1. Introduction
In general terms, geothermal energy comes from the heat generated in the Earth’s core, which can be used on the surface - directly or indirectly - in a variety of fields like health and wellness, agricultural methods, heating and cooling, industrial processes, and electric power generation, depending on the temperature of the resource [1]. Shallow geothermal energy generally refers to all the energy stored in the first 400 meters under the ground, with average temperatures between 10 and 30 °C [2], where usually Ground Source Heat Pumps (GSHP) can be installed.
GSHP are thermodynamic devices that use the relatively constant temperature of the ground as a medium to exchange heat, presenting a higher performance compared to conventional heating and cooling systems. In fact, soil provides higher temperatures than air for heating, and lower temperatures for cooling, and shows smaller daily temperature fluctuations than those observed in the air [3].
Their use is becoming increasingly popular globally as the development of new technologies, scientific knowledge and awareness increase [4], making possible for geothermal energy to be used directly as a simple way to contribute to energy efficiency, with multiple advantages, such as reductions in electricity consumption for air conditioning, low maintenance costs, and contribution in reducing greenhouse gas emissions from other heating methods [5]. However, it is necessary to implement regulations on their use, since there is a constant growth in the number of geothermal heat pump installations. In 2020, approximately 6.46 million geothermal heat pump systems (typically domestical installations, with powers around 12 kW) were estimated worldwide and a 54% increase over the number of installed units reported in 2015 [6]. European countries consistently have the greatest number of installations and take four criteria into account for their legislation on the sustainable use of GSHPs: accurate, technical drilling and installation methods; filling material of boreholes for ground-source systems; minimum distances between installations; and temperature thresholds [18]. However, there is still a lack of homogeneity in the legal definition of shallow geothermal energy between each european country and is one of the reasons why regulatory frameworks may vary between them. In Europe, Sweden is the leader in terms of installed geothermal heat pumps, with estimated installations of more than half a million in 2017. Germany and France follow with 0.38 million and 0.16 million, respectively, while Finland and Austria have around 0.11 and 0.1 million installations [26]. In Colombia, as in different European countries, the application of GSHP is linked to some fundamental principles of sustainable development regarding land use, drilling techniques and groundwater management, areas in which different regulatory entities participate, mainly the ministry of mines and energies and the ministry of environment and sustainable development. These regulations are not designed directly for shallow geothermal applications but should be considered when designing a system such as GSHP that may require drillings or groundwater extraction. Table 1 shows some of the main laws and decrees in Colombia related to GSHP use.
Table 1 Laws related to practices involved in the process of installing and operating a system with GSHPs.
Law | Description | Closed loops | Open loops |
---|---|---|---|
Law 373 of 1997 | The program for the efficient use and saving of water is established. | ( | |
Decree 2811 of 1974 | The National Code of Renewable Natural Resources and Environmental Protection is issued. | ( | ( |
Decree 1541 of 1978 | Regulations for the exploitation of groundwater are defined and obliges to obtain a concession for the exploitation of the Ground Water Resource (GWR). | ( | |
Decree 1594 of 1984 | The sanctioning procedures applicable to the GWR are defined. | ( | |
Decree 155 of 2004 | Methodology to determine the rate for the use of Groundwater is defined. | ( | |
Decree 1640 of 2012 | Instruments for planning and managing of hydrographic basins and aquifers are regulated. | ( | |
Resolution 815 of 1997 | It is obligatory to implement a measurement system for the exploitation of GWR. | ( | |
Resolution 250 of 1997 | Annual determination of static and dynamic levels and physicochemical monitoring of groundwater are required. | ( | |
Resolution 2173 of 2003 | Rates are set for the collection of evaluation and monitoring services for environmental licenses, permits, concessions, authorizations and other instruments for environmental control and management. | ( | ( |
Resolution 240 of 2004 | The minimum fee for water collection rates is defined | ( | |
Resolution 5589 of 2011 | The procedure for charging the environmental evaluation and monitoring services is set. | ( | ( |
Agreement 10 of 1989 | Rules are issued to manage the water for public use in the area of the Regional Autonomous Corporations (RAC) | ( |
Source: The authors
In Colombia, these types of applications are not common yet mostly due to the lack of knowledge and regulations regarding shallow geothermal energy and its use in tropical climatic conditions. In the tropics, the seasonal temperatures’ range is relatively lower compared to those ranges observed in places at higher and lower latitudes, bringing a considerable amount of uncertainty on the installation of GSHPs and their efficiency under these conditions, since GSHPs generally work better when the difference between underground and outside temperatures is greater [7].
Only one shallow geothermal project of this type has been executed in the country, according to the report No. 12 of December 31, 2019, issued by the Mining and Energy Planning Unit [8]. It was a pilot project in Tocancipá, Cundinamarca, where the company SAGG S.A.S. installed a cold room using a heat pump coupled to two 80 meters deep vertical heat exchangers, using a closed system, with a power of 12 kW, representing energy savings up to 75% of the total energy consumption for cooling at -10°C [9].
This work presents a methodological framework for the design of a GSHP, together with the review of the regulatory conditions in Colombia for these types of systems. A prototype installation at the University Escuela de Ingeniería de Antioquia (EIA) for the air conditioning of a classroom through GSHP is described and constitutes the first work that describe all the steps required for the design of this type of system for the study case mentioned. Moreover, this work seeks to present all the necessary information to familiarize the reader with these types of installations and to explain the benefits of renewable energies, such as geothermal. The results and recommendations could be applied to the design of any type of these installations in the Caribbean and Latin America region, where these systems are not well known yet, but where geo-thermal industry development is continuing to show huge promise [10]. Moreover, the use of geothermal resources contributes to achieve the Sustainable Development Goal #7, which aims at ensuring the access to affordable, reliable, sustainable, and modern energy expanding and upgrading energy services for developing countries and increasing the global percentage of renewable energy consumption [11].
2. Methods and materials
2.1Location of the study area
The EIA University campus Las Palmas is located at the kilometer 2 + 200 of the José María Córdova Airport Bypass, in the El Penasco village belonging to the municipality of Envigado, in the Antioquia department (Fig. 1).
According to the study of soil properties carried out at the EIA University [12], the geology of the area corresponds to residual soils derived from amphibolic rocks that have been covered by volcanic ash, lake deposits, and anthropic fills, with a water table found approximately at 2.5 meters below the surface.
3. Methodology
There are three fundamental stages for a shallow geothermal installation: design, installation, and monitoring. The correct design and planning allow us to reduce installation and operation costs, depending on the level of detail taken when observing the conditions of the place and the different variables present during the process. Fig. 2 shows a schematic illustration of the design process for a GSHP system.
3.1 Calculating the cooling loads
Cooling load calculation is based on two main principles: first, recognizing the thermal loads of the space and calculating their values, and second, by identifying the factors that most influence these values to determine strategies that could reduce them. It should be noted that a thermal load is the amount of heat that must be extracted or added to the target space [13], and the more detailed these values are computed, the more sustainable and efficient a Heating, Ventilation and Air Conditioning (HVAC) system can be designed.
The main external heat sources are the outside air temperature and solar radiation, calculated in eq. (1), taken from [14].
Where Q (W) is the heat gain, U (W/m2 °C) is the thermal transmittance of the conductive material, A (m2) is the area of the surface and CLTD (°C) refers to the “Cooling Load Temperature Difference”, which is defined as the difference between the outside temperature and the interior of the space. The CLTD for the case study was defined by measuring the external and internal temperatures at four different times during the day with average 3°C, while U was defined as 0.718 W/m2 °C for concrete, with a total area of 210 m2 accounting for the walls, floor and ceiling of the room, and a U of 5.2 W/m2 °C for glass with 6 m2 of windows [13].
For the calculation of internal loads, all the agents that provide heat must be considered, mainly the people and equipment that operate inside the space in this case. The value for the internal heat gains is calculated by the eq. (2).
Where Q is the heat gain in Watts, N is the number of internal agents and W (W) is the heat produced by each internal agent. The total cooling load is finally obtained by adding the resulting values of all the internal and external heat loads. For the case scenario of a full room, the number of people and computers was assumed as 25, maximum capacity of the room, and 4 ceiling lights, with people producing around 132 W, computers 169 W, and lights 130 W [13].
3.2. Selecting a heat pump
Once the peak load of the space to be air-conditioned has been identified, a heat pump that can satisfy this demand must be selected. GSHPs can generally work for both heating and cooling and can be found in a different range of operating powers. This selection process requires identifying the technical sheet of each heat pump to define important characteristics such as its power, type of electrical supply, temperature limits, coefficient of performance (COP), Energy Efficiency Ratio (EER), among others.
It is important to note that the placement of the heat pump during its installation should be near the heat exchanging system to prevent cost overruns.
3.3. Selecting a heat exchanger
After having selected a heat pump with enough power to meet the energy demand for the space, the installation should be configured either as a Ground Source Heat Pump (GSHP), Ground Water Heat Pump (GWHP) or Surface Water Heat Pump (SWHP), depending on the availability of resources such as sufficient land area, groundwater, or surface water.
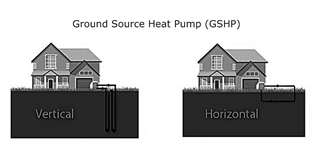
Source: The authors.
Figure 3 Schematic illustration of vertical and horizontal Ground-Source Heat Exchangers.
Ground Source Heat Pumps: both vertical and horizontal systems use arrangements of pipes in which their lengths and distributions on the ground will define the real efficiency of the entire system, thus the costs associated with its operation, as well as initial costs for the installation of the heat exchanger, given the amount of piping, fillings and excavations or drillings (Fig. 3).
Ground Water Heat Pumps: these types of systems work in a very similar way to the ground-source ones, with the difference that the heat exchange occurs directly between the heat pump and the extracted groundwater. Generally, they are the most efficient heat exchangers when the real extraction rate can meet the design values, only when the hydrogeological conditions of the reservoir allow it [4] (Fig. 4).
Surface Water Heat Pumps: these systems require much lower upfront costs than the previous ones, since no type of drilling or excavation is needed to access the heat exchanging medium. It can use a direct or indirect heat exchanger (as shown in Fig. 5) in superficial bodies of water such as rivers, ponds, or lakes, with enough volume and depth to ensure constant temperatures at the bottom.
Understanding each of these system’s advantages and disadvantages in relation to the project’s location and availability of resources is key to a good design.
When choosing an exchange system that best suits the physical space available, it is also important to consider legal aspects that can increase costs and slow down the development of the project. For example, in European countries, where this type of systems is widespread, there are different regulations and guidelines around the installation and operation of ground source heat pumps, both legally binding and formal recommendations [15]. In most cases in which their use has been regulated, a pattern can be observed: the recommendations given are based on scientific knowledge that promotes the correct operation of the heat pump, allowing the conservation of its efficiency throughout its useful lifetime, ensuring that the installation does not affect other structures or nearby facilities, nor the conditions of the physical space where it is located, following technical and adequate drilling techniques [16]. Likewise, the laws that cover shallow geothermal energy could be considered into two main categories: those laws related to the drilling process and its correct technique, which generally fall under the responsibility of the entity in charge of regulating mining practices in the country; and second, the laws associated with the extraction of groundwater and the management of aquifers, which are primarily the responsibility of environmental entities [17,18]. Both categories can be supervised by national, regional, or local authorities. In Colombia there is no important information on impact studies or direct regulatory proposals on the implementation of projects using shallow geothermal energy [8].
Once the legal aspects for the selected system are assessed, the design and calculation of the total length of the heat exchanger can be carried out. For this case study, a closed loop horizontal system, often called “slinky” due to its configuration, was selected given the available terrain next to the room shown in Fig. 1. The equation used to calculate this length is shown by eq. (3) [19].
Where L (m) is the total length for the buried piping, Qcond (W) refers to the heat pump’s condenser power in cooling mode calculated with eq. (4), Rt (m∙°C/W ) is the total thermal resistance of the system that will be calculated with eq. (5), ELT and LLT (°C) are the Entering Liquid Temperature and Leaving Liquid Temperature, respectively, which can be calculated with eq. (6), and finally Tg (°C) refers to the ground’s temperature at 3 m of depth, calculated with eq. (7).
In eq. (4) the EER is the heat pump’s Energy Efficiency Ratio and Qcr (W) is the peak cooling load calculated for the target space. These parameters can only be obtained after computing the peak cooling load and choosing the correct heat pump.
In eq. (5) the Rp (m∙°C/W) is the thermal resistance of the pipes, Rg (m∙°C/W) is the thermal resistance of the ground, Fc is a usage factor given by the total hours of work per month and Is is a trench spacing multiplier. In this case, the Rp was taken as 2.33 m∙°C/W for High-Density Polyethylene pipes with an internal diameter of 17 mm [24], which are commonly used for these applications due to their relatively high thermal conductivity and mechanical resistance. For Rg the taken value was 0.43 m∙°C/W given the ground was previously defined for the campus as inorganic silts with high plasticity, saturated, for which a thermal conductivity corresponds to approximately 2.3 W/m°C, based on the information obtained from [23]. The usage factor Fc determined for the heat pump was defined as 0.21, meaning six working hours per day, six days per week and an Is of 1.2 for trench spacing of 2 meters between them [19].
Where ELT (°C) can be found in the heat pump’s specifications and V ̇ (l/min∙W) is the circulating water’s flow rate inside the piping. From the recommendations given by [4], the Entry Liquid Temperature (ELT) was defined as 17 °C higher than the ground’s temperature, resulting in an ELT = 31.3 ° C, and a °V of 3 l/min∙W to avoid turbulent flow.
Where Tm (°C) is the average annual temperature of the air, As (°C) is the difference between the maximum and minimum annual temperature value of the air, X (m) is the depth of the ground and α (m2/s) is the thermal diffusivity of the ground. At the campus, the Tm is around 17 °C and an absolute maximum and minimum annual values of 28.8 °C and 5.4 °C, respectively [25], with a depth of 3 meters and an α of 6.77·10-7 m2/s, previously defined by [23].
3.4. Selecting an emission system
The designer can choose between a radiator or fan-coil emission system. In case of having a space that requires little energy demand, radiant soils or walls are ideal as a variation of the radiators and should be in the internal envelopes of the space to be air-conditioned that have the greatest contribution within the values of thermal loads [20]. These options are the most recommended systems when planning an HVAC system with GSHP for homes and commercial buildings.
4. Results
The cooling load was computed approximately based on two main assumptions: first, the cooling demands are limited to an hourly range between 10 h and 14 h, due to the increase of the exterior temperature based on the highest daily observed temperatures happening between those times. Second, the occupancy within the selected time range is maximum and all equipment is in operation.
For internal loads, computer equipment, people, and lights were considered, and the variables used for external loads were heat conduction through walls, floors, roof, and windows, as well as solar radiation through windows. The approximate results of the total cooling load for different times of the day are presented in Table 2.
The highest value is observed at 16 h, corresponding to 9,646 W (9.65 kW) (Table 3), for which a 1-9 kW “EcoGEO Basic” GSHP from the “Ecoforest” [22] brand is selected to supply the demand for air conditioning. Other brands can also be selected. In this case Ecoforest has been chosen as example since the information is easily accessible and free training courses are regularly held by the company.
Table 2 Resulting cooling loads at four different moments in one day.
Time of day | 10 h | 12 h | 14 h | 16 h |
---|---|---|---|---|
Cooling load (W) | 8,549 | 8,563 | 8,853 | 9,646 |
Source: The authors.
Table 3 Results from each variable affecting the total heat load value at 14h.
External Loads | ||
---|---|---|
Radiation through windows | 776.88 W | |
Conduction through windows | 156 W | |
Conduction through walls | 402.08 W | |
Conduction through the ceiling | 90.405 W | |
Conduction through the floor | 175.91 W | |
Internal Loads | ||
People | 3300 W | |
Computers | 4225 W | |
Lights | 520 W | |
Total Loads | 9,646 W |
Source: The authors.
Table 4 Distribution of the heat exchanger’s piping.
Number of trenches | 3 | 6 |
Piping length per trench (m) | 474 | 217 |
Area per trench (m2) | 130 | 60 |
Total required area (m2) | 911 | 952 |
Width x length (m) | 7 x 130 | 16 x 60 |
Source: The authors
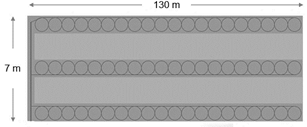
Source: The authors.
Figure 6 Schematic representation of the piping layout for the initial configuration of three trenches.
The availability of land next to the target room, as seen in Fig. 1, and the relatively low cooling demand for a single classroom, suggest that the best suitable heat exchanging system would be a ground source one, using the “slinky” configuration for piping to save space in the trenches, thus reducing upfront costs.
The heat exchanger’s length was computed using eq. (3). The first parameter considered was calculated from eq. (4), using a maximum cooling load of 9,646 W (taking the maximum value) and an EER of 5.2 for the selected heat pump, resulting in a value of 15,978 W for Qcond. The result from eq. (5) for the total thermal resistance of the heat exchanger was equal to 2.44 m°C/W. From eq. (6), the result for the Leaving Liquid Temperature LLT was approximately 38.49°C, and finally, from eq. (7), the minimum ground temperature resulted in around 13.3 °C.
These variables yielded a heat exchanger length value of approximately 1,301 m, which allowed to propose a distribution between three or six trenches, depending on the availability of land, for horizontal “slinky” type piping with a diameter of 1 m and a separation or “pitch” of 1 m. The separation between each trench was defined as 2 m, based on the tables provided by [19] and the depth was proposed at 3 m, below the water table, seeking to submerge the piping to improve the thermal conductivity of the ground. The area required for each distribution is presented in Table 4, with a schematic representation of the piping layout in Fig. 6.
5. Discussion
The total required area for the horizontal GSHP installation is an acceptable value, considering the available land at the site. An alternative design would be the vertical GSHP, which requires less surface but has a higher cost due to the need for drilling. For the horizontal design, the total installation cost would be affected by the length of the heat exchanger. Therefore, a sensitivity analysis was conducted to show the variability of the total pipe length when the most sensitive variables (soil temperature, ELT, LLT, and pump power) vary within a specified range, while keeping the other parameters constant.
Table 5 Variability of total heat exchanger length for ranges of input variable values.
Variable | Range | Heat exchanger length |
---|---|---|
Soil temperature | [11 - 15] °C | [1,190 - 1,433] m |
ELT and LLT | [29 - 33] and [36 - 40] °C | [1,456 - 1,206] m |
Pump power | [8,646 - 10,646] W | [1,186 - 1,411] m |
Source: The authors.
Besides the technical aspects, it is also essential to note that regulatory framework can also play a main role when considering the implementation of these kind of technologies. For example, projects using GSHPs in Colombia could benefit from the law 1715 of 2014 and its new update, law 2099 of 2021, which promotes incentives to all of those technologies that use non-conventional renewable energies (FNCE), such as income tax deductions of 50% of the investment, VAT (Value Added Tax) exclusion for equipment, parts, machinery, and services that are intended for pre-investment and investment for energy production, exemption from payment of custom fees on the importation of machinery, equipment, materials, and accelerated depreciation of assets necessary for generation with FNCE [8]. The recent Decree 1318 of 2022 that regulates the generation of electricity using geothermal resources is also part of the current regulatory framework, but this does not apply to a GSHP system.
6. Conclusions
Shallow geothermal systems used in domestic installations such as GSHP for air conditioning of spaces, are an economical option in the long term, especially for large installations. The systems described in this work have characteristics that must be studied in detail to conduct a comparative analysis between them, since the conditions of the ground, groundwater, and surface water sources will determine the installation capacity of a system with an adequate, cost-efficient, and sustainable GSHP. One of the main challenges that arise when designing these systems is the variety of disciplines that must converge during this process, so it is recommended to review this work as a prototype of an air conditioning project for the EIA University, which can be scalable using the concepts presented here. For this case study, the estimated heat exchanger length for a horizontal GSHP was estimated approximately to be between 1,190 and 1,433 m, considering alternative ranges of soil temperature, Entering Liquid Temperature and Leaving Liquid Temperature (Table 5).
The results obtained suggest that the area required to install the pipes in the ground is slightly greater than the available land next to the building where the target room is located. However, it is possible to determine the area of land available more accurately in the field, and propose another type of geometry for the trenches, either defining curvatures or simply reducing the pitch for the slinky piping. Some recommendations to continue this work and provide a more precise design are:
To accurately estimate the cooling load of the space to be air-conditioned. This process can be delegated to qualified personnel for the design of HVAC systems who use specialized software, allowing the designer to effectively come up with strategies to reduce energy demands and implement them.
Measuring the ground temperature and soil thermal properties, to better adjust the system to real local conditions. This recommendation applies not only for the development of this project, but it would also help to promote the application of shallow geothermal systems in Colombia with available ground temperature maps. Furthermore, the same strategy should be applied to all the countries where shallow geothermal systems are a valuable option to reduce energy costs and meet environmental goals.
To use specialized software both for the calculation of the length of the heat exchanger system, and for the economic and environmental analyzes of the project, to allow the long-term validation of energy savings and equilibrium conditions. Some programs even allow to configure the geometry of the piping, in case of being a closed system, although this process can be left to the discretion of the designer.
To consider the economic incentives for FNCE according to Colombian Laws 1715 and 2099, once the economic feasibility stage of the project is reached. Regulatory framework needs to be considered mainly for drilling, water extraction and groundwater use, when proposing these kinds of systems in Colombia. However, the country still does not present laws to measure and regulate the impacts of GSHPs as energetically efficient mechanisms related to geothermal energy.