1. Introduction
The life expectancy of humans and the world population are continuously increasing in time, giving rise to more abundant people with bone diseases such as osteoporosis, bone cancer, bone fractures, and bone infections [1-3]. As each case may be different, it is necessary for orthopedists to have a broad assortment of materials for a more personalized treatment. Several calcium phosphate (CaP) biomaterials are currently used as bone replacement materials in orthopedic or dentistry surgeries [4-6]. Among them, calcium phosphate cement (CPC) presents interesting characteristics like biocompatibility, osteoconductivity, injectability, in vivo self-setting, and the capability to incorporate any kind of active principle (proteins, growth factors, DNA, or drugs). All these characteristics are mainly given by the chemical composition (which is similar to the mineral component of the bone) and by the easy handling of the paste formed when the precursor, alpha tricalcium phosphate (α-TCP), is mixed with an aqueous solution [7-8]. Although CPC is widely used in bone treatment procedures, the research on new CPC formulations is continuously performed.
However, the in vivo behavior of the CPC might be compromised by surgical site infections that lead towards Osteomyelitis. Such disease is mainly caused by microorganisms like Staphylococcus aureus (S. aureus), which adheres to the biomaterial, grows a defined biofilm, and finally promotes an infection that may persist and proliferate to nearby tissues. A biofilm is a 3D structure formed by microorganism aggregates’ excretion of extracellular polymer substances (EPS), establishing a safe environment that protects these microorganisms from external chemical components and mechanical stresses [9-12]. One crucial step in the development of a high antibiotic resistant Osteomyelitis is the biofilm formation onto the material [13,14]. Thus, it is important to study how thickly and homogenously it grows during the first hours after the implant is colonized by the microorganisms so that future strategies can be proposed for prevention of this phenomena. One recent method for measuring biofilm thickness is by optical coherence tomography (OCT), which is an in situ, non-destructive, non-contact characterization technique that enables researchers to obtain a transversal image of the material and the grown biofilm without any contamination of the sample [15,16].
CPC is a biomaterial that can be obtained from TCP; also, CPC is in constant research due to the formation of calcium deficient Hydroxyapatite (CDHA) in a physiological medium, mimicking bone mineral component. Although there are some works that analyze CPC properties’ change due to different liquid-to-powder (L/P) ratios [17,18], no surface adhered S. aureus biofilm thickness is reported; neither is any relationship between the microorganism thickness and the CPC physicochemical properties reported.
This work aims to analyze the effect of the calcium phosphate cement liquid to powder ratio over its physicochemical properties (microstructure, specific surface area, rugosity, chemical bonds, compression, and flexural strength) and how these are related to the Biofilm thickness of two cement adhered S. aureus strains, one EPS producer and the other non-EPS producer.
The understanding of the relation between microorganism and substrate characteristics in the S. aureus biofilm formation on calcium phosphate cements would help future works to incorporate new physicochemical strategies to avoid biofilms on ceramics used as bone graft. In addition, the biofilm thickness analysis shown here is a starting point for the efficacy evaluation of CPC loaded with antibacterial agents.
2. Materials and methods
2.1. α-TCP synthesis
α-TCP was synthesized via high temperature solid state reaction by the mixture of calcium carbonate (CaCO3 Merck, Germany) and calcium hydrogen phosphate (CaHPO4 Sigma-Aldrich, USA) at a 2:1 molar ratio. It was prepared with a similar protocol presented by Pastorino et al [19], briefly, 16.13 grams of CaCO3 and 43.87 grams of CaHPO4 were mixed in a vertical shaker at 750 RPM. Sequently, the mix was poured into a platinum crucible and heated inside a furnace to 1400ºC, sustained at said temperature for 2 hours, then quenched by air jet and assisted by crushing with a stainless-steel hammer to enhance the powder surface area for cooling. Finally, the α-TCP obtained was milled in a 250 ml zirconia ball mill (S 100 centrifugal ball mill, Retsch GmbH, Germany) using four zirconia balls (d=40 mm) for 25 min at 400 rpm. The α-TCP powder was sieved through a 37 µm hole size mesh (#400 mesh, Pinzuar LTDA, Colombia). To guarantee the α-TCP powder was the desired one, X-ray fluorescence (XRF; Axios WDXRF, PANanlytical B.V., The Netherlands) was used to analyze the chemical composition and to calculate the calcium to phosphorous molar ratio (Ca/P). X-ray diffraction (XRD; Empyrean, PANanalytical B.V., The Netherlands), with a copper radiation source (Cukα, λ = 1.540598 Å), operated at 45 kV and 40 mA was used to determine whether α or β-TCP phases were present on the precursor powder in order to determine the goodness of the thermal process to obtain α-TCP. The XRD pattern was compared with the Joint Committee on Powder Diffraction Standards (JCPDS) 9-348 and 9-169 for the α and β phases, respectively, in the range of 2θ = 20-40º. In addition, Fourier Transformed Infrared Spectrometry (FTIR-DRIFT, IRTracer, Shimadzu, Japan) was developed to ensure the material presented only tricalcium phosphate related ions.
2.2 CPC synthesis
To obtain three different CPCs, α-TCP powder was mixed with distilled water at three different liquid-to-powder ratios (L/P) of 0.33, 0.44, and 0.55 ml/g, noted as C3, C4, and C5, respectively. The agitation of these two components was carried out continuously for one minute prior to molding. Samples for electronic microscopy analysis were poured in Polyethylene cylindrical molds (d=12.0 mm, h=6.0 mm) for compression (d=6.0 mm, h=12.0 mm) and flexural (w=4.0 mm, t=3.0 mm, l=45.0 mm) tests. Teflon molds were used according to ASTM standards C1424 and C1161, respectively. When visual cohesion was achieved, each sample was immersed on Ringer’s solution at 37ºC for 7 days to accomplish the setting reactions. Finally, samples were dried at 60ºC for 24 hours and stored in a desiccator. For the analysis of biofilm, formation samples C3, C4, and C5 were poured and molded in a 24 well plate and immersed in Ringer’s solution as previously explained. CPC XRD was obtained with the same characteristics explained for TCP, comparison was made against Hydroxyapatite standard JCPDS 9-432. The SSA was determined by nitrogen (N2) sorption using a Gemini V2.00 (Micromeritics, USA), and samples for this test were casted as cylinders (d=6.0 mm, h= 4.0 mm) and degassed in a nitrogen atmosphere at 120ºC for 12 h. The SSA analysis is reported using Brunauer-Emmett-Teller (BET) theory. The morphology and homogeneity of the three CPC was analyzed through a scanning electron microscopy (SEM; JSM-6490LV, JEOL Ltd, Japan) to determine whether the formation of calcium deficient Hydroxyapatite (CDHA) crystals was accomplished. Surface roughness was measured by profilometry (Surtronic S100, Taylor Hobson, UK) over the samples for flexural test; measurements were done over the face of the sample that was not in contact to the mold during the process. Both compression and flexural test were performed in a universal testing machine (AGS-X 50 kN, Shimadzu Ltd, Japan) with a head speed of 0.5mm/min.
2.3 S. aureus strains
Two S. aureus strains were used in this work: an EPS producing strain (S. aureus ATCC 25923) and a non-EPS producing strain (5298 isolated from a patient with an implant-related osteomyelitis at the University Medical Center Groningen, the Netherlands) [20]. Both S. aureus strains were thawed from -80ºC to room temperature and incubated on Blood agar plates at 37ºC in anaerobic condition for 24 h. Next, an isolated colony was taken and precultured in 10 ml of tryptone soya broth (TSB; Oxoid Ltd, Basingstoke, England) at 37ºC, anaerobically as well, for 24 h. This preculture was used to prepare a main culture of 100 ml in TSB and incubated for another 16 h at 37ºC.
For the biofilm growth over the CPC, the S. aureus main culture was sonicated (3 X 10 s), to break bacterial aggregates, and re-suspended in TSB to a concentration of 5 X 108 bacteria/ml, which was guaranteed by using a Bürker-Türk counting chamber. Later, for bacteria incubation, 1.5 ml of this culture was gently deposited over each CPC sample, and as controls, two empty polystyrene (PS) wells were filled, one with the EPS producing and the other with the non-EPS producing strain. The other empty wells were filled with distilled water to guarantee a good humidity level and to avoid culture evaporation. After 2 h, supernatant was replaced by 1.5 ml of fresh TSB, incubated for 16 h at 37ºC, and covered with aluminum foil to prevent liquid from evaporating. The test was performed under static conditions.
2.4 Biofilm analysis
Optical coherence tomography (OCT) images were taken using an OCT GANYMEDE-II (Thorlabs, Germany) at 24 h of incubation. Two images per sample were taken. When running the test, supernatant is replaced by sterile phosphate-buffered saline (PBS) at pH 7.4 for diminishing interference in image acquirement. OCT is an in situ, non-destructive characterization technique [15,16].
The OCT images were processed using open source software imageJ (version 1.51K, National Institute of Health, Bethesda, MD, USA). For biofilm thickness calculation, 10 measurements of each image were carried out. First, the bright bottom line was determined as the CPC surface and the white continuous dots above as the S. aureus biofilm. Next, the measurement lines were done from the baseline until the last dot considered present in the biofilm. The lines are perpendicular to the CPC surface at the measuring point. As the three CPC were manually casted in a 24 well plate, no flat cement surface will be observed on OCT images, as it will be seen in PS control.
3. Results and analysis
3.1 Calcium phosphate powder characterization
The XRF analysis of the synthesized calcium phosphate presented a purity of 98.77% as well as a molar Ca/P ratio equal to 1.49, consisting of 53.37±0.21 wt.% of CaCO3 and 44.79±0.30 wt.% of P2O5. The calcium (Ca) and phosphorus (P) elemental composition and the molar Ca/P are presented in Table 1. This result is in accordance with the theoretical molar Ca/P ratio values of 1.5 for tricalcium phosphates [4].
Table 1 Tricalcium phosphate elemental chemical composition and Ca/P molar ratio.

Source: The authors
As shown on Fig. 1, the sample XRD diffractogram was compared against the JCPDS 9-348 and 9-169 patterns, and mainly α-TCP peaks were identified in the material diffractogram (Fig. 1). Only one peak at 27.89° was attributed to β-TCP. In accordance to other authors this small impurity may be attributed to small quantities of magnesium, present in the reagents, which stabilizes the β polymorph [21-23] or to a non-fast enough cooling rate, as stated in some documents [24,25]. However, the high α-TCP purity indicates that this precursor will promote an apatite-like calcium phosphate cement if pH during setting is higher than 4.2 [23,26]. These results show that the thermal process used was adequate for obtaining α-TCP.
3.2 Calcium phosphate cement characterization
Fig. 2 shows the FTIR spectra of the thermal obtained TCP and of the three CPC. It is possible to see how the α-TCP transforms into the cement after 7 days of setting in Ringer’s solution at 37°C. Nevertheless, when comparing the three different L/P ratios cements among them, no difference can be noticed. The α-TCP spectra show two big peaks associated only with phosphate ion (526, 556, 955, 989, 1019 cm-1); on the other hand, C3, C4, and C5 present phosphate related bands (569, 590, 962 and 1000-1100 cm-1 band), hydrogen phosphate (870 and 1218 cm-1), hydroxyl (3570 cm-1), and specific peaks showing water (1650 cm-1) and carbonate (1993 cm-1) absorption. The presence of hydroxyl ion indicates that Hydroxyapatite formation has occurred, and the water and carbonate absorption is typical after setting in Ringer’s solution due to ions exchange.
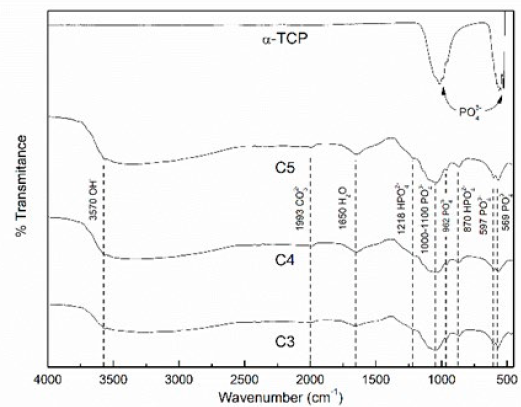
Source: The authors.
Figure 2 FTIR spectra of α-TCP and 7 days set CPCs at L/P ratios of 0.33 (C3), 0.44 (C4), and 0.55 (C5) ml/g. α-TCP peaks: PO4 3- (526, 556, 955, 989, 1019 cm-1); CPC´s peaks: PO4 3- (569, 590, 962, and 1000-1100 cm-1), HPO4 2- (870 and 1218 cm-1), OH- (3570 cm-1), H2O (1650 cm-1), and CO3 2-(1993 cm-1).
The XRD diffractogram of C4, after setting for 7 days, is presented on Fig. 3, where mainly peaks of Calcium deficient Hydroxyapatite (CDHA) is recognized; but some peaks of α-TCP and β-TCP are also observed. This shows that some alpha phase stills remain to react, due to CDHA precipitation on TCP surface which cover these particles, and then the process is then regulated by diffusion of α-TCP through CDHA. As β-TCP is a less reactive phase, after 7 days in Ringer´s solution it is still present on the cement. The morphologies of each CPC after the setting reactions are shown in Fig. 4. Mainly the obtained microstructures shown on micrographs at 5,000X are of crystal aggregates with a fine plate-like structure, which is distinctive of CDHA, or apatite-like cement, derived from α-TCP powder with particle sizes smaller than 37 µm [17,27,28]. The diminishing of the inter-aggregate crystals’ size was noted as L/P ratio rose. This phenomenon is due to the major dispersion of α-TCP particles hence creating more nucleation points for the CDHA crystals growth [29,30]. This is also reflected in the micrographs at 500X, as a less dense cement is observed as L/P ratio rises, which is in accordance with other works where CPC porosity was studied as a function of the L/P parameter [18,31].
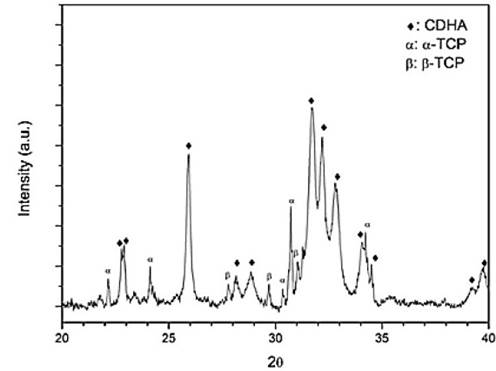
Source: The authors.
Figure 3 XRD spectra of C4 after setting for 7 days in Ringer’s solution (Reference:α-TCP JCPDS 9-348, β-TCP JCPDS 9-169, CDHA JCPDS 9-432).
Maximum compressive and flexural strength are presented on Fig. 5, where both mechanical tests show similar behavior when increasing the L/P ratio. The greater resistance is presented for C4 (11.27 ± 4.02 MPa compression; 7.49 ± 3.71 MPa Flexion) and similar lower values for C3 (8.24 ± 1.88 MPa compression; 4.85 ± 2.98 MPa Flexion) and C5 (5.21 ± 0.36 MPa compression; 3.93 ± 1.26 MPa Flexion). These mechanical results show that there is a non-linear behavior when increasing the L/P ratio in the worked range of 0.33-0.55 ml/g, which may be attributed to moldability differences of the cement pastes when mixing with water at different ratios. C3 is a quiet dry paste that presents discontinuities after molding (also observed on SEM images, Fig. 4a). On the contrary, C5 is a very liquid paste that fills the molds very easily, but it may incorporate air bubbles into the sample, generating large pores that diminish the mechanical strength. C4 has an intermediate moldability which makes it an easy-to-work paste that copies the mold shape well and generates more compact cement which can also be seen on the SEM images, Fig. 4c.
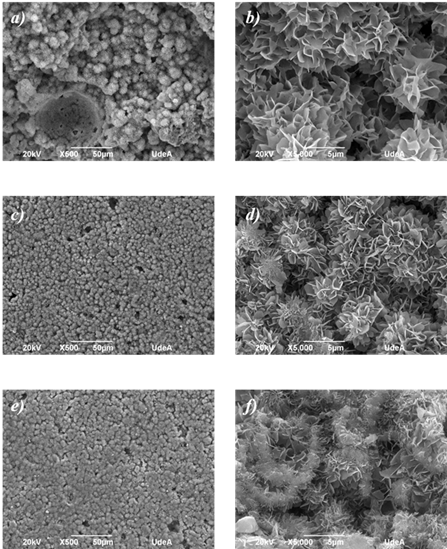
Source: The authors.
Figure 4 SEM micrographs of the fracture surfaces of the three CPC, C3 a) & b), C4 c) & d), and C5 e) & f). Scale bars of 50 µm and 5 µm for the images at 500X (left column) and 5000X (right column), respectively.
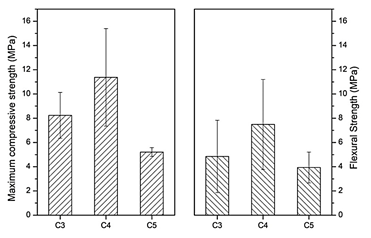
Source: The authors.
Figure 5 Maximum compressive and flexural strength of the calcium phosphate cements at L/P ratios of C3, C4, and C5 ml/g. Both tests performed at 0.5 mm/min.
The SSA applying BET theory and surface roughness is reported on Table 2 for each cement condition. It is notorious that the SSA values do not follow a linear behavior, which is inconsistent with what is presented by other researchers [17,32]. In this case, when increasing L/P ratio from 0.33 to 0.44, the SSA diminishes from 16.18 to 13.41 m2 g-1 respectively. However, when L/P ratio is increased towards 0.55, SSA increases as well to 17.18 m2 g-1. This result agrees with the mouldability behavior previously explained where the easier condition for compacting presents the lower SSA (C4) and samples with molding difficulties have higher SSA due to discontinuities and pores. The surface rugosity of samples measured on the face exposed to air is higher for C3, which once again is attributed to the low moldability, but rugosity diminishes to similar values for C4 and C5 which due to their high-water contents allow to give a flatter texture to the samples.
Source: The authors
3.3 Biofilm formation over CPC
The biofilm morphology over the three different CPC can be observed in Fig. 6 after 24 h. of incubation. Although biofilm aggregates may be identified over all samples, the two strains used did not evenly colonize the three CPC surfaces, with exception of ATCC 25923 over C4. However, both biofilm strains grew homogenously on the PS control. On the left side of the image, Fig. 6a shows a mushroom like structure of ATCC 25923 over C3, a distinct structure of biofilms [15,33]. The more stable biofilm is identified for the EPS producing S. aureus ATCC 25923 over C4 in Fig. 6c; also, in this biofilm some black areas inside the biofilm are identified, which corresponds to the fact that EPS is a substance that does not disperse the OCT signal; and thus, neither an interference signal nor image is generated.
Overall, the EPS producing S. aureus forms a higher biofilm than the non-EPS producing indicating, as reported in other studies [9,34], that EPS gives more stability to these bacteria clusters.
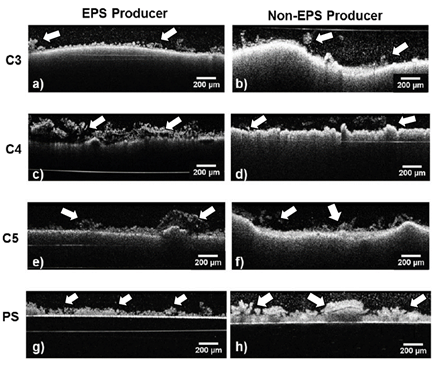
Source: The authors.
Figure 6 OCT images of the two S. aureus strains biofilm after 24h of incubation. ATCC 25923 left column a),c),e), and g) and 5298 right column b),d),f), and h) on CPCs (C3 first row; C4 second row; C5 third row) and on PS control (fourth row). Scale bar of 50 µm.
Fig. 7 illustrates the biofilm thickness quantification for both EPS and non-EPS producing bacteria (ATCC 25923 and 5298, respectively) after 24 h. of incubation as well as the SSA of the CPC. It is noteworthy that the EPS producing S. aureus ATCC 25923 presents the highest biofilm thickness over C4 (258.53 ± 97.73 µm) and the lowest over the C3 (112.92 ± 53.97 µm), being the first greater and the second less than the biofilm thickness over the PS control (179.43 ± 85.20 µm). The opposite behavior is presented by the non-EPS producing S. aureus 5298, where the minimum value was obtained on C4 (20.32 ± 9.18 µm) and highest over C5 (107.75 ±42.42 µm), all S. aureus 5298 biofilm thicknesses were under the PS control value (183.71 ± 52.51 µm; 24h). This indicates that S. aureus biofilm thickness behavior is proportional to the CPC SSA for the non-EPS producing bacteria and inversely proportional for the EPS producing one. This may be explained by the fact that the EPS will supply a major surface for the adherence of the bacteria biofilm to the CPC substratum, thus rendering a higher mechanical stability, which is one of the major characteristics of EPS [10,35].
4.1 Conclusions
The calcium phosphate cement precursor was confirmed to be α-TCP which leads to obtain an apatite-like calcium phosphate cement with fine plate-like aggregate morphology characteristic of CDHA. After 7 days of setting, C3, C4, and C5 present similar characteristics proven by FTIR; however, compression, flexion, SEM, and SSA show non-linear behavior as the L/P ratio was increased, which is attributed to the cement pastes moldability. C4 was easier to manipulate paste and then being the most compact cement with the highest mechanical resistance, indicating that the best tested L/P is 0,44 ml/g in terms of mechanical properties.
Biofilm formation of the Two S. aureus strains (the EPS and the non-EPS producing) was confirmed after 24 h of incubation onto the three CPC with L/P ratios of 0.33, 0.44, and 0.55 ml/g. Biofilm thickness showed a behavior with a breaking point above the L/P ratio of 0.44 ml/g (C4). In other words, for the EPS producing bacteria, the biofilm thickness was the highest, while for the non-EPS producing, the thickness was the lowest for this L/P ratio. The SSA of the CPC also showed a non-linear behavior. These two behaviors of the biofilm thickness and the SSA of the CPC suggest that the first is directly proportional to the second in the case of the S. aureus 5298 non-EPS producing bacteria. On the contrary, the S. aureus ATCC 25923 EPS producing bacteria is inversely proportional. This bacteria slime associated with the EPS not only helps the biofilm to be more resistant to antibiotics and external mechanical stresses but also improves the stability of the bacteria cluster, giving rise to a biofilm that does not depend on the substrate characteristics, such as surface area or porosity. This study has shown that 24 h of incubation of S. aureus strains (ATCC 25923 and 5298) is enough time for the growth of a biofilm on any of the three kinds of calcium phosphate cement here tested under static conditions and establish a base for the study of this bacterium over calcium phosphate cements by OCT.