1. Introduction
The former Soviet Union bought the Camus patent from France in 1949 to industrially produce large-format panels. Then the Giprostroy Institute in the late 1950s, created the series of houses 1-464. It was imported to Cuba in 1964, where it is popularly known as the Great Soviet Panel prefabricated system. It also appears in Chile after 1971, named by the Russian acronym KPD. The structural conception of the buildings is characterized by a crossed load transmission system, made up of panels and precast slabs, which are joined through wet joints. [1,2]
In the project documents of the Great Soviet Panel prefabricated system, the outer panels are designated by the letter E and have thicknesses of 15 cm and 25 cm thick for those located in the central zone and at the edges, respectively. The interiors are identified with the letters I, S and T, of these, the transverse ones are 12 cm and the longitudinal ones are 15 cm thick. Whereas, for precast mezzanine and roof slabs of 12 cm thick equally, the letter P is used. [2]
There are two types of buildings: with balcony and without balcony according to the terminology used initially, fundamentally with 4 or 5 levels. The buildings can have from 2 stairwells to 6, with expansion joints every two. See Fig. 1. The system thus designed, spatially, must withstand events of seismic grade 8, on the MSK scale. [2]
Of the total of 665 buildings (20433apartments) built in the study area, the authors [3], affirm that derived from the visual inspections of 200 buildings (7682 apartments), aspects leading to seismic damage are detected due to the pathological problems identified in elements structural and joints. In the sample, infiltration humidity was observed with the highest incidence in 80% of the apartments, followed by cracks and steel corrosion in 69.69% and 59.99%, respectively.
The transformations in the weight or the rigidity caused by the contraventions of the inhabitants is verified in the weight increase in 95% of the apartments in the sample, that is, due to the placement of water tanks not only in the areas of the service yard, but also in the landings of the stairs, living room and attached to the facades. In equal measure, by the placement of masonry partitions to create other premises. To a lesser extent, the rigidity is altered by the openings or elimination of panels, as well as the openings of slabs between 0.19 and 13.02%. In this order, the filling of the lattices, which is manifested in 97.63%, causes both weight and rigidity transformations. When analyzing the percentages of incidence of pathological damage and other aspects leading to potential seismic damage in the sample, it can be seen that they are very close to the ranges of the confidence intervals, for errors less than 3% in all cases.
In the previous studies by [4] where weight transformations due to the addition of water tanks and masonry walls were considered, as well as rigidity transformations only due to the opening of longitudinal panels, the impact of these aspects on seismic behavior is appreciated. Likewise, [5] obtain changes in the fundamental periods in 7 buildings with different operating conditions. It is necessary to assess a priori, the incidence of the aspects leading to potential seismic damage in the structural behavior of the entire sample diagnosed, to define the most appropriate rehabilitation actions, as well as the buildings that require immediate intervention. To meet this objective, 16 hypothetical variants were elaborated, taking into account the statistical analysis of the diagnosis made, which are compared with the 4 variants of the original typologies.
It is concluded that the potential seismic damages in the buildings constructed with the Great Soviet Panel are related to the increase of the fundamental period, both longitudinal, transversal and torsional. Pathological damage is the one with the highest incidence in this increase in the fundamental periods of vibration, followed by the increase in weight and the openings of panels or slabs.
2. Materials and methods
To evaluate the incidence of aspects conducive to seismic damage in the Great Soviet Panel system, 20 structural models are designed, of these 16 are hypothetical based on the statistical result of the diagnosis made, the remaining 4 models correspond to the original typologies. From the comparative analysis between the 4 and 5 level variants, the changes in the global control parameters such as the fundamental period (T) and basal shear are assessed.
A multi-mass model is used for dynamic analysis, which reflects the properties of materials, geometry and the links between component elements. The panels are modeled like the slabs, as finite elements type "Shell", joined continuously to each other to produce a rigid and homogeneous structural system. Likewise, stair slabs are modeled as "Shell" type finite elements connected to panels and slabs. The baseboards are considered simply supported on the continuous reinforced concrete base. Fig. 2 shows the isometrics of the geometric models of the original project.
4 hypothetical variants are considered for each typology. Table 1 shows the dimensions of the buildings in plan and elevation for each type of building (1,2,3,4A) and below the description of each of the hypothetical variants by type.
Group 1: 4 floors, without balcony
Group 2: 4 floors, with balcony
Group 3: 5 floors, no balcony
Group 4: 5 floors, with balcony
Description of the hypothetical variants
Variants 1,2,3,4B: Water tanks in courtyards, masonry wall in multipurpose areas. Filling of the lattice panels E-5 or E-4. Closure of the opening of the main door in a corner apartment, with the opening of the E-6 panel, in the typology without balcony.
Variants 1,2,3,4C: A 0.90m x 2.35m opening to a panel (I-8 or I-10) of the 2nd level, + Subgroup B. 1m x 1m opening in the P-7 slab.
Variants 1,2,3,4D: A 0.90m x 2.35m opening to a 1st level I-7 panel, and addition of a masonry wall + Subgroup B. 1m x 1m opening in the P-7 slab.
Variants 1,2,3,4E: The most critical variant among subgroups B, C, D, plus pathological damage in elements of the kitchen-bathroom and patio areas.
The strengths of the materials are obtained from destructive and non-destructive tests on concrete and steel, according to Table 2. Investigations [6-7] detail the results of the concrete tests that were carried out both on elements that are still in the warehouse area of the precast plant and on elements that are forming the buildings.
The modulus of elasticity of precast concrete is calculated by the expression recommended by [8] but with a reduction greater than 40%, as recommended by [9] for buildings made of precast panels. On the other hand, it increases by 20% because it is the seismic action of short duration, totaling a penalty of 28%. The shear modulus G is obtained from the modulus of elasticity E, assuming a Poisson's ratio = 0.17 for concrete.
The use of linear analysis requires the introduction of stiffness modifiers to reflect the degree of cracking and inelastic action that occurs in the elements immediately before creep, according to [8] and [10]; also to visualize in the structural models the incidence of the openings of the elements and the pathological damages present. The modifiers are obtained iteratively, through the calibration of the structural models, until the fundamental period of the model is greater than the natural or empirical period (T model > T natural or T empirical), since with increasing levels natural periods of movement increase. In this sense, [11] argues that the natural period of vibration of the linear system is equal to the period of the elastoplastic system only under small oscillations.
In the calibration of the subgroup E models, since they are large prefabricated panel buildings, the contributions of [11,12] are valued and values of the environmental vibration periods (TEV) from 0.14 s to 0.24 s, according to the results of [13]. In [11] when evaluating the period changes of a structure made up of cast-in-situ and prefabricated shear walls, for earthquakes of different magnitudes, in relation to forced vibrations, it obtained increases between 2-48%. In particular, [12] obtained by measuring the period of fundamental vibration of several buildings I-464 AC, subjected to explosions equivalent to an earthquake of intensity of 8 degrees or higher, obtained that the period measured after the explosion increases by these buildings by 15%.
Then, in the iterative calibration process, we start from the modifiers used in the preceding subgroups, and from the criterion that the fundamental period of the models is greater (between 2-15%) than the period of the vibrations environmental: T generated model > (1.02 ~ 1.15) TEV. Table 3 summarizes the flexural stiffness modifiers (multiplied by EI in all cases, where I is the inertia of the section and E is the modulus of deformation of the concrete) used in the calibrated structural models.
Permanent (G) and utilization (Q) loads are defined according to the [14,15] standards, respecting the considerations of the original projects. See Table 4.
Table 3 Stiffness modifiers in structural models.
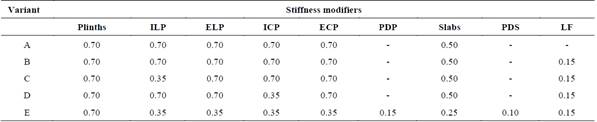
ILP: Interior Longitudinal Panels, ELP: Exterior Longitudinal Panels, ICP: Interior Cross Panels, ECP: Exterior Cross Panels, PDP: Pathological Damaged Panels, PDS: Pathological Damaged Slabs and LF: Lattice Fills.
Source: The authors
Additional considerations in the invariants of the structural modeling regarding the modeling of the loads are: water tanks with a height of 1.20 m in the service yards; 15 cm thick block walls that were added in the multipurpose areas and the filling of lattices with 10 MPa concrete. The own weight of all the elements is generated by the software from the specific weight of the material (25kN / m3).
Seismic (S) loads are modeled according to [16] standard, with the Response Spectrum Method (RSM) and the Equivalent Static Method (ESM) using the fundamental periods of the modal analysis. The three fundamental components of an earthquake, the two horizontal and the vertical, are considered, combining 100% of the seismic load in one of the main directions, simultaneously with 30% in the remaining directions. The seismic load, according to the [16] standard, in the vertical direction is modeled as an increase in the total permanent load that includes the structure's own weight, it is estimated at 20% of the permanent load due to the acceleration of response for a short period determined in the Design Spectrum for the considered soil profile.
Also, in each of the floors the accidental eccentricities of the centers of masses with respect to the centers of rigidities are considered. For the proposed model, it is verified that the centers of stiffness of each of the floors coincide approximately with their centers of mass, so that their position was assumed the same for all the floors. In the RSM method, the CQC (Complete Quadratic Combination) was used as the modal superposition formula, because it considers the proximity of the modes in the response, through the modal correlation coefficients. It is verified that the sum of the modal contribution factors is unity, as proposed by [11].
The design response spectrum is elaborated for residential buildings in the analysis region, considering the location of the studied buildings and reductions of the spectral ordinates for the energy dissipation of the Great Soviet Panel system. Next, the considerations for the elaboration of the spectrum shown in Fig.3 are detailed:
o Very high seismic danger zone (5), where the maximum horizontal ground accelerations (0.3g) for the design earthquake are due not only to the seismic zone but also to the category of the work. In the case of residential buildings, classified as "Ordinary", a "Basic Earthquake" is recommended, which for periods of useful life of 50 years and an accepted exceedance probability of 10% according to a seismic protection level D, they correspond to a return period of 475 years from the design earthquake.
o Type of soil: profile D, associated with rigid soils of any thickness that meets the shear wave velocity criteria (180 m / s ≤ Vs ≤ 360 m / s), or rigid soil profiles of any thickness that meet either of the two conditions shown:
1) 15 ≤ N ≤ 50 N: mean number of strokes of the standard penetration test, [strokes / foot]
2) 50 kPa ≤ Su ≤ 100 kPa Su: mean shear strength of the undrained test in cohesive soil strata.
o Structural system: E2 (Wall system)
o Reduction factor by ductility R = 1.5, because it is valued that they are prefabricated structures projected by repealed codes, with little ductility of the steel of the structural elements and an inadequate detailing of the sections of the elements.
The load combinations used are:
Combo 1: 1.2G+0.25Q+1.0Sx+0.3Sy +0.3Sz
Combo 2: 1.2G+0.25Q+0.3Sx +1.0Sy +0.3Sz
Combo 3: 1.2G+0.25Q+0.3Sx+0.3Sy +1.0Sz
Combo 4: 0.9G+1.0Sx +0.3Sy +0.3Sz
Combo 5: 0.9G+0.3Sx +1.0Sy +0.3Sz
Combo 6: 0.9G+0.3Sx +0.3Sy +1.0Sz
Combo 7: 1.2G+1.6Q
3. Analysis of the results
The global control parameters obtained through the application of the Response Spectrum Method and the Equivalent Static Method are exposed. Table 5 show the weight of the buildings (Ws), the fundamental periods (T), the basal shear (S) and the seismic coefficients (Cs), for each of the variants.
From the comparative analysis between the variants, the increase in the basal shear and the fundamental periods is observed; as the number of floors increases, the weight increases and structural modifications and pathological damage are incorporated. See Figs. 4 and 5.
The basal shear values are higher by the ESM and in the longitudinal direction, showing that in this direction of less lateral stiffness, the highest values of periods are presented. Likewise, when comparing the longitudinal periods of the variants of subgroups C and D, it is confirmed that the openings and elimination of panels in the longitudinal direction, transgress more than in all groups.
The fundamental translational periods are compared with those calculated by the empirical formula obtained for this system by [17]. These values are exceeded in the longitudinal direction in all variants except 3A. In the transverse direction they are not exceeded in the variants 1A-1B, 2A-2C, 3A-3C; 4A-4C. However, in no variant is the value of the approximate period Ta offered by [16] exceeded. It is verified that the fundamental torsion period is less than the translational period, with small differences between 1.90 - 5.39%, in the original variants 1A, 3A, 4A and hypothetical 1D, 3B-3E and 4B-4E. In variants 1B, 1C, 1E, 2A, 2B, 2C and 2E, the differences are shortened between 0.013-0.55%. This conditions inadequate torsional rigidity and coupling with the previous modes, mainly in 4-level buildings.
Expressions (1) and (2) allow us to calculate the values of the empirical periods.
T = 0.033 n (eq. 1) according [17]
Ta = CT (hn)x (eq. 2) according [16 ]
where:
n: number of floors
hn = total height of the building (m) from the base
CT = 0.047, x = 0.85 for E2 structural systems of reinforced concrete with rigid façade.
Longitudinal and transverse basal shear increases due to the increase in seismic weight, not due to the increase in longitudinal and transverse periods; because all the values of these periods (except the cross-sections in variants 1A, 1B, 2A, 2B and 2C) are greater than the corner period (T1) and remain on the plateau of the spectrum. For this reason, the seismic coefficients for the ESM remain constant in the longitudinal and transverse direction, except for those same variants in the transverse. The increments of the basal shear are slight, showing the highest values in the hypothetical variants 1E, 2E, 3E and 4E with increases by the ESM in the order of 2.30% - 3.60% in the longitudinal direction; 4.60-14.7% in the transverse direction. The largest increases are specifically in the 2E variant in the transverse direction. In the [2] research, it is stated that the increase in seismic demand is considerable, only if the vulnerability induced by the change in regulation is assessed.
In turn, the increase in periods is due both to the decrease in stiffness and to the increase in seismic weight. The decrease in stiffness is due to a greater extent to the presence of pathological damage in the structural elements. The increase of the seismic weight is given by the increase of the loads.
In the B variants, where the loads are increased, but the stiffness is also modified by the filling of the lattices in longitudinal panels, the period is increased, when comparing the results with the A variant (original project). For this reason, in these cases the increase in the loads has more effect than the “instantaneous” stiffness that the lattices would provide in these variants. In the remaining variants C, D and E, of all groups, the period continues to increase.
When evaluating the period increases in both directions in variants C and D, in relation to variants B, of all groups, it is concluded that these increases are due to the decrease in rigidity due to the openings of panels and slabs. At the same time, there are increases of 2.15-5.00% in variants C and 1.17-3.12% in variants D. Therefore, longitudinal openings have a greater impact than transverse ones in the change in behavior of these buildings.
The hypothetical variants with longer periods in all directions are 1E, 2E, 3E and 4E, where pathological damage is also considered. It is concluded then that the increase in the period is due to both the increase in seismic weight and the decrease in rigidity (due to panel openings, slabs and pathological damages). Increases are achieved in relation to the original project variants of 53 -71% in the longitudinal period, 82-106% in the transverse period and 90-116% in the torsion period.
In the research by [5] coincide with these results when they analyze the changes of the fundamental periods in 7 existing buildings, built with the Great Soviet Panel. From this analysis they conclude the higher incidence of pathological damage in the increments of the fundamental oscillation periods. One of those buildings analyzed, which does not present changes in weight or stiffness, nor in stiffness and weight, only presents pathological damage in the elements and structures, is where the greatest increases are obtained.
However, the pathological damages and structural transformations carried out by the residents, in the limit state of service, are not impacting to the same extent. In the research [18] it is concluded that, despite the displacements obtained in the floors, the drifts are less than the admissible ones.
4. Conclusions
This research assesses, through hypothetical variants, the structural behavior of buildings built with the prefabricated Great Soviet Panel system due to the presence in them of aspects conducive to potential seismic damage. The hypothetical variants were formed from the statistical analysis of the diagnosis carried out on a sample of 200 buildings, out of the total of 665 buildings built in Santiago the Cuba.
It is shown that in both 4-level and 5-level buildings, changes are caused in the global control parameters. The changes in the fundamental periods being more significant. Increases are achieved in relation to the original project variants of 53 -71% in the longitudinal period, 82-106% in the transverse period and 90-116% in the torsion period. With minimal differences between the fundamental torsion and translation periods, mainly in 4-level variants. Changes in seismic demand are slight. The largest increases are located in the 4-level variant (2E) in the transverse direction, but they do not exceed 14.78%.
Therefore, the potential seismic damages in the buildings constructed with the Great Soviet Panel are related to the increase of the fundamental period, both longitudinal, transverse and torsional. Pathological damage is the one with the highest incidence in this increase in the fundamental periods of vibration, followed by the increase in weight and the openings of panels or slabs. The latter cause little decrease in stiffness and at the same time little increase in the period, due to their poor representativeness in the sample. Therefore, it is urgent to undertake seismic-resistant rehabilitation actions, both in buildings with 4 and 5 levels, based on the incidence of each of the aspects leading to potential seismic damages that are present.