1. Introduction
Landslides are considered to be a natural phenomenon that causes great human and economic losses worldwide [1,2]. Landslide susceptibility is controlled by natural factors such as geology, geomorphology, hydrology, and soil characteristics; but human intervention plays an increasingly important role in landslide occurrence, especially in anthropogenic environments such as cities where landslides generally cause fatalities, direct economic losses due to infrastructure damage, and indirect losses due to interruption of vehicular traffic between cities [1]. For this reason, it is important to study the occurrence of landslides in anthropic environments in order to understand the combined effect of natural and anthropic factors.
According to the statistics presented by Centre for Research on the Epidemiology of Disasters - CRED [3], there were 315 natural disasters around the world in 2018, of which 4.4% were caused by landslides with a total of 292 fatalities. In economic terms, and taking into account records dating back to the year 1900, the year 2017 presented losses of US$314 billion, being the second costliest year after 2011 [3]. On the other hand, although economic losses tend to be concentrated in industrialised and developed countries, human fatalities are greater in less-developed and densely-populated countries such as Colombia [4,5].
In Colombia, most landslides are located on the slopes of the Andes Mountains [6]. According to data recorded in the Disaster Effects Inventory System - DesInventar (www.desinventar.org), 30,730 landslides were recorded between 1900 and 2017, resulting in 34,198 fatalities. Previous studies carried out in Colombia reported a high correlation between landslides and precipitation [6]. The Andean region exhibits a bimodal pattern, with most landslides occurring during the rainy periods (March-May and September-November).
In 2016, the landslide in Colombia with the highest number of victims occurred on October 26 at 7:59 a.m. local time at Las Nieves quarry in the municipality of Copacabana, in the northern Colombian Andes. The landslide resulted in 16 fatalities and temporarily closed the highway between Medellín and Bogotá, which connects the two most important cities in the country. The approximate volume of material mobilised was 25,000 m3, to which existing excavation material along the slope was added with a total mobilised volume of approximately 40,000 m3.
This article presents the results of the technical investigation that was carried out at the slide site. The research methodology involved the evaluation of the local and regional geology and geomorphology, field visits, sampling of the failure soil, analysis of rainfall presented during and days before the event, and subsurface flows associated with regional infiltration processes. The information collected showed a regional subsurface water flow from recharge zones in the plateau east of the slide, which subsequently generated an increase in pore water along the contact between weathered rock and slope deposits, as the possible trigger of the landslide. Finally, a stability analysis (statistical analysis and sensitivity analysis) was performed using the limit equilibrium method, where the effect of the rise in the ground water table was modelled as the trigger.
2. Location and description of the landslide
The landslide site is located in the town of Copacabana, located on the north-western side of the central mountain range of the Colombian Andes. The municipality of Copacabana is part of the metropolitan area of the Aburrá Valley and the highway that connects the cities of Medellín and Bogotá passes through it. The landslide occurred at kilometre 12 of this highway on October 26, 2016 at approximately 7:59 a.m. local time, in the sector named Las Nieves quarry, where granular materials are excavated. The landslide began in the upper part of the slope, between the southern and northern banks of the quarry. Fig. 1 presents the location of the event and a photograph of the magnitude of the movement. The crown of the landslide presented a spoon shape with its middle portion adopting a slightly conical shape and the toe (accumulation area) was clearly wider, suggesting a high degree of water content in the sliding mass. The mass, initially solid, was transformed into a flow that buried the industrial facilities of Las Nieves quarry, both lanes of the Medellín-Bogotá highway, and part of a restaurant located next to the highway.
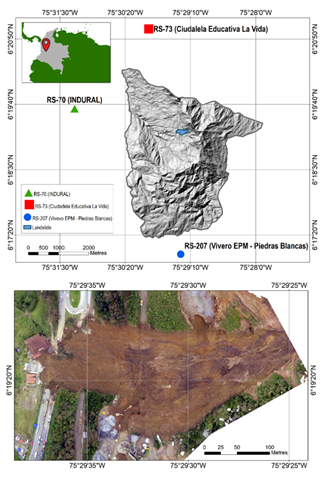
Source: The authors.
Figure 1 Location of the landslide in Las Nieves quarry, municipality of Copacabana, Colombia.
The calculation of the slip volume was estimated by taking field measurements in the slip area. The crown and toe of the landslide were measured, as well as the distance from the toe to the crown. Finally, to calculate the volume, the thickness of the deposit (the soil that failed) was considered which varied between 3 and 5 meters. The thickness of the failure was corroborated with a field visit, and an average volume of approximately 25,000 m3 was obtained. Fig. 2 presents an image of the quarry before the landslide; this figure shows the effect of anthropic intervention due to extraction of material from the quarry which increased the slope of the terrain considerably.
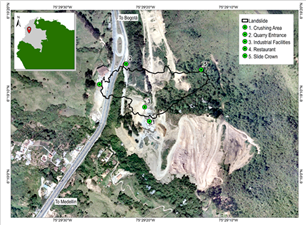
Source: The authors.
Figure 2 Location of reference sites in the area of the landslide in Las Nieves quarry, municipality of Copacabana, Colombia.
2.1 Setting of the study area
The database DesInventar is a compilation of disasters that have occurred in the Aburrá Valley since 1880, associated with phenomena of geological, hydrological, and anthropogenic origins. The landslides database compiles 2,046 records, which were used to evaluate the historical relationship between the occurrence of disasters in the region and the rainy season. Fig. 3 shows the number of recorded events compared to the average monthly precipitation in the Aburrá Valley. It is important to mention that the first record of a landslide was registered on October 22, 1921 in the city of Medellín.
The data are consistent with previous studies conducted in the region [7], which indicate a high correlation between the occurrence of landslides and the rainy season. Fig. 3 shows that the highest number of landslides occurred in the months of May and October, with values of 303 and 329, respectively. In contrast, January and February showed the lowest number of landslides, with a total of 56 and 70, respectively.
Additionally, from Fig. 3, it is important to observe that most of the landslides occurred at the end of the rainy season when there is higher water content in the soils, which may reflect the effect of antecedent rainfalls on the generation of a greater number of landslides in the months of May and October.
2.2 Rainfall analysis
Rainfall is one of the most common triggers of landslides. However, the failure mechanisms are complex and involve different factors that influence the hydrological behaviour of the slope, as well as aspects related to shear stress and soil strength along the fault surface [8]. Landslides associated with rainfall occur due to the rapid increase in pore pressure within the subsoil [9,10] or due to the loss of soil suction [11]. For the analysis of the landslide in Las Nieves quarry, data from the rain station network of the Environmental Early Warning System (SIATA), specifically stations RS-70 (Indural), RS-73 (Ciudadela Educativa La Vida), and R-S207 (Vivero EPM - Piedras Blancas) were used. The information from these rain stations comprises the rainfall intensity data between the years 2010 and 2016. The location of these stations is shown in Fig. 1 (RS-70, RS-73 and RS-207).
Fig. 4 shows the analysis of hourly rainfall intensity for the selected stations, from 21-26 October 2016. From this figure, it is seen that RS-207 presented a total accumulated rainfall of 125.9 mm for the five days prior to the slide, with a rainfall duration concentrated in the early morning of October 25, one day before the landslide, with a maximum hourly rainfall of 43.3 mm. This condition reflects the reduced presence of rain during the five days prior to the landslide and the dispersed distribution of significant rainfall during the 24 hours prior to the landslide. At RS-70 and RS-73, the total accumulated rainfall was 17.5 and 50.9 mm, respectively, which occurred during the five days prior to the event, with a maximum hourly rainfall of 12.4 and 28.8 mm, respectively. At these stations, the duration of the events was concentrated, as at RS-207, in the early hours of October 25. None of the stations analysed in this study indicated that rainfall occurred at the time of the landslide. This information was corroborated by personal interviews with people that were at the quarry at the time of the slide.
2.3 Geology
The study area is located in the Central Cordillera of the Colombian Andes, formed by a metamorphic basement of Permo-Triassic age [12] and intruded by Cretaceous plutons. In the northern part of the study area, there are amphibolites that are moderately-fractured in the north and highly-fractured in the south. The weathering profile of the amphibolite does not show residual soil development, and very locally, a layer of limy clay saprolite less than 1 m thick was observed, whose colour varied from light cream grey to yellow brown. It is important to emphasise that the degree of weathering in the rock varies between I and III according to the degree of fracture according to Dearman [13]. In the study zone, a transitional contact with gneissic texture type rock was observed, with well-defined quartz and mica (QM) bands that vary in schistosity depending on the degree of fracturing.
Locally, very pronounced folds and highly-sheared zones with quartz veins were observed. In the weathered profile, most of horizons V and II were present, while the other horizons (III, IV, and VI) were absent or underdeveloped [13]. The saprolite is of silty sandy granulometry. The foliation is subparallel to the slope with a tendency in strike and dip N10W/50W, with variations of EW/subvertical to the north and N50E/30SE. The joint patterns in the gneiss show a continuity of up to 2 m, density of up to 8 diaclases per metre, openings from 1 to 8 mm (with those of 3 mm being more common), and very rough side walls filled with clays. The main families are N45W/74NE and N65E/77NW.
Much of the area is covered by slope deposits with complex spatial and temporal relationships contextualised in the geological map. The slope deposits located in the failure zone correspond to a talus type deposit and that corresponds to deposits formed mainly by the action of gravity. These deposits produced accumulation of angular rock fragments with scarce or absent matrix in areas of steep slopes (Fig. 5). The average thickness of the talus is on the order of 2 metres. There are also rocky materials in the area that can be mapped at a working scale, but whose origin is due to anthropogenic intervention and excavation of quarry material.
2.4 Geomorphology
From the geomorphological point of view, it was found that more than 70% of the study area is composed of slope deposits, the multitemporal and spatial analysis of the area of interest allowed us to identify and map three series of past landslides that were grouped to be presented. These show a direct relationship with the intense denudation processes occurring in the Aburrá Valley. The first and most relevant is a huge relict landslide complex named, El Cabuyal, located in the central part of the area, which includes Las Nieves quarry. The second denudation process corresponds to a relict landslide complex namely, Las Peñas, located in the north near the southern portal of the tunnel. The third landslide complex is called Las Nieves. These landslides complexes show at least three phases of activity. The most recent phases correspond to active and/or latent landslides (Fig. 6).
During the study, at least two types of major mass movements have occurred: landslides and rock falls. More than 150 previously unstable areas were inventoried that make evident the occurrence of landslides in the area. These were classified as active, latent, and inactive according to the criteria of Cruden and Varnes [14], based on multi-temporal analysis, spatial analysis, and field data, before and after the landslide of 26 October 2016.
About 75 % of the crowns are related to landslides of rotational or translational types that occur where there are slope deposits, while about 25% correspond to landslides caused by falling rocks. Thirty-two per cent of the mapped crowns are active and concentrated on the upper edge and on the rock scarp, while the latent crowns are concentrated on the rock scarp and in areas with slopes greater than 30° and correspond to 37% of the movements. Finally, the inactive crowns correspond to approximately 31% of the movements and are located predominantly on the sliding cones in the lower part of the study area, where debris flows outcrop.
2.5 Hydrogeology
To understand the effect of groundwater on landslide processes, it is necessary to recognise that water moves through a medium formed by the soil and the underlying rock mass, and that the movement of groundwater from the infiltration zone to the discharge zone is an integral part of the hydrological cycle. It is therefore important to identify how surface and infiltrated water reaches the subsoil on steep slopes and how it contributes to the generation of landslides in order to refine methods of analysis of slope stability.
From the hydrogeological point of view, the study site is located in a regional recharge zone [15], with a predominant geology of igneous and/or metamorphic rocks and an impermeable matrix; the hydraulics are controlled by the secondary permeability of the rock mass, which is characterised by low water-storage capacity. The study area is located in a mountainous system with highly-fractured rocks which play an important role in the flow of water that depends on recharge levels, transit points, and discharge sites.
In order to understand the underground flow system, geological, geomorphological, and hydrological information, as well as an inventory of water discharge points were used as part of the research work. The infiltration recharge zones are associated with low slope areas which correspond to the geomorphological unit known as the plateau. These areas are considered potential infiltration zones which in theory do not contribute to surface runoff. On the contrary, all rainfall inputs are converted into recharge of the system that moves the flow through the discontinuities present in the rock mass.
Fig. 7 presents the location and inventory of surface water sources. Due to the importance of understanding the processes that originated the Las Nieves quarry landslide, emphasis should be placed on the quarry gauging points (AM-1, AM-2, and AM-3), which roughly represent the position of the ground water table in the zone of immediate influence on the landslide. These points, together with the springs at EA-1, EA-2, and EA-3, represent the vertical oscillation band of the ground water table in the area of Las Nieves quarry. Specifically, point EA-2, located in the escarpment of the landslide, was gauged in the days following the landslide and yielded a flow of 3 L/s which gradually decreased over the following weeks until it stabilised at approximately 1 L/s between January and February 2017.
In addition, an isobase analysis was performed in which the intersections of the first- and second-order drains were related to the position of the base level according to the methodology described by Grohmann [16]. The point of intersection is an indicator of the piezometric level, which is part of the contribution to the base flow that in turn maintains the surface flow of the drains found in the area studied.
In order to evaluate the influence of the geological structures of the rocky massif on the slope hydrology, a structural geological survey was carried out with a total of 322 data of strikes and deps, of which 80 were taken in amphibolite and 242 in gneiss. The data were processed and analysed statistically to determine the predominant families of discontinuity in the area. Based on this analysis, it was possible to verify that part of the flow that is recharged in the plateau zone, transits through the discontinuities of the gneiss lithological unit, and is discharged along the escarpment that separates the Aburrá Valley from the plateau. The intense fracturing of the gneiss was verified by the mechanical characterisation of the discontinuities. The discontinuities facilitate the movement of the groundwater flow as a function of the roughness, width, and continuity, among other characteristics, of the structures [17]. Additionally, the results show that the amphibolite is less fractured, so its secondary permeability is lower compared to the secondary permeability of the gneiss.
In the study area, the nearly vertical dip of the gneiss favours deep water flow in this rocky mass. By combining the sets of discontinuities, it is possible to establish a network of planes with north-west orientations which favours the hydraulic connection between the plateau and the failure zone (Fig. 8).
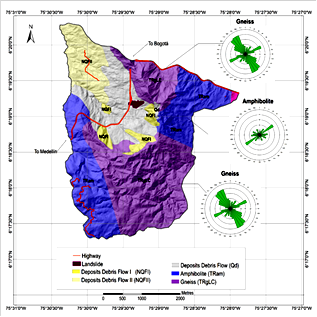
Source: The authors.
Figure 8 Directions of discontinuities for the two different lithological environments found in the landslide zone.
Based on the information described above, the hypothesis for the triggering factor of the landslide, is that the water from antecedent precipitation recharged the plateau zone, predominantly at sites of low slope and in the presence of residual soil, thus favouring processes of water infiltration and retention. Consequently, the infiltrated water was conducted through the discontinuities existing in the gneiss, to the slopes where the landslide occurred. The water that infiltrated through the discontinuities in the gneiss accumulated over the amphibolite, causing an increase in the water level at the failure zone. A diagram of the hydrogeology is presented schematically in Fig. 9.
2.6 Geotechnics
Five boreholes were drilled in the colluvial deposits where the landslide occurred, and soil samples were classified and tested for mechanical strength. The results of the soil classification are presented in Table 1; those of mechanical strength in Table 2. The soil presents a unit weight (Y m ) of 16,6 kN/m3, a specific gravity (Gs) of 2,73 and a porosity (n) of 50%. For the determination of the strength parameters, the following considerations were taken into account:
Parameters obtained from direct shear tests on field samples in the laboratory: Several soil samples were taken in the field from the soil that made up the sliding mass. Due to the heterogeneous nature of the colluvium, the samples did not offer the necessary quality for the execution of the direct shear test. Only one sample could be properly tested. The rest of the samples were used for soil classification.
Parameters obtained from existing literature: Strength parameters were assumed for soils with similar characteristics and previously reported by Whitman and Bailey [18].
Parameters obtained using Hoek-Brown (H-B) and Barton criteria: In order to obtain a greater number of strength parameters, the GSI (Geological Strength Index) method presented in Marinos et al. [19] was used, in which shear strength is represented as a curvilinear Mohr envelope. The criterion takes as its starting point the properties of the intact rock and then introduces factors to reduce or degrade these properties based on the characteristics of the discontinuities in the rock mass. Although initial field observations indicated that the failure material corresponded to a slope deposit; for merely geotechnical effects, the deposit can be assimilated to an intensely fragmented and weathered rock mass. This assumption has proved useful in several applications within the local geological environment, especially when accompanied by values obtained from laboratory tests and literature. Applying these strength criteria, the failure envelopes were projected to estimate the friction angle and cohesion of the soils analysed.
With the strength parameters obtained, assumed, and estimated, the average values for cohesion and friction angle were calculated (Fig. 10). In addition, the standard deviation (SD) of the parameters and their coefficients of variation were calculated. Finally, a region determined by the mean values of cohesion and friction (± SD) of the strength parameters was obtained, from which the stability analyses were performed.
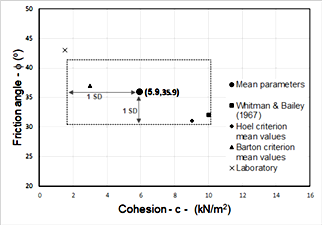
Source: The authors.
Figure 10 Region of variability considered for the cohesion and friction angle values used in the stability analysis.
Taking into account the information collected in the study area, we proceeded to establish the boundary conditions and the possible failure model. Colluvial deposits were found in the fault zone, and the amphibolite and gneiss units identified in the geological survey were located at depth. The topographic and geological profile along the central axis of the slide is presented in Fig. 11.
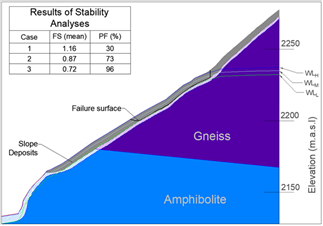
Source: The authors.
Figure 11 Profile along the central axis of the slide and position of the water levels
From the hydrogeological information collected, it was specifically shown that at point EA-2 (Fig. 7), located on the scarp of the landslide, there was a discharge of water in the days following the landslide, with a flow of 3 L/s, which gradually decreased in the following weeks until it stabilised at approximately 1 L/s between January and February 2017. This allowed us to propose a scenario in which the landslide was caused by a rise in the ground water table as a result of the infiltration of subsurface water from the plateau, favoured by the degree of fracture and the greater secondary permeability of the gneiss with respect to the amphibolite, as shown in the diagram in Fig. 9.
According to the information presented above, and from the values of the mechanical strength parameters and their variation (Fig. 10), a limit equilibrium statistical analysis was performed to determine the effect of the ground water table on the overall stability of the analysed slope. For the analysis, the SLIDE program [20] was used. For the analysis, normal distribution functions for cohesion and friction were assumed according to the mean values (± SD) established in Table 2. Three cases of groundwater-level positions were analysed, as shown in Fig. 11, and the results are described below:
Case 1: ground water level position (WLL). The position of the ground water table at the boundary between the colluvial deposit and the rock base is assumed. For this case, a mean safety factor of 1.16 was obtained with a probability of failure of 30%.
Case 2: Middle ground water level position (WLM). It is assumed that the ground water table is located at a height equal to half the thickness of the colluvial deposit. In this case, a mean safety factor of 0.87 was obtained with a probability of failure of 73%.
Case 3: High ground water level position (WLH). A phreatic level is assumed which mostly saturates the colluvial deposit. In this case, an average safety factor of 0.72 and a probability of failure of 96% were obtained. For all cases, the failure surface was naturally located along the contact between the rock base and the colluvial deposit as shown in Fig. 11.
Due to the uncertainty of the strength parameters, a sensitivity analysis was performed to identify all possible combinations between the friction angle and the cohesion of the soil that would produce safety factors equal to 1. The results of this analysis are presented in Fig. 12. In this figure, the dots represent the combinations of cohesion and friction that result in safety factor values between 0.95 and 1.05 for the three water levels considered (Case 1, 2, and 3). The continuous lines between the dots show the trend of the combinations of the strength parameters indicating that the greater the available friction angle, the less cohesion is required to reach the safety factor of 1, and vice versa. Any combination of the strength parameters below these lines indicate safety factors less than 1, for each of the cases studied.
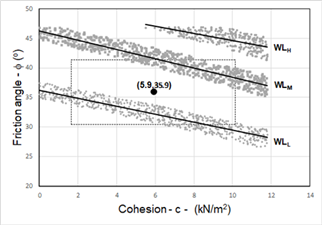
Source: The authors.
Figure 12 Results of the sensitivity analysis of the ground water table position on slope stability.
Fig. 12 shows a shaded rectangle within the estimated parameters used for the analysis are located as explained above. The comparison of the assumed parameters for the soil and the results of the safety factors equal to 1 for the three cases studied allowed us to conclude the following:
Case 1: WLL. In this case, there is a large area above the trend line that would indicate the possibility of combinations of fiction angle and cohesion that generate safety factors greater than 1. However, some combinations of these strength parameters could generate safety factors below 1 (area of the rectangle below the line).
Case 2: WLM. In this case, the vast majority of parameter combinations results in safety factor values less than 1, indicating a high probability of failure.
Case 3: WLH. In this case, all possible combinations of strength parameters produced safety factors less than 1, which indicate the impossibility of mass stability under these conditions.
The overall result presented in Fig. 12 indicates low initial stability conditions in the area studied (Case 1), with a considerable reduction in stability for cases in which the ground water table rises in the soil (Cases 2 and 3).
3. Discussion of the causes and mechanisms of failure
From the analyses presented in this document along with the other aspects studied, the following factors are considered as contributing to the Las Nieves landslide:
Anthropogenic intervention associated with the extraction of material from the quarry and the construction of access roads for the extraction machinery after the year 2013 were observed. These processes led to a considerable decrease in the already low stability of the slope deposits over the metamorphic rocks on steep terrain. Additionally, the surface drainage was altered because the road was constructed without any water drainage channels. The results of the stability analysis showed a safety factor close to 1, even for the lowest water level (WLL), indicating a low stability condition.
The local geological setting shows the presence of slope deposits of different types (debris and sludge flows, talus, recent sludge flows, landslide flows) on the slope. The type of soil found in the matrix of the deposit that failed corresponds to material of granular domain with low content of fines, which, in turn, present low cohesiveness. These soils correspond to unconsolidated deposits that are affected by increases in saturation due to increases in the ground water table as a result of direct and indirect infiltration by rain. The increase of water levels in the soil reduces the effective stress as well as the shear strength of the material.
Numerous morphodynamic processes characterised by various relic and dormant landslides were identified, showing the dynamics of denudation processes in this zone. Analysis allowed us to conclude that the studied slope presents a high level of landslide susceptibility, some of great volume as shown in the geomorphological map located immediately to the south of the area where the Las Nieves landslide occurred.
As for hydrological information, there is no evidence of rainfall occurrence on the day of the landslide at Las Nieves quarry. Prior precipitation in the plateau region east of the studied slope is the only factor that can explain the presence of persistent discharge of water on the slope occupied by Las Nieves quarry.
The conceptual hydrogeological model indicates that precipitation on the plateau easily infiltrates the intensely fractured gneiss, a lithological unit that outcrops in the upper part of the failed slope, facilitating an underground flow that moves preferentially towards the north-west. Subjacent to the gneiss is the amphibolite unit, clearly less fractured and therefore with less secondary permeability, in which the flow is delayed causing a localised increase in the phreatic level and permanent discharge of water evidently controlled by the gneiss-amphibolite contact.
The rainstorms of at least three days prior to the landslide occurred in the plateau, and rains of the previous weeks, in the same region, promoted the rise of the ground water table in the slope that occupies the quarry to the point of reaching the colluvial deposits located, as a superficial cover, over the fractured and weathered gneiss.
4. Conclusions
The purpose of this investigation was to discover the cause and failure mechanism of the landslide that occurred on October 26, 2016 near the municipality of Copacabana, Colombia. The research methodology involved field visits, sampling of the failure soil, study of the geology and geomorphology of the area, analysis of rainfall during and days before the event, and subsurface flows associated with regional infiltration processes in order to conduct a stability analysis and corroborate our hypothesis concerning the triggering factor. Based on the data obtained in the investigation and the results of the stability analysis, the following conclusions can be drawn from the study:
The geology of the area showed that the landslide occurred along the border between the existing slope deposits and the contact between the fractured gneiss and the less-fractured amphibolite. This stratigraphic condition could be decisive for the generation of the slide.
From the hydrogeological information collected, it was shown that near the escarpment zone of the landslide, there was discharge of water during the days following the landslide. This allows us to propose a scenario in which the landslide was caused by the rise in the ground water table as a result of the infiltration of subsurface water from the plateau, favoured by the degree of fracture and greater secondary permeability of the gneiss with respect to the amphibolite.
The statistical stability and sensitivity analyses were performed by assuming that the variation in the ground water table, resulting from subsurface water infiltration, validates the nearby conditions of mass instability even when a low ground water table is assumed, as well as the decrease in stability as the ground water table rises.
From the geomorphological point of view, evidence was found in the study area of numerous morphodynamic processes characterised by different landslides of different ages. These processes were duly located and constitute the evidence that allows us to conclude that the studied slope naturally presented a high level of susceptibility to the occurrence of landslides.