1 Introduction
Every year about seven million people die due to air pollution and over 90% of the world's population live in areas where the air quality guidelines are not followed. The greatest contribution to air pollution in large cities is because of emissions from the use of diesel engines [1-2] and particulate matter smaller than 2,5 microns (PM2,5) is responsible for the diseases and mortality associated with air pollution [3,4]. PM2,5 particles penetrate the lungs and might reach the circulatory system [5-7] and organs such as the brain, liver, heart, and germ cells [2,8]. Contact of particles and their components with epithelial cells might cause DNA damage, genetic mutations, inflammatory response, and progression of atheromatous plaques. These events are associated in different ways with carcinogenesis, acute and chronic respiratory diseases, cardiovascular diseases, and neurological diseases [2,3,6], [9-15]. However, the underlying biological mechanisms of toxicity of PM are not well understood [14,16,17].
PM presents a fractal-like morphology and is a complex mix of compounds, which include: metals, gases, highly persistent organic compounds such as quinones, polycyclic aromatic hydrocarbons (PAH), and other heterocyclic aromatic compounds [2,18-21]. The PM emitted by diesel engines is heterogeneous and varies in composition, physicochemical properties, and toxicity. These features depend on the technology and operating conditions of the engine, as well as the type of fuel used [12,20-22].
The need to reduce emissions from diesel engines has motivated the search for less polluting fuels that can be used with current engines [23]. Although biodiesel and bioethanol, both derived from vegetable biomass, have been employed in blends with fossil diesel [23-27], information on the impact of their PM emissions is insufficient and contradictory. Hence, there is a growing concern about its potential effects on health [11,22,28-30].
In vitro biomarkers are important tools for detecting the toxicity of different agents such as PM and to evidence a wide range of molecular and cellular responses associated with PM adverse health effects. Moreover, they offer relevant information about the toxicological diversity of these materials and help to define prevention and mitigation strategies [14,16-17,31-33].
The assays to evaluate reverse mutation using different bacterial strains with specific mutations allow to characterize different compounds responsible for a mutagenic effect [1,34-42]. In the alkaline version, the comet assay detects a broad spectrum of DNA damage, such as fragments of single or double-strand breaks, lesions to alkali-labile sites, and incomplete excision repair sites [39,40,43-48]. The analysis of differentially expressed genes to evaluate the response to PM toxicity in human cell lines through Next Generation Sequencing (NGS), makes possible to identify new biomarkers and also, to relate the genotoxic effect with metabolic pathways and cellular processes affected by the PM [16,49].
2 Materials and methods
2.1 Engine characteristics and obtention of particulate matter
An Isuzu 4JA1 pre-Euro I, 2,5 L, direct injection, four-cylinder diesel engine was used, whose characteristics, operating conditions, nano-structural and morphological properties of the PM are discussed in [26,50]. The engine was started on clean low-sulfur fossil diesel, it was kept on stationary conditions until reaching 2410 rpm and the PM was collected at 43 and 95 Nm of torque. When these conditions were reached, 10% of the energy fraction of diesel was substituted with ethanol injected through a multipoint injection system (dual-injected ethanol/diesel fuel). In order to eliminate any residue after running the engine with the dual-injected ethanol/diesel fuel, the combustion system was drained for 15 min before being run again with clean low-sulfur fossil diesel.
Four undiluted PM samples were filtered and collected with stainless steel fibers positioned at 1,5 m after the exhaust manifold pipe. Gas temperature was kept below 200°C to avoid oxidative reactions in the PM, and the PM recovered by scraping the stainless-steel fibers was stored in sterile amber glass containers.
2.2 Organic extraction
The collected PM was sonicated with dichloromethane (DCM) as a solvent [28,51-62].
Fifty milligrams of PM from each fuel was mixed with DCM in a 1:2 ratio, the mix was sonicated at 40Hz for one hour at room temperature [63]. Subsequently, it was centrifuged for 10 minutes at 2.000 rpm, the extracted suspension was collected, and the process was repeated three more times using the precipitate. The supernatant was filtered through Nylon membranes (0,22 µm), followed by evaporation under a gentle stream of nitrogen gas and resuspended in dimethyl sulfoxide (DMSO). Because we were not able to weigh the organic extract of each fuel, doses were expressed in amounts (µg) starting material of PM of each fuel, so, the maximum concentration of each extract was of 0,16 µg PM Equivalents/µL. The expression of concentration in this way has been used by Claxton when referring to NIST Standard Reference Material (Diesel SMR 1650) [64] and by Umbuzeiro et al for fractions of air particulate material [65].
Clean stainless-steel fibers with the same characteristics as those used to collect the PM were subjected to the previous organic extraction process to determine their influence on the toxicity of the PM extracts.
2.3 Mutagenicity assay on Salmonella/microsome
The mutagenic activity of the organic extracts was evaluated with the Salmonella/microsome test [36], the Kado micro-suspension method [38,66]. TA98 and TA100 Salmonella strains auxotrophs for histidine (his-) and sensitive to detect most environmental mutagens were used [42]. The TA98 strain with a frameshift (hisD3052, Δ(uvrB, bio), rfa, Apr (ampicillin resistance) (pKM101) and the TA100 strain with base substitution (hisG46, A(uvrB, bio), rfa, Apr (ampicillin resistance), (pKM101), both strain in the absence of (-S9) and the presence of (+S9) metabolic fraction from a male rat's liver (induction with Aroclor 1254, Moltox S.A., USA). For each strain, nine concentrations from each extract were used per assay: 0,002; 0,005; 0,01; 0,02; 0,04; 0,07; 0,15; 0,3 and 0,6 µg equiv, of PM/µL on strain TA100 and 0,0002; 0,0005; 0,001; 0,002; 0,005; 0,01; 0,02, 0,04, 0,07, 0,15 µg equiv, of PM/µL on strain TA98. Negative controls were dMsO and the extract of clean stainless-steel fibers, and positive controls were Daunomycin (6 µg/plate) for strain TA98 -S9, Sodium Azide (1,5 µg/plate) for strain TA100 -S9 and 2-Aminoanthracene (5 µg/plate) for both strains, TA98 +S9 and TA100 +S9. The results were expressed as average revertants/µl and potential mutagenic activity was considered when the treatments showed statistically significant differences with the negative control and when the concentration-response relationship was significative (p<0,05) in any of the used strains.
2.4 Cell culture
The liver epithelial cell line HepG2 ATCC® (CRL -10741 LOT: 61777384), competent for the expression of a great variety of cytochromes P450 and widely used for genotoxicity assays of complex mix [67] was cultivated in Dulbecco's Modified Eagle Medium (DMEM), supplemented with fetal bovine serum (FBS) at 10%, 50 IU/ml of penicillin, 50 ug/ml of streptomycin. Cells were incubated at 37°C in a humid atmosphere and 5% of CO2. Cells were used when reached a cell confluence of 80% and changed every 20 passages.
2.5 Cell viability through mitochondrial functionality (MTT reduction) in the HepG2 cell line
Cell viability in the HepG2 line was evaluated through the MTT assay by reduction of the compound 3-(4,5-dimethylthiazol-2-yl)-2,5-diphenyltetrazole bromide. The methodology suggested by [68] was followed, 5.000 cells/well were seeded in 96-well dishes and were exposed in darkness to 20 µl of each extract of organic PM, then, were incubated at 37°C in humid atmosphere and 5% of CO2 during 24 hours. Twenty 1:1 serial dilution was evaluated, ranging from 0,16 to 0,0005 µg equiv of PM/µl.
Later, 10 µl of MTT were added to the cells, placed in an orbital shaker at 37°C for 5 h and then, 100 µl of cold isopropanol were added to each well to dilute the formazan crystals. The culture plates were placed in an orbital shaker platform for 12-24 hours and absorbance was measure using a Multiskan-GO spectrophotometer (Thermo Scientific) at 570 nm.
Viability (%) was calculated according to the equation:
Where: OD TnT is the optical density of the treated cells and OD neg.con is the optical density of untreated cells or negative control (clean stainless-steel fibers extract).
2.6 Genotoxicity in the HepG2 cell line with the Alkaline Comet essay
Genotoxic activity of the organic PM extracts from fuels was determined by alkaline version of the comet assay modified by McNamee [69]. Thirty-five thousand HepG2 cells were seeded in 12-well dishes, cells were treated with 10 µl concentrations of 0,0007, 0,0013; 0,026; 0,005; 0,01; 0,02; 0,042, 0,08 and 0,16 µg equivalent of PM/µl for 24 hours at 37°C in a humid atmosphere and 5% of CO2. Afterwards, cells were embebed in low melting point agarose and placed on gelbond films, then lysed and subjected to alkaline electrophoresis (pH > 13). Finally, the cells were stained with GelRedTM (Invitrogen Corp; CA, USA), observed and measured using a fluorescent microscope Nikon® Eclipse 55i (Tokyo, Japan) with a 20X objective. The length of the comet was randomly measured in 100 cells with an eyepiece micrometer (um) [70-72], the fraction of damaged cells (%DC) and the cut-off point were from the equation:
Where: D is the damaged DNA, avg. len. nc is the average length of DNA migration from the negative control or untreated cells (clean stainless-steel fibers extract) and SD is the standard deviation of the mean.
Three independent assays were performed, which included untreated cells, cells subjected to the clean stainless-steel fiber extract as negative controls, and 100 µM of H2O2 as positive control. A genotoxic effect was considered when the treatments exhibited statistically significant differences with the negative control and the concentration-response relationship was significant (p<0,05).
2.7 RNA extraction andRNA-seq analysis in theHepG2 cell line
For gene expression analysis, HepG2 cells were grown as described above and exposed for 24 hours to 0,16 µg equivalents of PM/µl of the organic extract obtained from each fuel, fossil diesel and dual-injected ethanol/diesel. The experiment involved three technical replicates per organic extract, including negative controls (HepG2 cells exposed to organic extract from clean stainless-steel fibers). Total RNA extraction was performed to 1x 106 cells from each group with Trizol (Invitrogen) following the manufacturer's recommendations. Quantity and quality of the RNA was determined with an UV light spectrophotometer on a NanoDropTM2000 (ThermoFisher Scientific) at an absorbance of 260 nm. The RIN (RNA Integrity Number) was determined by utilizing the Agilent RNA 6000 Pico kit (Agilent) and a Bioanalyzer (Agilent). The sequencing libraries were prepared with the TruSeq RNA library Prep kit (Ilumina INC, CA, USA) using poli-T beads for mRNA enrichment. Finally, paired-end libraries (2 x 150 nt) were sequenced in a HiSeq 4000 plataform (Ilumina Inc., CA, USA).
The raw reads underwent a cleaning process to completely remove reads or low-quality ends (Phred score <30), for which the TRIMMOMATIC software version 0.39 was implemented [73]. Subsequently, the clean reads were mapped to the reference human genome (GRCh38) with the STAR software version 2.6.0 [74] with all the default parameters. The reads count associated with each gene were generated with the HTSEQ-COUNT software version 0.11.1 utilizing all the default parameters [75].
The differential gene expression analysis was performed with the EDGER package version 3 [76]. Differentially expressed genes (DEGs) were identified by establishing a cut-off point of log2 FC (Fold Change) of 1,5 and a P value <0,05. The REACTOME database version 73 was implemented to group DEGs according biological processes [77] and enriched pathways reported by the database were selected considering a P value <0,05.
3 Results
The organic extract from the clean stainless-steel fibers did not show mutagenic or genotoxic activity, therefore biological activity of the organic extracts of the fuels was not affected.
3.1 Mutagenicity with the Salmonella/microsome assay
The organic extracts of PM obtained through both operation modes, 43 and 95 Nm of torque, from fossil diesel and from dual-injected ethanol/diesel were mutagenic (Fig. 1), especially in TA98 strain in absence of metabolic enzymes. Extract from dual-injected ethanol/diesel was more mutagenic than fossil diesel extract. Organic extracts of PM obtained at 43Nm were significantly more mutagenic than those obtained at 95Nm of torque (p<0,05).
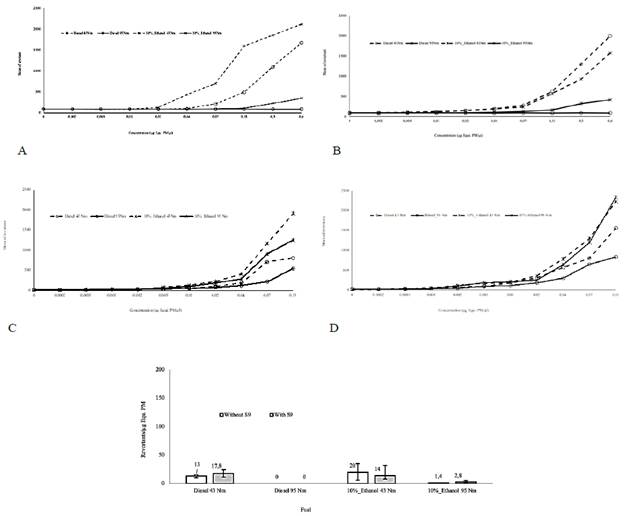
Results represent the average of three independent experiments. Concentration - response significance obtained with the ANOVA was p<0,001.
Source: authors.
Figure 1 Evaluation of activity mutagenic in the strains TA 98 and TA 100 with and without S9. Concentration - mutagenic response curves of the PM organic extracts from fossil diesel and dual-injected ethanol/diesel fuel in the TA100 and TA98 strains in the absence (-S9) and presence (+S9) of metabolic enzymes. Figure 1A: mutagenic activity TA 100 -S9, Figure 1B: mutagenic activity TA 100 + S9, Figure 1C: mutagenic activity TA 98 -S9 and Figure 1D: mutagenic activity TA 98 + S9. The average of revertants (X ± SD) in negative controls were: TA100 strain + DMSO (- S9) (88 ± 2) and (+S9) (94 ± 3); TA98 strain + DMSO (-S9) (24 ± 3) and (+S9) (28 ± 2); TA100 strain + extract from clean stainless steel fiber (-S9) (93 ± 5) and (+S9) (97 ± 3); TA98 strain + extract from clean stainless steel fiber (-S9) (25 ± 4) and (+S9) (27 ± 3). The average of revertants (X ± SD) in positive controls were: TA100 strain + Sodium Azide 1,5 µg/plate (-S9) (2300 ± 90); TA98 strain + Daunomycin 6 µg/plate (-S9) (1354 ± 45); TA100 strain + 2-Aminoanthracene 6 µg/plate (+S9) (1684 ± 76) and TA98 strain + 2-Aminoanthracene 6 µg/plate (+S9) (670 ± 18).
3.2 Cell viability through mitochondrial functionality (MTT reduction) in the HepG2 cell line
After exposing HepG2 cells to 20 concentrations of organic extracts from PM for 24 hours, there was no evidence of loss of mitochondrial functionality. Therefore, the extracts were considered non-toxic.
3.3 Evaluation of genotoxicity with the Alkaline Comet assay in HepG2 cells
Independent of the fuel or the operation mode of the engine, all the extracts were genotoxic. The concentration at which most of the organic extracts caused damage to 50% of the cells oscillated from 0,02 to 0,04 µg equivalent of PM/µl. One-hundred percent of the cells exhibited DNA damage from 0,08 µg equivalent of PM/µl with all the extracts. Regarding the operational mode, a higher genotoxic effect was evident at 43Nm of torque (Fig. 2).
3.4 Gene expression in the HepG2 cell line: RNA-seq Transcriptomics
Considering the results of the comet assay, the 0.16 µg equivalent of PM/µl concentration of the organic extracts obtained at an engine load of 43Nm was selected to perform the gene expression analysis. After normalizing the data, the average count of reads was 17 with approximately 10.000 genes detected with at least 5 reads for each of the conditions analyzed. Fifty percent of the detected genes had a count of 39 reads. Differentially expressed genes were identified when the expression profiles of HepG2 cells exposed to organic extracts from both fuels, fossil diesel and dual-injected ethanol/diesel, were compared to the expression profile of HepG2 cells exposed to extracts from clean stainless-steel fibers.
Fig.3 shows the distribution of DEGs of each organic extract from the fuels compared to HepG2 cells exposed to clean stainless-steel fibers extract (negative control). Both treatments displayed a higher proportion of upregulated genes in contrast to downregulate genes. Organic extract of PM from fossil diesel affected fewer genes than the organic extract of PM from dual-injected ethanol/diesel. Non-coding RNA were detected, although the methodology involved a mRNA enrichment based on polyT beads, those transcripts correspond to pseudogenes, micro-RNAs, long non-coding RNAs (LncRNAs), and antisense genes.
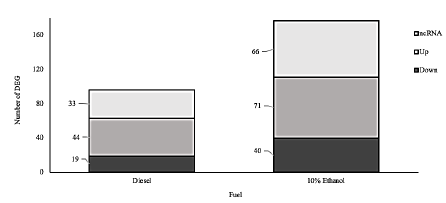
Source: authors.
Figure 3 Differential expressed genes (DEG). Number of differentially expressed genes (p<0.05) with respect to untreated cells in the HepG2 cell line. Cells were exposed for 24 h to 16ug equivalent of PM/µl of PM organic extract of fossil diesel and dual-injected ethanol/diesel. ncRNA: non-coding RNA, Up: upregulated genes, Down: downregulated genes.
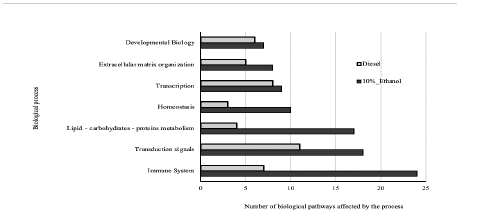
Source: authors.
Figure 4 Main biological processes affected by organic extract PM. Main biological processes Number of biological pathways affected by the DEG in HepG2 cells. After 24 hrs exposure to 0,16 µg equivalent of PM/µl of the PM organic extracts of fossil diesel and dual-injected ethanol/diesel. Reactome databse was implemented to group DEGs in each biological process.
An enrichment analysis of pathways in the Reactome database identified the most affected biological processes. In their respective order, the immune system, transduction signals and lipid-carbohydrate-protein metabolism (Fig. 4). The enrichment results evidence that the organic extracts modulated the expression of various pleiotropic genes, such as the CASTPERG (Cation channel sperm associated auxiliary subunit gamma) and CXCL8 gene (C-X-C motif chemokine ligand 8) for the diesel organic extract, and the FOS genes (Fos proto-oncogene, AP1 transcription factor) or CSF1R (Colony stimulating factor 1 receptor) for the dual-injected ethanol/diesel extract. It was also observed that several genes might affect a single pathway, for example, for the immune system, the interleukin signals, cytokines, and the JAK-STAT pathway through cytokines (Table 1).
Table 1 Processes and biological pathways affected by DEGs. Annotation corresponds to Gene Ontology (GO) - Reactome terms._
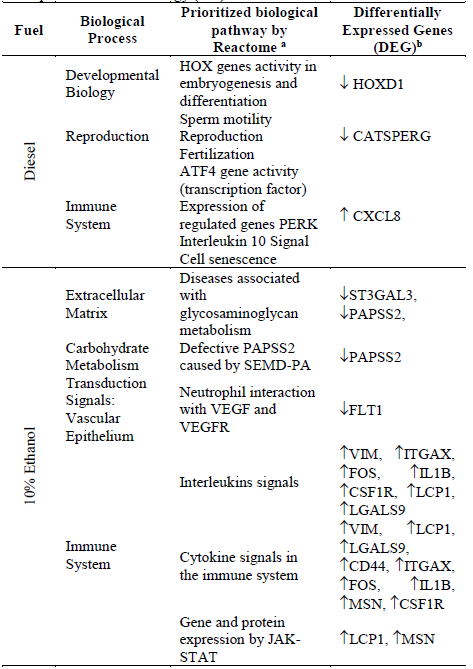
ap<0,05
bDEG up (↓) and DEG down (↓).
HOX: Homeobox Gene, CATSPERG: Cation Channel Sperm Gene, CXCL8: Interleukin 8 Gene, LCP1: Lymphocyte Cytosolic Protein Gene, MSN: Moesin Gene, VIM: Vimetin Gene, CD44: Molecule Differentiation Gene, CSF1R: Colony Stimutating Factor Gene, IL1B: Interleukin 1 Gene, ITAGX: Integrin Alfa X Subunit Gene, ST3GAL3: Beta Galactoside Sialyltransferase Gene (membrane protein), PAPPSS2: Phosphoadenosine Phosphosulfate Transferase Gene, FLT1: Tyrosine Kinase Protein Gene member of Vascular Endothelial Growth Factor Receptor (VEGFR), FOS: Proto-oncogene FOS, LGALS9: Galectin Gene (extracellular matrix).
Source: authors.
Among other enriched pathways, those related to the embryogenesis and fertility were found in cells treated with the extract from diesel, while the dual-injected ethanol/diesel extract affected carbohydrate metabolism pathways. It is important to highlight that some modulated genes from both PM organic extracts take part and link the complex network of the DNA damage repair (DDR) system with the immune system pathways through different mechanisms (Table 2).
Table 2 DEGs associated to the DDR system and biological processes of the immune system
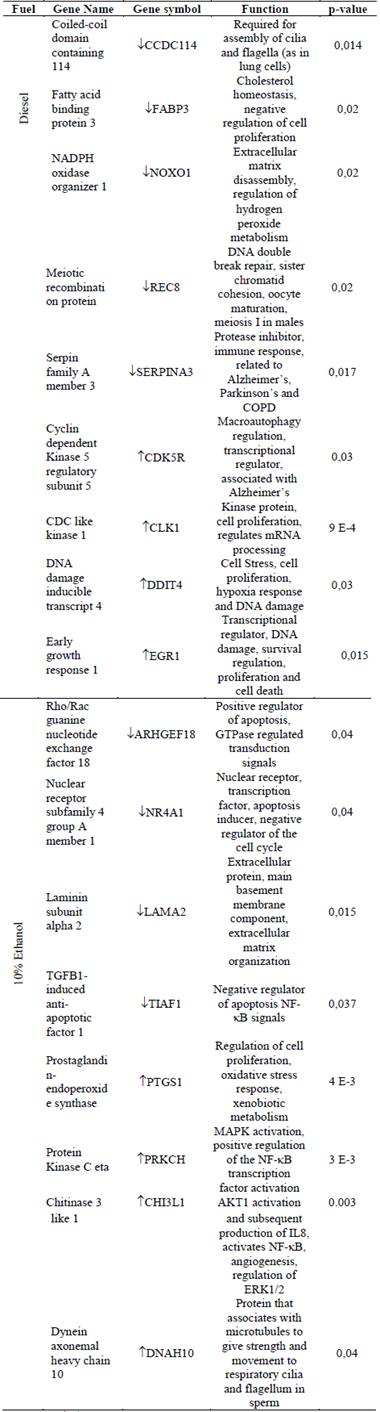
The arrows ↑, ↓ indicate gene regulation, up-regulated genes (Up) or down-regulated genes (Down), respectively more oxygenated aliphatic groups (alkenes, methylenes, and methyls) in the surface of the PM than fossil diesel, mainly when the engine operated at 43Nm of torque. Hydrocarbons, especially PAH, could be responsible for the mutagenicity with +S9 [81], while the aliphatic groups could cause mutagenicity without metabolic activation (-S9) [34]. Additionally, the increase of NOx in the combustion of diesel spread with alcohols could favor the formation of modified PAH as nitro-PAH, whose reactivity is greater in the TA98 strain without S9 [1,82]. Salmonella strains with the sensibility to specific chemical groups should be tested to identify the chemical groups in the extracts responsible for the mutagenicity, such as YG1041 (sensitive to nitroaromatics without S9 and amino aromatics with S9), YG5185 (sensitive to unmodified PAH), TA104 (sensitive to free radicals) or YG7108 (highly sensitive to alkylating agents), among others. Furthermore, the PM organic extract could be chemically characterized according to the differential mutagenic response of the strains.
Source: authors.
4 Discussion
Evaluation of the toxic potential of the PM derived from the combustion of fossil fuel fumigated with bio-alcohols has reached increasing interest because of the risk to public health [76]. The results of this investigation showed toxicity of the organic fraction from PM emitted by a pre-EURO I diesel engine fueled with diesel and ethanol through a modified multipoint injection system, to replace 10% of the energy fraction of diesel with ethanol. However, the health effects of PM from new fuels are still unknown, so it is a priority to explore the toxicological characteristics of these particles in large urban centers.
In this study, we could not weigh the organic extract of PM, thus, we don't determine the percentage of extractable organic material because the particles are not fully extractable organics. In addition, we did not perform any chemical characterization of the PM extracts. But it is well known that PM from fuels is a cocktail of compounds, implement a battery of in vitro bioassays covering major toxicological endpoints as genotoxicity, mutagenicity, and activation of cellular mechanisms, are considered of pivotal importance to identify potential risks to ecosystems and human health [77]. The results provide information about mutagenic and genotoxic compounds in PM from the dual-injected ethanol/diesel fuel.
All organic extracts of PM were mutagenic for at least one strain used in the Salmonella/microsome test. The strain TA98 with or without metabolic activity was the most sensitive to detect mutagenicity. These results indicate that organic extracts contain diverse mutagens, some (stable, persistent, and bioaccumulative) require metabolic activity, the mutagenicity without metabolic activity (-S9) evidenced other mutagens more reactive and less persistent. Organic extracts from dual-injected ethanol/diesel showed statistically significant differences to induce mutagenicity compared to the fossil diesel extract. Nevertheless, the mutagenic emission factor (rev/MJ or rev/kg fuel burned) is the most critical value to determine whether the emissions are mutagenic [78], this study did not measure that parameter.
Concerning the influence of the engine load, the organic extract obtained at 43 Nm of torque was more mutagenic, which results in agreement with other investigations [12,20-22,79]. This can be explained because the engines that remain on idle or low load for long periods, operate with an excess of air and therefore, the combustion efficiency is reduced, and the emission of particles is favored [15,26]. According to Kisin et al., [25], when comparing the mutagenicity of PM in the TA98 strain, the biological reactivity of PM increase when heavy or light engines operate at lower torque, which agrees with the results of this research.
Although the extracts were not chemically characterized, the mutagenic activity (with or without metabolic activity) and the differential sensitivity of both Salmonella strains suggest the presence of chemical groups responsible for such activity. According to previous reports with the same fuels used in this study [26,50], the combustion of dual-injected ethanol/diesel produces between two and four times more total hydrocarbons, large amounts of nitrogen oxide (NOx), and organic extracts of fosil diesel.
Treatments with the PM organic extracts induced DNA ruptures evidenced by the comet assay and the genotoxic potency values of the extract from the dual-injected ethanol/diesel showed an increase of the genotoxic effect in the hepatic cell line HepG2. In an earlier research, dual-injected ethanol/diesel was genotoxic and induced a greater number of cells with damage on human lymphocytes compared to fossil diesel. These effects were influenced by the operating conditions of the engine, the extracts from a low load (43Nm of torque) displayed higher genotoxicity [22]. The differences detected in the genotoxic profiles of the PM the evaluated fuels could be because replacing 10% of the energy fraction of diesel with ethanol through port injection produce more organic carbon emissions, total hydrocarbons [26], and high content of oxygenated and aliphatic groups compared to fossil diesel [50]. Hydrocarbons, especially PAH and aliphatic groups could be responsible for the induced genotoxicity in HepG2 cells from the PM organic extracts of the dual-injected ethanol/diesel [1,34,81,82].
The differential gene expression through RNA-seq displayed significantly different effects (p<0,05) between the two extracts, the organic extract from dual-injected ethanol/diesel regulated a greater number of genes. Both organic extracts modulated non-coding RNAs (ncRNA), these molecules are frequently involved in physiological and pathological processes such as inflammation, cell cycle regulation, and cancer, for example, patients with pulmonary disease presented an altered expression of ncRNA [83]. The role of these markers in the PM toxicity should be explored using specific methodologies to enrich ncRNA, evaluate different exposure times or cell lines.
Different pathways and genes involved in the cellular process of the immune system were more affected by both extracts. The organic extract from dual-injected ethanol/diesel significantly increased the production of transcripts of cytokines and interleukins, as IL1B, IL6, and TNF-a, while the organic extract from fossil diesel mainly altered the IL10 pathway. The expression of cytokines and interleukins could be activated by transcription factors, among others, due to oxidative stress resulting from exposure to PM [21], which might be responsible for the genotoxic effect and be associated with lung aging and respiratory damage [84]. Cytokines and interleukins are involved in cellular processes as inflammatory response, extracellular matrix, and cell junctions remodeling, which are associated with chronic respiratory diseases [2]. It has been established that an increase in cytokines and interleukins affects the adherent junctions between cells, which induces transduction signals that stimulate the release of the vascular endothelial growth factor (VEGF). In this study, the gene ontology analysis allowed to identify a disturbance of the VEGF signaling pathway when cells were exposed to extract from dual-injected ethanol/diesel fuel. Such disturbance alters the vascular permeability and induces inflammation with deleterious consequences on arteries and veins, which is related to cardiovascular accidents such as acute myocardial infarction and atherosclerosis [85].
In this research, there was an increase in transcripts such as IER3, CSF1, CHI3L1, PRKCH among others, whose protein products take part in the activation of mitogen-activated protein kinases (MAPK) to modulate the activity of pro-inflammatory transcriptional factors, such as NF-kB, which induce the production and release of cytokines and chemokines [86]. Transcripts from genes such as STABILIN 1, MATR3, FTL1, PCAM1, were differentially regulated by the PM organic extracts and could induce an inflammatory response through an alteration in membrane receptors or membrane transporters, affecting second messengers such as intracellular calcium, and disturbing the interaction with intracellular targets, cholesterol synthesis, lipid, and carbohydrate metabolism. These conditions trigger multiple transduction signals and promote the production of chemokines and cytokines [86,87].
It has been reported that exposure to organic fractions derived from PM induces immune response because of DNA damage. Genotoxicity activates the signal network related to DNA damage response (DDR) which triggers immune system signals [86,88-91]. Genomic instability induced by the damage of PM organic extracts on the DNA could increase cell proliferation, inhibit apoptosis, and remodel intercellular junctions, processes with pro-inflammatory properties. The mutagenic activity and DNA damage evidenced in this research, are sensed by proteins of the DDR system which form complexes with kinase proteins at the site of damage. In this way, they phosphorylate some proteins that participate in the regulation of the cell cycle, DNA repair systems, and transcription factors that regulate the immune system such as IL receptor, JAK's kinases, transduction, and transcription activation signals [88,89,92]. As an example, single and double-strand DNA breaks, evidenced by the comet assay, induce interferon genes through ERK-1/2 signals and the MAPK cascade. On the other hand, the AP sites generated by the DNA base excision repair (BER) system are a substrate for the AP endonuclease enzyme. This enzyme also regulates transcription factors for NF-kB, a protein necessary for the expression of TNF, IL8, and IL6 [88,92]. PM organic extracts evaluated in this research regulated apoptosis-related genes (ARHGEF18, TIAF1-TGFB1), proliferation, cell cycle, and MAPK cascade regulators (ERK1/2, AKT1, and NF-kB), genes involved in DNA repair, and extracellular matrix remodeling (CLK1, ERG1, DDIT4, REC8, LAMA2, NR4A1, CHI3L1), among others.
3. Conclusions
Considering these research findings and the successive use of different polarity solvents that broaden the spectrum of the extracted compounds from PM, is important to study the toxicity potential of PM fractions to dilucidated which compound are responsible for the toxicity of this material. Furthermore, it is essential to consider the toxicity of different size materials and to validate the participation of the proposed genes in each step of the model through q-PCR.
Cells grown in 2D cell cultures and exposed to PM extracts allow establishing the interactions between microparticles, their components, and the modulated cellular processes or metabolic pathways. Despite these advantages, 2D cell culture conditions poorly imitate the cell-cell functional interactions nor replicate the specific mechanic and biochemical microstructure of an organ. Consequently, this kind of research should be continued by implementing experimental techniques such as co-cultures and 3D cell cultures, which mimic the structural and functional complexities of human cells as accurately as possible.
Considering the challenge of experimental procedures without the use of animal models, implementing bioanalytical tests based on biomarkers, in conjunction with epidemiological and environmental studies, constitutes an effective strategy to protect public health. These tests offer rapid and reliable results to predict the effects of different atmospheric pollutants on human beings.