1. Introduction
In recent decades, great advances have been made in research regarding the development of new composite materials, in accordance with market requirements and the need for specific engineering applications [1].
Ceramic industry produces tons of solid waste resistant to chemical, physical and biological degradation, which end up in landfills daily [2-4]. In the United States of America alone, 145 million tons of construction waste are produced annually, derived from cooked bricks that are used in the manufacture or homes [4].
In the process of obtaining the clay products, furnaces are used that emit approximately between 70-282 g of CO2/kg of cooked brick. Similarly, cooked brick particulate material is generated and large amounts of energy are consumed [5]. As a result, the emission produced gradually increase the carbon dioxide footprint to the environment, and on the other hand, the manufacturing methods employed in this sector contribute to the increase in the energy crisis [4-6].
Consequently, numerous investigations have been carried out related to the reuse of industrial waste, implementing green building materials that promote sustainable development, in one of the most polluting sectors of the planet [2-6].
Tailings consist of grounded rocks and effluents from ore extraction process in mines. Some researchers investigated the viability of using tailing from hematite mine by mixing it with fly ash and clay to produce conventional fired bricks. It is found that, good quality fired bricks can be produced from these mixtures by moulding at a pressure of 20-25 MPa and water content of 12.5-15% for 2 hours in a brick kiln at a temperature of 980-1050 °C [5]. Several authors present this kind of waste or others of an organic nature, to be reused in alternative materials, which allow soil conservation, reduction of greenhouse gas emissions and increased energy efficiency [7].
Otherwise, the automotive industry is one of the most benefited sector with technological advances in design, due to the incorporation of composite materials [8]. Therefore, the high demand in the land transport vehicle marked, mainly focuses on reducing energy consumption, forcing the manufacturing of light elements from material such as aluminum alloys and polymer matrix composites [9].
In this research project, the mechanical properties of a thermosetting resin composite with particulate reinforcement of ceramic waste were determined, in order to analyze a possible application in the automotive sector. Initially, standardized specimens were manufactured from unsaturated polyester resin mixed with ground waste from the brick industry. Mechanical flexural tests were carried out under ASTM D790 standard, to subsequently validate the results by the finite elements method, in an application of a motorcycle brake lever. Likewise, the dispersion of the microparticles in the matrix and the fracture surface was analyzed by scanning electron microscopy. Finally, a prototype of the brake lever was development with the proposed material and the assembly was carried out on a sport-tourism motorcycle. In conclusion, this kind of clay-type waste is presented as a viable alternative of materials to be used in industrial applications.
2. Methodology
2.1. Materials
As a part of the composite, a high viscosity unsaturated polyester orthophthalic thermoset matrix was used, marked under the reference P-2002 by the company Industria de Resinas S.A.S. The manufacturer recommends the use of 2% p/p methyl-ethyl ketone peroxide (MEK peroxide) as a catalyst.
As a reinforcement, ceramic waste was used that comes from clay brick fragments, which were supplied by the warehouse of materials La Flecha, located in the municipality of Pamplona, Colombia. Initially, sintered clay powder with grain size (GS) less than 0.85 mm (< sieve #20) was obtained, processing the brick fragments in a ball mill.
Then, a sample of 5.37 kg of sintered clay powder (Fig. 1) was refined, under the conditions of ASTM C136/C136M, using sieves #40, #60, #80, #100, #140, #200, and the bottom [10].
Finally, the granulometry of 100 (m > GS > 150 (m (under sieve #100 and over sieve #140) was selected, which had a presence of 9.31% in the total of the sample, without apparent macrometric impurities. The sample was dried in an oven at 100 ° C for 24 hours, before being mixed with the polyester resin.
2.2. The manufacturing process
The manufacturing process used was cast molding, as shown in Fig. 2. Initially, the amounts of resin and reinforcement were weighed, taking as a reference a percent of 10% p/p of sintered clay powder and 2% p/p of catalyst. Later, mixing was done with the aid of magnetic stirring at a temperature of 80°C, in order to achieve optimum dispersion of the particles in the polymer matrix [11].
Finally, the contents in the molds were emptied and allowed to cure at 20°C for 7 days.
2.3. Flexion mechanical characterization
The three-point bending was executed in a universal testing machine SHIMADZU UH-600kN, following the conditions of ASTM D790 [12]. The dimensions of the specimen were 150x10x6 mm (length x width x thickness), with a length of 75 mm between supports (support span). The rate of crosshead motion R was 1.80 mm/min and was calculated with Eq. (1):
When, Z is rate of straining in mm/mm/min, L is support span in mm, and d is depth of beam in mm (thickness of material) [12].
In addition, the test was performed on five specimens of polyester resin (PR) and five specimens of the polyester resin composite with sintered clay powder (PR-SCP), for comparative purposes.
2.4. Scanning electron microscopy
The fracture surface obtained in the flexural test of the PR-SCP sample was analyzed using a scanning electron microscope TESCAN, model MIRA 3 FEG-SEM. The sample was metallized in gold plating to improve electrical conductivity.
2.5. Break lever simulation
The geometry of the pieces was drawn in Solidworks computer-aided design software, based on the dimensions of a sport-tourism motorcycle brake lever. These mechanical parts are made of aluminum casting or forged steel, and their function is to push the brake fluid to produce the braking of the front wheel. The mechanical requirements of these parts depend on the force exerted by the driver's hand [13].
An image of the rear lever is shown in Fig. 3a and Fig 3b corresponds to its virtual model (original CAD). Fig. 3c represents a modified CAD, which was necessary to redesign with respect to the original geometry, in order to facilitate the manufacturing process and meet the mechanical requirements by varying the assignment of material in the finite element method simulator.
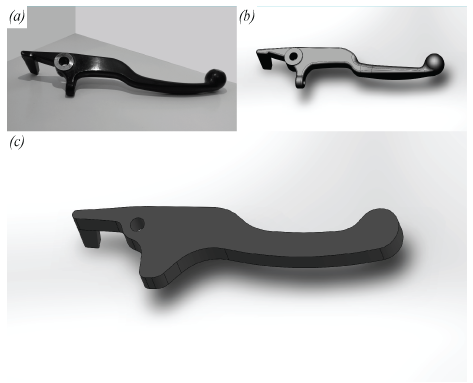
Source: The Authors.
Figure 3 Geometries: (a) Brake lever of sport-tourism motorcycle. (b) Original CAD. (c) Modified CAD.
Operating conditions for a brake lever were parameterized by real performance in limited circumstances, taking into account that the braking system is the most important component in vehicles to maintain the integrity of the passengers [14]. Consequently, it was assumed that the time needed to reach the maximum grip strength was less than 2 seconds, and the optimal values of maximum voluntary contraction are obtained within a period of 3 seconds [15]. Likewise, according to an anthropometric study conducted by Lee & Sechachalam (2016), a value of 320 N (32.60 kg) was taken as the grip strength maximum exerted by the dominant hand [16].
In accordance with the above, three simulation scenarios were proposed by the finite element method (FEM), taking into account the same operating conditions, to analyze the behavior in the design of a brake lever, with the help of the ANSYS R16 software:
Simulation 1: original CAD, assigning the properties of an aluminum alloy.
Simulation 2: original CAD, assigning the properties of PR-SCP composite.
Simulation 3: modified CAD, assigning the properties of PR-SCP composite.
For the three aforementioned cases, the material was assumed as homogeneous and isotropic. In addition, the mechanical properties of the aluminum alloy were taken from ANSYS Engineering Data (Young modulus of 71 GPa, yield strength of 280 MPa, and ultimate strength of 310 MPa), and those of the PR-SCP composite were taken from mechanical characterization to bending.
Table 1 shows the most important characteristics of the mesh for each of the geometries (CAD discretization).
Tetrahedral and hexahedral elements with average size of 1mm and curvature normal angle center of 15°, was used for mesh of original and modified CAD, respectively. Also, proximity and curvature advanced size function with relevance center and smoothing high, was implemented.
These parameters describe the mesh that converges to the solution, using the appropriate number of elements to reduce computational expenditure, with the Mechanical APDL solver.
The setup of the simulation parameters was performed as shown in Fig. 4. When, A is standard earth gravity of 9.81 m/s2, B is hand force of 320 N (32.60 kg), C is a frictionless support, D is a cylindrical support free tangentially, and E is an elastic support (5 N/mm3).
The simulated operating time was 10 seconds with an application period for the maximum grip force of 3 seconds, as shown in Fig. 5. The other parameters remained constant with respect to time. In each of the proposed scenarios, the mass of each geometry, the equivalent stresses (Von Mises) and the static load safety factor were determined, using Eq. (2) for the latter.
When, SF is safety factor of application,σ max is flexural strength of material in MPa, and σ equ is equivalent stress in Mpa (Von Mises).
The purpose of the simulation is mainly to determine the influence of the change of material and the geometry of the piece, in the mechanical behavior of the levers studied.
3. Results and analysis
3.1. Flexural properties
Fig. 6 and Table 2 show the results of the flexural test, where a linear-elastic behavior is evident in both materials. It is important to note that the nature of the thermoset matrix of PR is fragile and, therefore, the PR-SCP composite material adopts the same behavior.
However, the RP-SCP composite obtained a flexural modulus of 1.17±0.19 GPa, a flexural strength of 48.32±5.89 MPa, and a deformation to fracture of 7.52±1.28%. This demonstrates that sintered clay powder reinforcement reduces stiffness and fragility, and increases mechanical strength, compared to PR without reinforcement, which has a flexural modulus of 1.30±0.25 GPa, a deformation to fracture of 5.89±1.72%, and a flexural strength of 45.23±5.34 Mpa.
3.2. Analysis of morphology of PR-SCP composite
In Fig. 7 the fracture surface is observed characterized by cracks in different planes that form the river lines (black arrows), and corroborate the fragile behavior described in the destructive test [17,18]. In addition, two phases are distinguished: a continuous one that has smooth surfaces (thermosetting resin), and the sintered clay powder microparticles that are uniformly dispersed and wrapped within the matrix.
The micrograph of Fig. 8 shows big particles with irregular shapes, which have a size distribution that averages a value of 18.83±9.38 µm. It could be considered that these particles originate as a product of the union of finer grains during the manufacturing stage, giving rise to the formation of agglomerates that would act as stress concentrators, and consequently to the premature failure of the material.
In Fig. 9 the presence of finer particles of spheroidal shape with sizes ranging in average of 0.89±0.43 µm. These micrometric fragments have an optimal dispersion in the matrix, due to the good aspect ratio (l/d), whereby a greater interfacial area per unit volume is generated between the clay grains and the polyester resin, and by both is reflected in the mechanical properties.
3.3. Lever validation by finite element method
In Fig. 10 the values obtained in the simulations are shown, with respect to the distribution of stresses and the critical areas that are presented in each of the scenarios. It should be noted that the equivalent stress and the safety factor are directly related and are inversely proportional, as shown by Eq. (2).
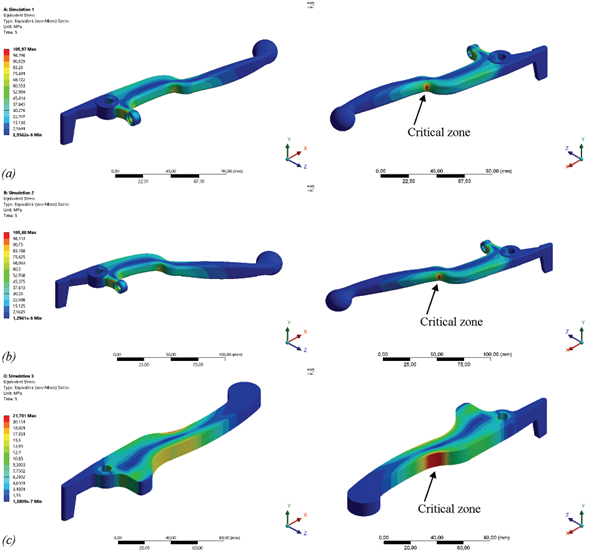
Source: The Authors.
Figure 10 Maximum stress distribution at t = 5s. (a) Simulation 1. (b) Simulation 2. (c) Simulation 3.
Table 3 shows the quantitative results of the simulations. In simulations 1 and 2, a similarity in stress distribution is evidenced, with maximum values of 105.97 and 105.88 MPa, respectively (Fig. 10a and 10b). However, there is a decrease in the safety factor, from 2.64 to 0.46, and a reduction in the mass of 44.51 g. Consequently, there is a decrease in the performance of the original part, when the properties of the PR-SCP composite are assigned, and therefore, it is not recommended to manufacture the lever with this geometry using the polyester composite material/10% waste.
On the other hand, in simulation 3, the modified geometry reveals a significant improvement in stress distribution, with a maximum value of 21.70 MPa (Fig. 10c). This result is directly attributed to the simplification of geometry and the increase in volume in critical areas. Accordingly, the minimum safety factor in the proposed piece reaches a value of 2.23. In accordance with the above, it was possible to validate compliance with the minimum mechanical requirements at static load required by the application, and additionally there was a reduction in the mass of 21.43 g, with respect to the original geometry made of aluminum alloy.
3.4. Manufacturing of brake lever
According to the results obtained in the simulations, the manufacturing of a lever prototype was made, taking as reference the modified geometry and the PR-SCP composite material proposed in this research project. The above was executed in three phases:
In the first phase the mold design was carried out in Solidworks, to later be manufactured in MDF (medium-density fibreboard). The layers were laser cut, in order to obtain accuracy in the geometry of the lever (Fig. 11).
In the second phase (Fig. 12), the mold was coupled and prepared, impregnating a thin layer of mineral oil on the walls. Then, the polyester resin composite material with 10% sintered clay powder was emptied into the mold, guaranteeing the complete filling of the material in the interior. Subsequently, it was allowed to cure for 7 days, under standard conditions at room temperature and pressure.
In phase 3, the piece was assembled, making adjustments for the final geometry, without apparent surface imperfections, and with options for improvement from the ergonomic point of view. Finally, the part was assembled on the motorcycle handlebar, correctly fulfilling its position (Fig. 13) and function. However, it is necessary to carry out exhaustive tests of the prototype, so that in the future its real mechanical behavior can be validated, and not only virtually.
4. Conclusions
In this research project, reinforced unsaturated polyester resin was combined with 10% p/p of ground brick waste. Bending tests were performed at three points to determine mechanical behavior. Subsequently, fracture surfaces were analyzed using the SEM. The data obtained in the flexural test was used to validate a finite element application, specifically an automotive part that drives the braking mechanism. Finally, a prototype of the brake lever was manufactured and the assembly was done on a sport-tourism motorcycle.
The flexural test revealed a linear-elastic behavior and fragile fracture by the RP-SCP composite. The SEM images of the fracture surface confirmed the fragility of the material, demonstrating that it is owing the nature of the thermoset matrix that presents the river lines, and not to the presence of ceramic particles that are uniformly dispersed and enveloped by the matrix. However, due to the presence of different granulometries, it is advisable to rigorously classify the grain size, in order to obtain greater uniformity of the material on a micrometric scale and improve its mechanical properties.
The results of the simulations showed a decrease in the performance of the levers, by making a change in the assignation of the traditional, material by the PR-SCP composite. However, by simplifying the geometry and increasing the volume of the piece, a better distribution of stress in the proposed piece was demonstrated and the use of the RP-SCP composite in it was validated, complying with the minimum mechanical requirements.
Particle waste from the brick industry is an important source of pollution for the environment. Therefore, in the present investigation they are shown as an alternative material to be used as reinforcement in polymer matrix composite materials, with industrial applications in the automotive sector. However, it is necessary to carry out exhaustive tests on automotive prototypes, in order to validate their real behavior.