1. Introduction
Polyhydroxyalkanoates (PHAs) have become important due to their mechanical properties, which resemble those of plastics derived from petrochemicals, being rigid and brittle plastics even similar to rubber. They are naturally produced by microorganisms as intracellular carbon reserves and are biodegradable, eliminating the problem of persistence associated with conventional plastics [1,2].
Despite the environmental advantages of PHAs over petrochemical-based plastics, its use is limited by the high production costs estimated between € 2-5 / kg, exceeding the prices of petroleum-derived plastics for which it is estimated at approximately € 0.93 / kg, directing its production towards specialized applications and preventing them from being competitive from an economic point of view [3-6].
This has stimulated the study of better processes that allow increasing the productivity and reducing the production costs of these biopolymers. Some of the alternatives implemented have been: bioprospecting towards the search of new producing strains, use of unconventional substrates rich in carbon and trace elements, improvement of the extraction process and variation in the strategies of the cultivation process (fed batch and continuous) [1,7-10].
In terms of the culture systems used as a measure to improve the PHAs production process, the systems fed batch are the most studied, since they allow to obtain greater productivity [11]. This mode of operation has advantages compared to traditional fed batch, since it makes it possible to obtain high cell density cultures, which advantage the PHAs production process because this is an intracellular metabolite. This is because the method allows microbial growth to be maintained at a specific rate by means of the controlled addition of nutrients to the medium, avoiding negative effects of substrate inhibition [12].
The fed batch also includes different feeding methods, each with specific characteristics that depend on the type of control. One of the methods used is the feeding method without feedback control. This method is based on the estimation of feeding profiles in an empirical way and includes different strategies, such as: the addition of constant velocity flows (fixed), flows with increased feed rate (linear) and exponential feeding, the latter being the most used. Although these methods allow a simple feeding profile to be established, they do not allow process control because they do not control the possible changes in the physiological response of the microorganism to the environment and to the culture conditions. As a result, the residual concentration of the substrate cannot be controlled, which can negatively affect the growth of the microorganism and the accumulation of the polymer [8]. For this reason, the method defined for nutrient feeding is a critical factor in obtaining cultures with high cell density because it affects not only the growth but also the volumetric productivity, which will be reflected in the amount of polymer produced.
The objective of this study was to improve the production of PHAs from a new feeding model without feedback control, using a wild strain of bacteria and an unconventional substrate for the culture medium.
2. Materials and methods
2.1. Microorganism
A PHA-producing bacterial isolate, identified as Burkholderia sp, belonging to the Biotransformation Research Group of the Universidad de Antioquia, was used. It was maintained at -20°C in tryptose soybean broth (TSB) with 30% glycerol for preservation.
2.2. Culture medium
A cassava hydrolysate of an inedible variety was used as the carbon source, supplemented with salts from the MMS reference medium [13]. The hydrolysate was obtained by adding 4 mL of STARGENTM 001 enzyme per L of culture medium under conditions of 80 °C, 300 rpm and 4 h [14]. The composition of the MMS medium is: 6.7 g/L Na2HPO4.7H2O; 1.5 g/L KH2PO4; 5.46 g/L (NH4)2SO4; 0.2 g/L MgSO4.7H2O; 0.06 g/L iron ammonium citrate; 0.01 g/L CaCl2.2H2O; 1 mL trace elements.
2.3. Carbon/nitrogen ratio (C/N) and carbon / phosphorus (C/P)
To evaluate the effect of the C/N ratio, an elemental balance of the culture was performed using the C/N ratio of the MMS medium as a reference. The stoichiometric balance was established with glucose as the carbon source and ammonia as the nitrogen source. The substrate yield in biomass reported by Alcaraz et al. (2019) [15] for the production of PHAs with cassava flour hydrolysate (YX/S: ≈ 3 mol/mol) was taken as a reference and the elementary formula of a microorganism was used as a producer (Cupriavidus necator: CH1,77O0,5N0,248).
C6H12O6 + aO2 + bNH3 = YX/SCH1,77O0,5N0,248 + dH2O + eCO2
Once the balance was established, 5 different C/N ratios were defined and the amount of ammonium sulfate ((NH4)2SO4) required for each ratio was calculated (Table 1).
The evaluation of the C/P ratio was made on the basis of the different phosphorus salts that make up the reference medium, using the concentrations of this medium as the central point. Subsequently, 5 C/P ratios were defined (Table 2).
The evaluation was performed in Erlenmeyer-type cultures with 100 ml of culture medium, using 10 g/l sugar as the basis for the experiment. Cultures were performed under standard conditions of temperature (33°C), initial pH (7.0) and agitation (150 rpm). The other components of the medium were held constant. Cultures were performed in 500 ml Erlenmeyer flasks with gauze plugs to facilitate gas transfer. All experiments were performed in triplicate.
Table 1 Treatments to evaluate the C/N ratio according to the stoichiometric balance.
Ratio C/N (g/g) | N* (g/L) |
---|---|
3.46 | 5.46 |
4.54 | 4.16 |
6.91** | 2.73** |
12.28 | 1.54 |
75.50 | 0.25 |
N*: concentration of (NH4)2SO4 required for the culture medium according to the C/N ratio.
**Carbon/nitrogen ratio (C/N) defined by the reference medium.
Source: The authors
Table 2 Treatments to evaluate the C/P ratio according to the stoichiometric balance. Source: The authors
Ratio C/P (g/g) | P1 (g/L) | P2 (g/L) |
---|---|---|
3 | 8.01 | 1.92 |
5 | 4.81 | 1.08 |
7.17* | 3.35* | 0.75* |
10 | 2.40 | 0.58 |
14 | 1.72 | 0.38 |
(P1) Concentration of Na2HPO4.7H2O and (P2) KH2PO4 required for the culture medium according to the Carbon/phosphorus ratio. * (C/P) ratio defined by the reference medium.
Source: The authors
2.4. Dissolved oxygen concentration
It was evaluated from an experimental design on a central response surface composed of 5 central points, using the STATGRAPHICS Centurion XVI.I. The design related two factors, dissolved oxygen concentration (%O2) and air flow (L/min). The range of factors was defined based on what was reported in the literature: aeration 0.5 - 2.0 vvm; O2 saturation: 10 - 40% [11,16].
The evaluation was performed in a 1.5 L Applikon ADI 1030 Bio Controller bioreactor with a Bio Xpert Lite data acquisition program and equipped with a Mettler Toledo M300 to control the supply of pure oxygen.
Cultures were evaluated at a sugar concentration of 20 g/L and an inoculum volume of 8%. The air flow was defined by a rotameter and the oxygen concentration of the medium was controlled by a cascade control system with agitation variation, by in-line measurement of O2 with a polarographic submersible membrane electrode.
2.5. Evaluation of the culture using exponential feeding
Among the different types of feeding without feedback control, exponential feeding is the most commonly used, as the model is designed based on the specific growth rate (µ) of the microorganism [11,17] (eq. 1).
Where:
F(t): substrate feed flow (ml/min)
µ: specific growth rate (h-1)
YX/S: biomass yield per unit of substrate (g/g)
Vo: volume of culture at start of feeding. (L)
Xo: biomass at the start of feeding (g/L)
t: cultivation time (h)
S: feed substrate concentration (g/L)
The model was derived to maintain the concentration of residual substrate at a desired level. The model predicts the substrate flow required to produce a defined amount of biomass (ΔX2) at a later time interval, assuming the growth trend produced by the microorganism in the immediately preceding time interval (ΔX1) is constant (Fig. 1). The rate is determined based on the on-line measurement of the biomass produced in the culture. For the above, the kinetic behavior of the culture is recorded in time intervals and the amount of biomass formed in the evaluated time period is determined. From these results and with the YX/S yield of the culture, the substrate flow required to feed is predicted to produce the same increase in biomass concentration in a similar time period ΔX2 = X1.
The exponential substrate flow pattern (dF/dt) was established for cultivation with a time interval of 30 min. The different feeding profiles were defined according to the variation of the µ parameter. The nutritional requirements of the strain were guaranteed by the addition of the other nutrients from the culture medium, maintaining the concentrations defined in the MMS medium. The nitrogen source (NH4 +) was provided by the 28% NH4OH supply and used for pH control, as reported in the literature [9,18,19].
2.6. Culture evaluation using predictive substrate feeding
The model was derived in order to maintain the concentration of residual substrate at desired level. The model predicts the substrate flow required to produce a defined amount of biomass (ΔX2) at a later time interval, assuming the growth trend produced by the microorganism in the immediately preceding time interval (ΔX1) as constant (Fig. 1). The rate is determined based on the online measurement of the biomass produced in the culture. For the above, the kinetic behavior of the culture is recorded in time intervals and the amount of biomass formed in the evaluated time period is determined. From these results and with the YX/S yield of the culture, the substrate flow required to feed is predicted to produce the same increase in biomass concentration in a similar period of time.
2.6.1 Model considerations
The substrate concentration of the culture medium remains constant
The biomass concentration formed in the feeding period will be equal to that obtained in the previous interval
The change in the volume of the culture medium between two immediate feeding points is minimal or close to zero
2.6.1 Balance for biomass
Since the feeding period is defined for a short time interval and considering that the substrate concentration of the feed is high, it can be assumed that the change in the volume of the culture medium between two immediate feeding points is minimal, therefore, you can assume that, in this way:
Considering the death rate to be negligible and assuming a Monod-like behavior and that, therefore, ( remains constant and can be equalize to the dilution rate
assuming a pseudo-stationary state.
Balance for substrate:
Assuming there is no substrate accumulation, then:
Since the product is part of the biomass and its formation is related to growth, the balance can be simplified by assuming that the substrate is consumed for biomass formation and maintenance is negligible, thus solving for X:
By replacing equation (7) in equation (4), the relationship between the flow of substrate that must be added to the system to form an estimated biomass concentration at a given time can be established.
Where:
F: substrate flow during the feeding period (ml/min).
YX/S: biomass yield per unit of substrate (g/g).
𝛥X: estimated biomass concentration to be formed during the feeding period (g/L).
𝛥t: feeding period time (h).
Si: feed substrate concentration (g/L).
V: volume of the culture at the end of each feeding period (L).
Where:
V0: reactor volume at the start of each feeding period (L).
f: substrate flow used in the immediately previous feeding period (ml/min).
2.7. Quantification of biomass
Growth was determined by optical density (OD600nm) and dry weight biomass was determined by gravimetry. Samples of 5 mL were taken and filtered through a membrane using 0.45 µm cellulose filters. Samples were dried at 65ºC to constant weight.
2.8. Sugar concentration
It was quantified by high performance liquid chromatography (HPLC) on an Agilent Technologies 1200 chromatograph, model 61362A. Samples were centrifuged at 5000 rpm for 7 minutes and diluted in 0.008 N H2SO4 mobile phase. They were then injected under the following conditions: column temperature 35 °C, flow rate 0.6 mL/min and run time 12 min.
2.9. Polymer extraction
To extract the polymer, the acid digestion method reported by Acosta et al. (2018) [20] was used. To recover the biomass, the samples were centrifuged at 5000 rpm for 12 min. The resulting pellet was diluted in 200 mL of distilled water and autoclaved at 121 °C, 1 atm for 30 min. It was centrifuged again and the recovered pellet was mixed with 200 mL solution of 0.1 M sulfuric acid. It was then heated at 90 °C for 2 h, after which the pH was adjusted to 10 with 5 N NaOH. The pellets were centrifuged for recovery, washed with water and then suspended in a 2:1 solution (distilled water: 6% v/v hypochlorite) for 2 h at room temperature. The polymer was recovered by centrifugation under the same conditions and dried in an oven (65°C) until a constant weight was reached.
2.10. Polymer identification
It was characterized by infrared (IR) spectroscopy using a Pelkin-Elmer Spectrum BX infrared spectrophotometer. A 99% purity PHB (polyhydroxobutyrate) standard from Sigma-Aldrich was used as a control sample to identify the characteristic functional groups of this biopolymer.
3. Results
3.1. Influence of the C/N and C/P ratio
The results obtained show that growth is favored as the amount of nitrogen (a) and phosphorus (b) available increases (Fig. 2). In the 3 lower ratios, the behavior was similar and a higher amount of biomass was obtained, approximately 3.1 g / L. In the higher ratios, the biomass produced was lower, indicating that in these conditions, growth is limited due to the lower availability of these nutrients. In the specific growth rate (µ), no statistically significant differences are observed between the different conditions. This indicates that the growth rate of this bacteria is not dependent on the concentration of nitrogen or phosphorus in the medium, but the concentration of biomass formed is dependent on the availability of these two nutrients. In relation to the yield of biomass / substrate (YX/S), it is shown that the capacity of use of the substrate for the formation of biomass is also affected by the availability of nitrogen in the culture, being the highest yield in C/N less than 6.9; however, in the C/P ratios, there are no significant differences in this parameter in any of the treatments evaluated, this indicates that there is no correlation between the availability of phosphorus on the ability to use the substrate towards biomass formation by these bacteria. In polymer formation, a higher concentration (≈ 1.6 g/L) was obtained in the lower ratios, that is, when the microorganism was not limited by nitrogen or phosphorus, but when evaluating the ratio between polymer/biomass (YP/X), no differences are shown in the different C/N and C/P ratios. This result suggests that the formation of PHAs in these bacteria can occur both under conditions favorable for growth and under nutritional imbalance, since its formation is not conditioned by nutrient limitation and occurs in the same way under conditions of availability. This aspect is important for the implementation of cultivation strategies, since it would not require separate stages of growth and accumulation, and could be carried out simultaneously, shortening process times.
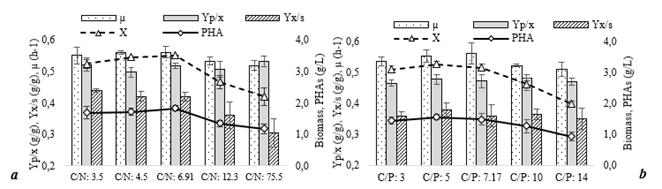
Source: The authors
Figure 2 Effect of the C/N (a) and C/P (b) ratio on the formation of biomass (X), polymer (PHAs), specific growth rate (µ) and YX/S and YP/X yields.
3.2. Polymer identification
The results of the infrared spectrum show a 99.26% correlation between the absorption bands of the C=O and CH3 functional groups (region 1740 cm-1 and 2970 cm-1, respectively) of the obtained polymer and the PHB standard (Fig. 3). This biopolymer has a high crystallinity (>50%), a glass transition temperature of 4°C and a melting temperature of about 180°C. It has been reported to have mechanical properties similar to synthetic polyesters such as polylactic acid (PLA) [21,22]. Due to its biocompatibility, this biopolymer is an interesting material for tissue engineering as it has been shown to enhance the culture of various cell types and can be mixed with other types of biocompatible molecules to form biofilms [23,24].
3.3. Dissolved oxygen evaluation
The results of the effect of dissolved oxygen concentration and air flow on the growth of this bacteria are shown in Fig. 4. A positive effect of increasing the O2 concentration on the formation of biomass (A) is shown, reaching approximately 8 g/L at values between 30 and 40% dissolved oxygen. This behavior is similar to that reported in the literature, where it is shown that the growth of PHA-producing bacteria is favored with high oxygen availability in the culture [11,25]. However, above 40% saturation, a tendency to decrease biomass production is observed, possibly indicating a state of oxidative stress at higher concentrations. Similarly, the specific growth rate of the bacteria is favored with increasing O2 concentration (B).
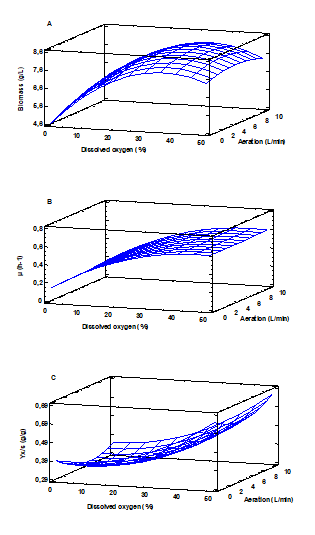
Source: The authors
Figure 4 Evaluation of dissolved O2 concentration and aeration on biomass formation (A), specific growth rate (B) and biomass substrate yield (C).
From an O2 saturation of 40%, the maximum growth rate is reached (≈ 0.6 h-1). When evaluating the YX/S performance (C), a positive effect on the substrate conversion capacity towards biomass formation with the increase of O2 is also demonstrated, reaching the highest yield (0.55 g/g) in higher concentrations at 40%. Regarding the relationship between aeration and O2 concentration, no statistically significant correlation is observed between both factors. This indicates that, under the conditions in which the tests were carried out, these factors do not correlate, which explains why there is no evidence of a dependence between aeration and the kinetic parameters evaluated. As shown, both the growth, the specific growth rate and the ratio of the biomass formed to substrate consumed are not affected by changes in the supplied air flow. These results are in contrast to those reported by Kshirsagar et al. (2012) [26], where there is a dependence on growth with air flow.
Comparing the effect of the O2 concentration on the growth and substrate consumption kinetics (Fig. 5), it is evident that the dissolved oxygen concentration is a determining factor in the process, as it affects the culture times. When a low oxygen saturation (4%) is used, the kinetics of both biomass formation and substrate consumption are slow, reaching a final biomass concentration of 5.5 g/L after 36 h and leaving a residual substrate of approximately 4 g/L. As the O2 concentration of the medium is increased, the growth and substrate consumption kinetics are favored and the culture times are shortened. When an oxygen saturation close to 40% is used, the highest amount of biomass (8 g/L) is obtained, improving the yield in the same way, as explained above. In turn, under these conditions the process times are significantly reduced requiring only 12 hours of cultivation (vertical line spacing). The above is relevant for the production of PHAs since both productivity and process performance are improved.
3.4 Cultures with exponential and predictive feeding
Fig. 6 describes the behavior of growth kinetics and residual substrate in cultures with exponential and predictive feeding. The results show that it was possible to increase the biomass concentration to 16 g/L compared to the batch culture (8 g/L), when using the exponential model and a substrate feeding profile calculated with a ( of 0.6 h-1 were used (A). However, it is evident that the bacteria stop growing after 3 hours of feeding (9 hours of culture). This behavior is associated with a possible substrate inhibition effect. The above is based on evidence from previous studies related to the production of PHAs, where the growth of the microorganism was negatively affected at substrate concentrations greater than 25 g/L [27,28]. When the culture was evaluated with a feeding profile with ( = 0.4 h-1 (B), the biomass concentration was doubled with respect to the previous results, reaching a value close to 31 g/L, however, from at 11 h of culture (5 h of feeding) an increase in the residual substrate concentration above the inhibition point is observed and it is again proven that growth stops. In the case of the results obtained with the predictive feeding strategy (C), it is observed that the residual substrate concentration is maintained below the inhibitory concentration of the microorganism throughout the culture. Under these conditions, a biomass concentration of approximately 41 g/L was obtained at the end of the process, improving by 25% that obtained with the exponential feeding strategy. Although the biomass production was improved with this model, however, it is evident that the growth rate of the bacteria decreases after 11 h of cultivation, reaching seasonality when a concentration close to 41 g/L is obtained. Since the residual sugar concentration in this culture could be controlled, this is not related to inhibition by substrate or nutritional stress, as this bacterium had nutrients available to continue its growth. The causes could be attributed to the accumulation of inhibitor products derived from the bacteria´s own metabolism. Although this behavior could limit the obtaining of better results in this type of culture systems, the ability of this bacteria to simultaneously produce PHAs during its growth, would allow the future implementation of cultures in continuous systems, which could increase the productivity of the process.
In the production of PHAs (Table 3), when the exponential model was used, 6.3 and 18.1 g/L were obtained (for ( = 0.6 h-1 and ( = 0.4 h-1, respectively). With the predictive feeding model, production was improved and 24.1 g/L of polymer was obtained. In terms of PHAs productivity, a maximum of 0.75 g/L.h was obtained with the reference model, which is lower than that obtained with the predictive model, which was 1.01 g/L.h of polymer. In terms of process yield, there were no differences between the two models, with a substrate to polymer conversion (YP/S) of 0.30 g/g and a YX/S of about 0.5 g/g. Regarding the percentage of PHAs accumulation, a value close to 60% of polymer accumulation with respect to the total biomass weight was achieved with both models.
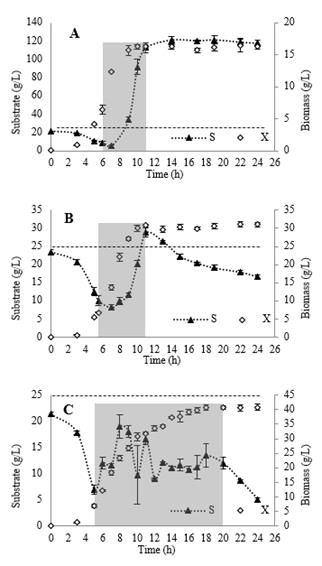
Source: The authors
Figure 6 Growth kinetics and substrate profile obtained by using exponential feeding (A: µ = 0.6 h-1 and B: µ = 0.4 h-1) and predictive feeding (C). The dark gray zone indicates the feeding period. The spaced horizontal line indicates the limit substrate concentration for the microorganism.
When comparing these results with the reports found in the literature [4], where biomass and polymer concentrations higher than 200 g/L are shown by commercial or genetically modified strains, the results obtained in this study although they are low, they are promising if they are considered to have been obtained with a native strain. In addition, the kinetic characteristics of this bacteria, in terms of its rapid growth and having a polymer accumulation capacity that does not depend on conditions of nutritional stress by nitrogen or phosphorus, makes the times required for its cultivation shorter. The above would allow the implementation of cultivation strategies in continuous systems, since it does not require independent stages of polymer growth and accumulation. This last aspect is very important because the cultivation times affect the productivity of the process. As an example Špoljarić et al. (2013) [29] (47 g/L of biomass and 30 g/L of PHAs) and Shang et al. (2008) [30] (103 g/L of biomass and 28 g/L of PHAs), who obtained a larger amount of biomass and polymer than those obtained in this research, however, the culture time was longer, causing the productivity achieved to be only 0.33 and 0.61 gPHAs/L.h, in 91 and 48 h, respectively, lower than that obtained in this study (1.01 gPHAs/L.h).
Table 3 Parameters obtained for the culture with exponential and predictive feeding.
Feeding Parameter | Exponential (( = 0.4 h-1) | Exponential (( = 0.6 h-1) | Predictive |
---|---|---|---|
X (g/L) | 16.3 ± 0.35 | 30.9 ± 0.54 | 40.9 ± 1.12 |
PHAs (g/L) | 6.3 ± 0.96 | 18.1 ± 1.33 | 24.1 ± 1.14 |
YP/S (g/g) | 0.17 ± 0.04 | 0.30 ± 0.02 | 0.30 ± 0.012 |
YX/S (g/g) | 0.43 ± 0.05 | 0.51 ± 0.01 | 0.48 ± 0.012 |
%PHAs | 38 ± 4.8 | 58 ± 2.7 | 59 ± 1.2 |
P (g/L.h) | 0.26 ± 0.04 | 0.75 ± 0.06 | 1.01 ± 0.047 |
X = biomass; PHAs = polymer; YX/S = biomass yield per unit of substrate; YP/S = product yield per unit of substrate; YP/X = product yield per unit of biomass; P = polymer productivity.
Source: The authors
4. Conclusions
The applied methodology allowed to determine the nutritional requirements of nitrogen and phosphorus, which improved the growth of the bacteria, as well as the apparent independence in its limitation for the accumulation of the polymer. Regarding the oxygen demand, it was possible to determine the saturation necessary for the process, improving the growth and production of PHAs by significantly reducing the culture times.
The predictive feeding strategy proposed in this research allowed to improve the results of PHAs production with respect to the exponential model, reaching a 25% increase in both biomass concentration and polymer productivity obtained and becomes a good alternative for the control of the feeding of substrate in cultures in fed batch, since it integrates as much the use of an alternative substrate, the use of a native bacteria, as the development of a strategy of cultivation in fed batch that allows to improve the productivity of the process.