of Mn. In this respect, T t rises to higher temperatures when the concentration of cobalt increases, exhibiting a clear correlation between these occurances. However, the values of T c and T t do not coincide which can be attributed to the samples’ magnetic inhomogeneity. This type of inhomogeneity is attributed to the multiple peaks that cross over into the imaginary part of the measured susceptibility.
Table 4 Biaxial strain, Curie temperature, transition temperature, activation energy and electrical resistivity for LaMn1-xCoxO3 samples with x= 0.1, 0.2, 0.3, 0.4 and 0.5.
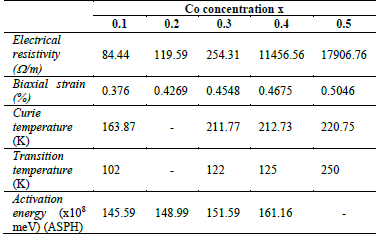
Source: The Authors.
In contrast, it is well known that there are remarkable differences in the magnetotransport properties for thin films and bulk materials which have been mainly been ascribed to biaxial strain induced by the substrate on the structure of the thin films owing to the differences in the lattice location (Lattice mismatch) between the film and substrate. In the case of magnetite samples with CMR, the coupling electron lattice plays a key role in understanding their electric and magnetic properties. The Jahn-Teller effect describes this coupling which is caused by biaxial distortions that allow the degeneracy levels of 𝑒𝑔 from the 𝑀n of a crystalline medium to be surpassed.
Table 4 indicates how the increase in the effects of strain produces a rise in the resistivity of the sample, i.e., the change in the constant lattice parameter can be explained by the small size of the cobalt ion in comparison to the Mn3+/4+ ion. In addition, a double peak structure is observed in the susceptibility measurements which is produced by a high concentration of cobalt which, in turn, could be related to the strain effects and domain wall motion.
As depicted in Fig. 9, the increase in Co concentration in LaMn1-xCoxO3 samples produced an increment of the biaxial strain ε bi , which shifts Tc to higher temperatures. Such shifts can be explained by the substitution of Mn (B-sites) with Co ions, which are smaller (radius of Co3+ = 0.54 Å , radius of Ni3+ = 0.56 Å, radius of Mn3+ =0.58 Å).
2.3. Effects of strain on LaMn 1-x O 3 magnetite samples
The behavior of LaMn 1-x Ni x O 3 samples at 0.1 < x < 0.5, in both magnetic and electric parts, was determined by taking magnetic susceptibility measurements (real and imaginary parts) and taking measurements of the resistivity as a function of temperature using the conventional four probe method.
All samples were characterized by taking measurements of X-ray diffraction (see Fig. 10), which shows an orthorhombic structure pbnm categorized within the 62 space group. The lattice constants a,b,c and the unit cell volume (V) were determined by Powder Cell software (see Table 5). As depicted in Fig 11, the lattice parameters decrease when exposed to the concentration of 𝑁𝑖, which can be explained by the lower ionic radius of nickel (ionic radius of Ni = 0.60, ionic radius of Mn= 0.80)
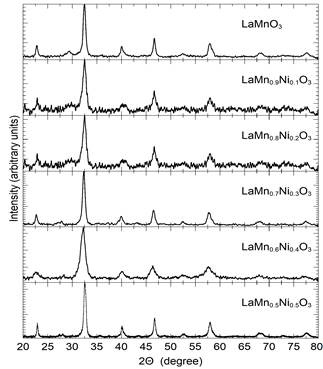
Source: The Authors.
Figure 10 The XRD patterns of the LaMn 1-x Ni x O 3 samples with x = 0.0 to 0.5.
Table 5 Lattice constants and unit cell volume of the LaMn 1-x Ni x O 3 samples with x = 0.0 to 0.5.
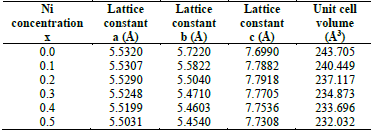
Source: The Authors.
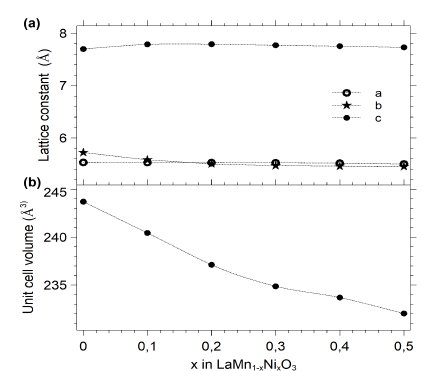
Source: The Authors.
Figure 11 Lattice constants (a) and unit cell volume (b) variation as a function of Ni concentration in LaMn 1-x Ni x O 3 samples. Dashed lines are visual guides.
The spectrum from X-ray diffraction obtained exhibits a single-phase system; impure phases were not observed according to the equipment’s indications.
Furthermore, the observed shifts are related to the different ion sizes of 𝑁𝑖. Using EDX spectroscopy, the analysis of chemical composition shows variations from the stoichiometry < 5 % and the concentration of 02 was not substantially affected (variations of less than 2%) by the heat treatments to which the samples were subject.
As depicted in Fig. 12, the real (a) and imaginary (b) parts of normalized ac-susceptibility are shown (X/X 0, and X/X 0 respectively) as a function of temperature for different concentrations of nickel.
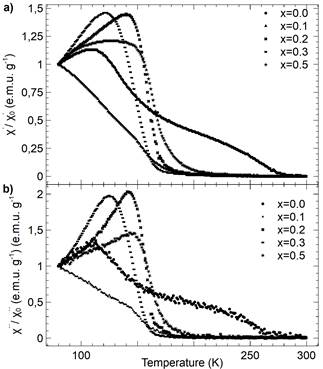
Source: The Authors.
Figure 12 (a) Real (X/X 0 ) and (b) imaginary (X/X 0 ) component of the normalized ac-susceptibility as a function of temperature for different compositions of LaMn 1-x Ni x O 3 samples (x = 0.0, 0.1,0.2,0.3 and 0.5).
The Nickel-doped samples exhibit a remarkable behavior-which can be attributed to their spin-glass state-that can be observed as a peak in ac-susceptibility measurements. This behavior could be explained as the result of the competition between the antiferro and ferromagnetic regimes. Similar situations have been observed in different compounds [25]. It is well known that the orbital antiferromagnetic state of 𝐿αMn03 is easily destroyed, resulting in a ferromagnetic state. Nevertheless, such ferromagnetic behavior could be the result of many other mechanisms that depend on the nature of substituted cations and substitutional levels [26].
The observed peaks that cross into the susceptibility measurements (X´/X´) have been attributed to double-exchange interactions of 𝑀n3+−𝑂2 2−−𝑀n4+,, which gradually vanish due to the interaction of an antiferromagnetic superexchange of the kind 𝑀n4+−𝑂2 2−−𝑀n4+ and 𝑁i4+−𝑂2 2−−𝑁i4+ [27].
Table 6 shows the transition temperatures of the insulated metal (T t ) and the magnetic transition (T c ) of the processes mentioned above. Although the transition temperature values do not explain any coincidence between the non-monotonic behavior and the concentration of Ni, all samples indicate a correlation between electric and magnetic phenomena. The general trend of different transition temperatures increases depending on the concentration of Ni.
Table 6 Biaxial strain, Curie temperature, transition temperature, activation energy and electrical resistivity for LaMn 1-x Ni x O 3 samples with x = 0.0 to 0.5.
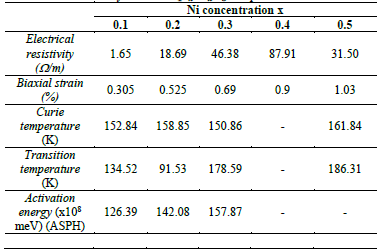
Source: The Authors.
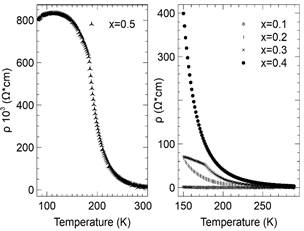
Source: The Authors.
Figure 13 Resistivity (() as a function of temperature for different compositions of LaMn1-xNixO3 samples (x = 0.1 to 0.5)
The corresponding resistivity as a function of temperature for all nickel-doped samples is shown in Fig. 13. A metal-insulator transition was observed. The transition temperature T t was determined by the maximum value of dR/dT vs. temperature. A significant increase in the resistivity as a function of the concentration of nickel was observed, and is depicted in the overlay of Fig. 10, which shows the resistivity of the sample that was a doped with 0.5.
The transition temperature (T t ) does not monotonically change when nickel is present, which correlates appropriately with the magnetic measurements (see Table 6). Nevertheless, T c and T t do not exhibit the same values, which is attributed to magnetic inhomogeneity. The inhomogeneity of the samples is characterized by multiple peaks in the imaginary part of the susceptibility [25].
The pseudo-perovskite RO3 at the A-site is available and the applied pressure produces an increase in the rigid octahedron rotation RO6. In the perovskite, the A-ion inhibits a possible collapse and the applied pressure decreases the rotation using the decrease in ionic radius mismatch which shows a denser packing of the 02 ion surrounding the ion at the A-site. Several papers that have studied the effects of pressure on PrNi0 3 samples and the results of crystallography of neutrons have confirmed that the octahedron Ni0 6 rotates less when pressure is applied; θ tends to 180° and the Ni- 0 bond is compressed.
In contrast, the measurements of resistivity as a function of temperature (see Fig. 13) are the outcome of the notable increase in resistivity in the samples as a function of the concentration of either Co or Ni. In addition, the results of ρ vs. T with T>T t (T t denotes the metal-insulator transition temperature) can be explained accurately at lower doping levels (x ≤ 0.1) by means of mechanisms related to adiabatic and non-adiabatic polaron hopping. Nevertheless, increasing the concentration of either Co or Ni, the hopping mechanism of variable range can also explain the experimental results obtained here at certain temperatures more accurately. At high doping levels (x ≥ 0.5), the experimental results describe an overall range of temperatures that fit properly into a mechanism explained as hopping at variable range.
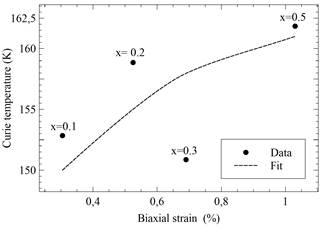
Source: The Authors.
Figure 14 Evaluated biaxial strain versus Curie temperature for different compositions of LaMn1-xNixO3 samples (x = 0.0, 0.1,0.2,0.3 and 0.5). Dashed lines are visual guides.
In the case of LaMn0 3 perovskite, the Mn ions fill the B-sites, which are surrounded by 02 octahedrons, meeting at the corners to form a tridimensional lattice, while the Lα ions fill the A-sites between these octahedrons. Electronic conduction involves the movement of charge between orbitals d in Mn and p in 0 2 ; the p - d orbital overlap is very sensitive to geometrical changes (angles and bond lengths) generated by variations of ion size at A-sites or external applied pressure.
3. Conclusions
The 𝐿α0.67−𝑥𝑃r𝑥𝐶a0.33𝑀n3 samples at 0.13 ≤ 𝑥 ≤ were produced by a solid state reaction method and exhibited a decrease in their lattice constants when the concentration of Pr was increased. This can be attributed to the different ionic radius size of the lanthanum and praseodymium, and the increase in “tensile” strain (εbi > 0). The increaset in biaxial strain causes a decrease in T c which is fitted to a quadratic function, i.e., it is possible to assume that the biaxial strain increases the Jahn-Teller splitting (JT) from e g levels and hence increases the localization of the electrons.
In the case of
samples at 0.0 ≤ 𝑥 ≤ 0.5, it was found that the adiabatic and non-adiabatic polaron transport mechanism accurately describes the outcomes of resistivity as a function of temperature. Furthermore, an increase in strain also increases the resistivity in the material, which can be attributed to the changes of the lattice constant related to the small size of the cobalt ion compared to the Mn3+ /Mn4+ ion.
In addition, in such samples it was possible to identify a double peak structure in the susceptibility measurements at higher concentrations of cobalt, which is related to the strains and the initial domain wall motion. The increase of T c and T t , as well as the Eα of polarons would suggest the important role of the electron-lattice interaction in this doped magnetite.
n the case of
a correlation between magnetic and electric properties was observed. The substitution of nickel and cobalt into the manganese sub-lattice increases the resistance of the samples and preserves the orthorhombic structure 0´. This behavior could suggest that the range of examined substitutional levels and the experimental conditions of synthesis, used for nickel and cobalt, exhibit a behavior of trivalent ion.
In addition, such samples show an increase in terms of the biaxial strains εbi, and their temperature T c , when the concentration of nickel is higher.