INTRODUCTION
Medicinal plants are one of the oldest forms used for the treatment of diseases, due to the healing properties of substances present in certain species [1]. A plant is considered as medicinal when it has active substances that provoke in the human body reactions that can range from cure to disease relaxation. Medicinal plants have a promising world market in the face of growing demand [2].
However, there is a lack of studies that is reflected in the frequent lack of quality of the product offered, making it commercially less competitive [3]. The medicinal plant of the species B. orellana L., family Bixaceae, produces fruits called annatto, whose popular name originates from the Tupi word "uruku", which means "red". It is a shrub originating in Central or South America, more specifically from the Amazon region, which grows spontaneously from Guyana to Bahia [4].
In this sense, aiming at postharvest quality, including the durability of the compounds, it is important that B. orellana L. has its water content reduced soon after harvest. Drying, therefore, is one of the fundamental stages of post-harvest, being directly linked to the quality of medicinal plants. In the case of medicinal plants in which they have the presence of essential oils (EOs), drying has a direct influence on sensitive components [5].
Drying and storage are fundamental steps among the post-harvest processes for obtaining quality products, which are the basis for the manufacture of herbal medicines. Drying is a complex procedure due to the structure and composition of the material, the transport phenomena involved and the biological variability. The drying rate depends on variables such as temperature, speed, relative humidity of the drying air and product characteristics. Thus, mathematical modeling and simulation of this process are useful tools to deal with its complexity and obtain adequate operating conditions [6,7].
Therefore, the positive and negative effects of the drying process depend on the conditions and according to the sensitivity of the chemical substances, which can be lost by volatilization, as is the case of Eos [8, 9]. In order to obtain greater knowledge about the effect of drying on the chemical composition of medicinal plants, it is necessary to make use of specific analyses. In the case of EO, it is regularly recommended to use the gas chromatography system coupled to the mass spectrum (GC/MS), in order to identify substances derived from secondary metabolism [10, 11]. Therefore, it is of fundamental importance to know the sensitivity of the active ingredient of plants and the adequacy of drying conditions to their quality in the final product.
In addition, EO's are used in topical formulations. Because they have antimicrobial action, they can inhibit bacterial growth and promote the wound healing process, providing a better alternative to treatment [12]. EOs extracted from medicinal plants have been widely used successfully in research aimed at controlling phytopathogens. It presents as secondary metabolites with naturally volatile substances that interact with each other, forming classes of compounds with microbial potential, especially terpenes, monoterpenes, sesquiterpenes, aromatic compounds, phenols, aldehydes, ketones, alcohols and esters [13, 14].
These various combinations of chemical constituents presented by EOs can control food oxidation and bacteria that have consistently high resistance to antimicrobial agents such as Salmonella cholerasuis, Listeria monocytogenes, Staphylococcus aureus and Escherichia coli. Although the EO's represent an interesting possibility for the preservation of food, it is necessary to know its antibacterial and antioxidant properties [15,16]. In this context, this work aimed to evaluate in an unprecedented way the influence of drying air temperature, as well as to adjust and statistically model the drying curves of the leaf, in addition, to estimate the yield and toxicity of the EO of the leaf by B. orellana.
MATERIALS AND METHODS
Collection of plant material
The fresh leaves of B. orellana L. to perform the drying kinetics were collected in the Herbarium Ático Seabra of the Federal University of Maranhão under the register of n° 00815, in the city of São Luís-MA in July 2019. The leaves were collected manually in the morning, presenting water content in 54.16%. The material was transported in thermal packaging to the Laboratory of Research and Application of Essential Oils of the Federal University of Maranhão (UFMA) for screening, determination of water content and drying in a digital greenhouse of convective air FANEM 520.
The collected leaves were analyzed in the laboratory and selected for visual aspects, using only undamaged plant material. Manual cuts were made, with caution in the standardization of the cuts. The cuts were performed transversely in parts of a maximum of 5 cm in length and 2 cm in width.
Chemical profile of B. orellana L.
To identify the constituents, present in the fresh leaf of B. orellana L., we used the gas chromatography technique coupled to Mass Spectrometry (GC-MS) in the Fuel, Catalysis and Environmental Center (NCCA) of the Federal University of Maranhão (UFMA). The identification was also made in the dry leaf and EO constituents that presented the best results regarding antimicrobial and toxicity assays.
The conditions of analysis were as follows: Method: Adams. M; Injected volume: 0.3 µL; Column: Capillary HP-5MS (5% diphenyl, 95% dimethyl polysiloxane) (Equivalent DB-5MS or CP-Sil 8CB LB/MS), in dimensions (30 m x 0.25 mm x 0.25 µm); Drag gas : He (99.9995); 1.0 mL/min; Injector : 280 °C, Split mode (1:10); Oven: 40 °C (5.0 min) up to 240 °C at a rate of 4 °C.min-1, from 240 °C to 300 °C (7.5 min) at a rate of 8 °C.min-1; t = 60.0 min; Detector : EM1; EI (70 eV); Scan mode (0.5 sec/scan); Mass range: 40 - 500 daltons (one); Line transfer: 280 °C; Filament: off 0.0 to 4.0 min; Linear quadrupole mass spectrometer. The AMDIS (Automated Mass spectral Deconvolution Mass & Identification System) program was used to identify the compounds in the sample.
Determination of the initial moisture percentage (%) and drying
The determination of the initial humidity (%) of the leaves of B. orellana L. was obtained through a Quimis moisture scale. For each replicate of the assay, approximately 1g of the plant material with a temperature of105 °C per 10 min was used. As a comparison, the gravimetric method was used, using approximately 2 g of the samples previously weighed on an analytical scale and packed in a drying oven at 105 °C, for 5 h, until it reached constant mass, in three replications according to the ANVISA [17].
The drying of the leaves of B. orellana L. was conducted digital greenhouse of convective air FANEM 520, with standard air velocity in 1 m/s, on alternate days using the temperatures of 35, 45 and 55 °C and the relative humidity (RH) of the ambient air was monitored through a digital thermohygrometer (model INS-28 Intrusul).
A mass of 200 g was used for each drying temperature and about 3g of the samples were placed on plates coated with aluminum of dimensions 90 x 15 mm, and the mass was monitored throughout the process by discontinuous weighing at intervals of 5, 10, 20, 30 and 60 min by means of a Shimadzu AUY220 analytical scale, until the end of the process. The weighings were performed until the mass variations were insignificant. Completed when there was no mass variation in 0.0100 g between five successive weighings. Equation (1) was used to determine the moisture ratio (RU) during the drying of annatto leaves for the different drying temperatures:
Note: RU: moisture ratio, non-dimensional; Ubs: annatto water content, decimal b.s.; Ubsinitial: initial water content, decimal b.s; Ue: equilibrium water content, decimal b.s.
Mathematical modeling
The RU values obtained for each drying air temperature were analyzed through six different empirical and semiempirical equations and nonlinear regression, traditionally used in the description of the drying process of agricultural products [18-21], according to table 1.
Table 1 Mathematical models of nonlinear regression to predict the drying phenomenon of B. orellana leaves.
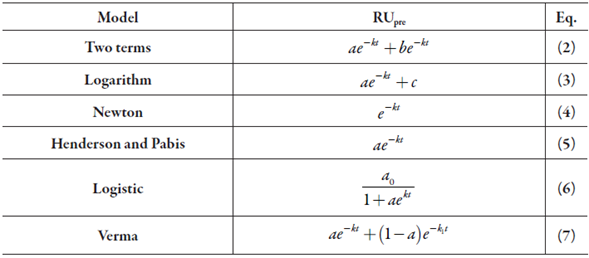
Note: k, k 1 = drying constants (s-1); a, a 0, b, c = coefficients of the models.
For the adjustment of mathematical models, nonlinear regression analysis was performed using the QuasiNewton method and the statistical program Statistica 10.0.
The criteria adopted to determine the best fit of the models to the experimental data were the coefficient of determination (R2) and the mean quadratic deviation (DQM) by equation (8). Subsequently, the residuals and the standard error for each model was analyzed.
Note: RUexp: value observed experimentally; RUpred: value calculated by the model; N: number of experimental observations.
Extraction of Eos
The leaves of B. orellana L. dried in the convective air-drying oven at temperatures of 35 °C, 45 °C and 55 °C were individually crushed in an electric knife mill (Fritsch Pulverisette 14, Oberstein, Germany), and subsequently stored for extraction of their EOs.
For the extractions of the EOs, the hydrodistillation technique was used, with a modified glass Clevenger extractor, coupled to a round bottom balloon of 6000 mL packed in an electric blanket as a heat generating source. The leaves in each extraction routine were packed in the round bottom flask, adding distilled water in a ratio of1:10.
Hydrodistillation was conducted at 100 °C for 3h, collecting the extracted EOs. After extraction, the EO's were dried with sodium anhydrous sulfate (Na2SO4) and stored in amber glass ampoules under 4 °C refrigeration to avoid possible losses of volatile constituents.
The physicochemical parameters of the EO extracted from dry leaves at 35, 45 and 55 °C were analyzed for density, refractive index, alcohol solubility 70% (v/v), color and appearance as described by the Brazilian Pharmacopoeia [22].
Toxicity
This test was performed according to the methodology described by Meyer et al. [23]. In a rectangular container, with a partition containing holes of approximately 0.02 cm thickness spaced by 0.5 cm and evenly distributed, artificial saline solution (60 g L-1 of distilled water) were added (60 g of sea salt/ 1L of distilled water). The container was placed inside an incubator illuminated by a fluorescent lamp, with aeration. On one side of this container, about 64 mg of Artemia salina cysts were added, taking care that they did not cross the partition. The part of the system containing artemia saline cysts was covered with aluminum foil, so that the organisms, at birth, were attracted by light on the other side of the system, forcing them to cross the partition. This procedure aims at homogenizing the physical conditions of the test organisms. Incubation was performed for a period of 48 h. Throughout the test the temperature was monitored.
For the evaluation of the lethality of Artemia salina Leach, a saline solution was prepared stock of each EO in the concentration of 10 000 mg L-1 and 0.02 mg of Tween 80 (active tense). Rates of 5, 50 and 500 µL of this were transferred to test tubes and supplemented with saline solution previously prepared up to 5 mL, obtaining at the end concentrations of 10, 100 and 1000 mg L-1, respectively. All tests were carried out in triplicates, where ten larvae in the nauplium phase were transferred to each of the test tubes. For white control, 5 mL of the saline solution was used for positive control K2Cr2O7 and for negative control 5 mL of a 4 mg L-1 solution of Tween 80. After 24 h of exposure, the count of the live larvae was performed, considering dead those that did not move during observation or with the slight agitation of the vial.
The criterion established by Dolabela et al. [24] was adopted for classification of EOs toxicity, being considered highly toxic when LC50 ≤ 80 mg L-1, moderately toxic to 80 mg L-1 ≤ LC50 ≥ 250 mg L-1 and slightly toxic or nontoxic when LC50 ≥ 250 mg L-1. Statistical analysis of the data for the toxicity test was performed according to the Reed and Muench [25].
Antimicrobial activity
Four strains of bacteria were used: Escherichia coli (ATCC® 25922™) and Staphylococcus aureus (ATCC® 25923™). These were previously identified and confirmed by biochemical tests. Pure microbial cultures maintained in TSA Agar were peaked for brain and Heart Infusion Broth (HIB) and incubated at 35 °C until they reached exponential growth phase (4-6 h). After this period, the cultures had their cell density adjusted in 0.85% sterile saline solution, in order to obtain turbidity comparable to that of the standard McFarland solution 0.5, which results in a microbial suspension containing approximately 1.5x108 CFU mL-1 according to Clinical and Laboratory Standards Institute [26].
The disc diffusion technique was performed according to the Clinical and Laboratory Standards Institute [26], which standardizes the sensitivity tests of antimicrobials by disc-diffusion. First, the plates were prepared with the Mueller Hinton Agar (MHA) culture medium after its solidification was distributed to the microbial suspension on the surface of the agar and left at room temperature for 30 min. Soon after the discs containing 50 µL of EOs and discs with defined concentrations of antibiotics. Using sterile tweezers, the discs were distributed on the surface of the agar. The plates were incubated in a bacteriological greenhouse at 35 °C for 24 h. The diameters of the inhibition halos were measured, including the diameter of the disc. These trials were done in triplicate. The values of the inhibition halos were the mean measurements of the three results. Tests performed in triplicate.
RESULTS AND DISCUSSION
Chemical profile of fresh leaves and drying
Figure 1 shows the chromatogram obtained for the fresh leaves ofB. orellana L. Thirty compounds were identified in the fresh leaf of B. orellana, listed in table 2, of which are predominantly sesquiterpenes and hydrocarbons. The major components were isocaryophillene (26.58%), 1R-α-pinene (19.49%) and β-pinene (12.04%).
Table 2 Chemical constituents identified in the fresh leaf of B. orellana (annatto).
Order | RT (min) | % | Compounds NIST08 | Ret. Index |
---|---|---|---|---|
1 | 3.547 | 19.49 | 1R-α-pinene | 948 |
2 | 5.583 | 12.04 | (-)-β-pinene | 943 |
3 | 8.063 | 0.80 | β-myrcene | 958 |
4 | 8.153 | 0.29 | cyclobutanemethanol | - |
5 | 8.726 | 0.14 | limonene | - |
6 | 9.452 | 0.16 | n-hex-trans-2-enal | - |
7 | 9.784 | 3.35 | trans-ocimene | - |
8 | 9.831 | 1.12 | γ-terpinene | - |
9 | 10.108 | 4.13 | cis-β-ocimene | - |
10 | 11.361 | 0.21 | cis-3-hexenyl acetate | - |
11 | 12.452 | 4.44 | γ-hexenol | - |
12 | 13.932 | 1.08 | copaene | - |
13 | 15.189 | 0.35 | α-bergamotene | - |
14 | 15.297 | 5.39 | caryophyllene | - |
15 | 15.868 | 1.11 | α-guaiene | - |
16 | 16.121 | 0.30 | α-bergamotene | - |
17 | 16.218 | 1.40 | β-garnesene | - |
18 | 16.682 | 1.30 | isoledene | - |
19 | 16.910 | 26.58 | isocaryophillene | - |
20 | 16.959 | 3.85 | elixene | - |
21 | 17.238 | 0.71 | α-himachalene | - |
22 | 17.397 | 4.44 | isocaryophillene | - |
23 | 20.242 | 0.18 | nerolidol | - |
24 | 20.785 | 0.23 | ethyl tetradecyl ester | 2832 |
25 | 21.245 | 0.16 | phenoxethol | 1212 |
26 | 22.524 | 0.13 | di-epi-α-cedrene | 1403 |
27 | 22.779 | 0.32 | dimethyl phthalate ; | 1440 |
28 | 23.582 | 4.50 | palatinol A | 1639 |
29 | 26.100 | 0.98 | pentadecanoic acid | 1869 |
30 | 26.477 | 0.82 | n-hexadecanoic acid | 1968 |
β-caryophillene present in the composition was described as being responsible for the anti-inflammatory, antibacterial, anti-endemic and antifungal action, analgesic and anti-inflammatory β-bisabolen [27]. And the karyophyllene oxide described as acting directly in the inhibition of fungi [28]. The compounds γ-muurolene and β-bisabolene were described as being responsible for microbial inhibition [29].
Figure 2 shows the drying curves of the annatto leaves B. orellana L. at temperatures of 35, 45 and 55 °C, represented by the moisture ratio as a function of time. It is possible to observe the influence of temperature on drying curves, presenting consistent reduction in drying times with temperature increase, providing curves with a typical exponential trend, behavior reported in products [18-21].
This behavior is due to the higher rate of water removal from the product due to the higher energy transfer in the form of heat, caused by the increase in temperature [30]. This is based on the principle that by increasing the temperature the heat transfer to the leaves is increased, so the rate of migration of water from the interior to the surface-environment will be higher. In addition, there is a high correspondence between the data observed experimentally and the data estimated by the model, evidencing that the statistical indexes employed were effective to select the model.
Maximum drying times were around 11am for the temperature of 30 °C, 7 h for the temperature of 45 °C and 2 h for the temperature of 55 °C. Table 3 shows the statistical indices of the adjustments obtained through nonlinear regression using the Statistica 10 software of the mathematical models of Two Terms, Logarithm, Newton, Henderson and Pabis, Logistic to the experimental data of the kinetics of drying of annatto leaves and their respective coefficients of determination (R2) and mean quadratic deviations (MDD). Used in the selection of mathematical models.
Table 3 presents the statistical criteria used to choose the models that best describe the drying process of B. orellana L leaves [31]. All adjusted models presented an adjusted coefficient of determination higher than 99%, except for the temperature of 45 °C where only the Verma model was higher than 0.9900.
Table 3 Adjustment of the analyzed models to the experimental data of the drying kinetics of the leaves of B. orellana L.
Model | 35 °C | 45 °C | 55 °C | |||
---|---|---|---|---|---|---|
R2 | DQM | R2 | DQM | R2 | DQM | |
Two terms | 0.9962 | 0.0335 | 0.9647 | 0.0973 | 0.9943 | 0.0496 |
Logarithm | 0.9963 | 0.0334 | 0.9808 | 0.0808 | 0.9977 | 0.0318 |
Newton | 0.9960 | 0.0346 | 0.9440 | 0.1208 | 0.9936 | 0.0526 |
Henderson and Pabis | 0.9962 | 0.0335 | 0.9647 | 0.0973 | 0.9943 | 0.0496 |
Logistic | 0.9962 | 0.0335 | 0.9647 | 0.0973 | 0.9943 | 0.0496 |
Verma | 0.9965 | 0.0322 | 0.9912 | 0.0472 | 0.9943 | 0.0496 |
Thus, this being a criterion for selecting the models we could infer that the satisfactory models to predict the drying of the species would be the Verma and Logarithm models. As emphasized by Madamba et al. [32] and Almeida et al. [33], in addition to the coefficient of determination (R2) to better predict the most satisfactory model, the NDMD (deviation for estimation) applies. Based on this, it can be seen that for the same models this parameter remained acceptable.
Figure 3 presents examples of waste distribution observed in this work, being a random distribution (a) and a biased (b) for the Verma and Logarithm models for drying at 35 °C, respectively, in the adjustment of b drying data.
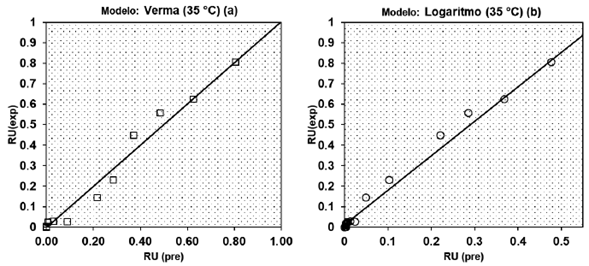
Figure 3 Distribution of residues randomly (a) and a biased (b) for Verma and logarithm models at 35 °C.
In figure 4 and figure 5 show the distribution of residues for the temperatures of 45 and 55 °C, respectively, for both models. The results found are similar to the temperature of 35 °C, with random distribution (a) and a biased (b) for the Verma and logarithm models.
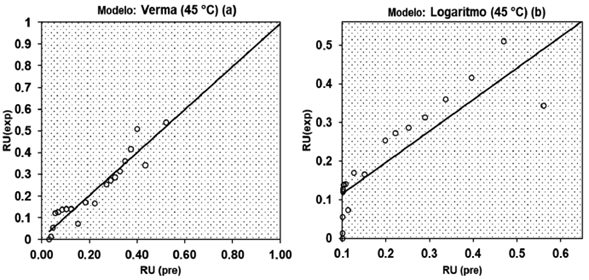
Figure 4 Distribution of residues randomly (a) and a biased (b) for verma and logarithm models at 45 °C.
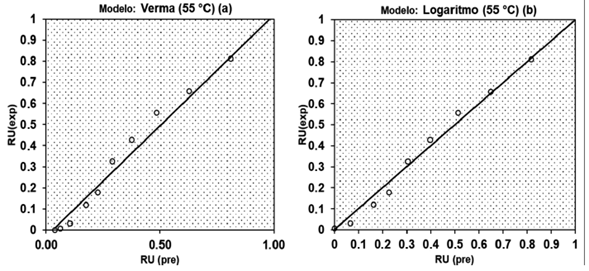
Figure 5 Distribution of residues randomly (a) and a biased (b) for Verma and logarithm models at 55 °C.
The distribution of residuals is a subjective evaluation, but usually a model is considered acceptable if the residual values are in a horizontal zone close to zero, forming random distributions. If the distributions of the residues form geometric figures, present regions in which the model underestimates or overestimates the actual condition or tends to accumulate at a point outside the axis, the distribution of its residues is considered biased and the model is to represent the phenomenon in question [34].
One of the criteria also commonly used for model selection is the standard error of the analysis of the residues and according to Reis et al. [35] values lower than 1 indicate a good fit of the models. Both Verma and Logarithm models were also within this criterion since the parameter values observed in table 4 were relatively low.
The aim was to recommend a model that had the widest possible scope to describe the drying kinetics. Thus, among the recommended models, Verma was selected, since it was the only model with adequate adjustment to describe drying in all proposed conditions. The Verma model is a traditional model, often recommended to describe the drying of agricultural products in works similar to this [34, 36, 37].
According to Coradi et al. [38], the increase in drying air temperature causes the deterioration of the material and consequently alters the integrity of the cell structure. Thus, possibly at a temperature of 45 °C, the cell membrane system remained intact, and was more resistant to water removal, explaining the drying time. Because of this the temperature of 45 °C made it possible to obtain the smallest error for the models tested among this one of Verma, which was the model chosen for the representation. Since the drying process can interfere with the biologically active principle of medicinal plants, greater knowledge about the chemical properties of B. orellana L becomes necessary.
Chemical profile of dry leaves at 45 °C
Figure 6 shows the chromatogram obtained for dry leaves at 45 °C ofB. orellana L.
Twenty compounds were identified in the dry leaf at 45 °C of B. orellana (table 5). The major components were β-bisabolene (42.45%), 1R-a-pinene (11.82%), β-farnesene (9.54%), caryophyllene (9.01%), β-pinene (5.84%) and α-guaiene (5.15%).
Table 5 Chemical constituents identified in EO of dry leaves at 45 °C of B. orellana (annatto).
Order | RT (min) | % | Compounds NIST08 | Ret. Index |
1 | 3.547 | 11.82 | 1R-α-pinene | 948 |
2 | 5.601 | 5.84 | (-)-β-pinene | 943 |
3 | 10.112 | 0.79 | cis-β-ocimene | 976 |
4 | 13.934 | 2.30 | copaene | 1221 |
5 | 14.574 | 0.24 | isoledene | 1419 |
6 | 15.191 | 1.28 | bergamotene | 1425 |
7 | 15.299 | 9.01 | caryophyllene | 1494 |
8 | 15.871 | 5.15 | α-guaiene | 1490 |
9 | 16.121 | 1.36 | β-cedrene | 1398 |
10 | 16.218 | 3.85 | (Z)-β-Farnesene | 1440 |
11 | 16.386 | 0.48 | β-farnesene | 1440 |
12 | 16.682 | 1.78 | isoledene | 1419 |
13 | 16.782 | 0.29 | valencene | 1474 |
14 | 16.927 | 42.45 | β-Bisabolene | 1500 |
15 | 17.241 | 1.28 | α-patchoulene | 1403 |
16 | 17.399 | 9.54 | β-farnesene | 1440 |
17 | 20.239 | 0.88 | trans-nerolidol | 1564 |
18 | 21.056 | 0.25 | spathulenol | 1536 |
19 | 22.521 | 0.21 | α-bergamotene | 1430 |
20 | 23.580 | 1.20 | palatinol A | 1639 |
Therefore, when comparing these results to the studies by Amorim et al. [39] and Viuda-Martons et al. [40] on the composition of the EO of B. orellana L. leaves, differences were found in relation to the chemical substances present and their concentrations. Rather et al. [41] also studied the chemical characterization of B. orellana L. seeds and found twenty-four substances, among them, the ones with the highest concentrations were: ishwarane (18.6), β-farnesene (10.52%) and caryophyllene (8.01%). These variations of substances and their quantities can be attributed mainly to environmental conditions, plant age and harvesting methods. Possibly the habitat of the plant contributed to the different substances and their concentrations, since the climate and soil type [42].
Due to the drying conditions of 45 °C temperature, some substances showed variation in their concentration, such as 1R-α-pinene oxide and β-pinene (5.84%) that showed an increase in the mean concentration with the increase in drying temperature, isocaryophyllene was not found with the drying of the leaf at 45 °C, possibly, with the increase in temperature and speed of the drying air, the volatilization of some substances, causing their concentrations to be reduced, evidencing the thermosensitivity of these substances. It is believed that the stress caused by drying induces an increase in the terpene content in dry plants, compared to the fresh plant [43].
Physicochemical parameters of Eos
After drying at established temperatures, The EO's were extracted and their physicochemical properties were determined. The physicochemical parameters of EOs are important not only for quality determination, but also for the control of their purity. The results are presented in table 6.
Table 6 Physicochemical EO parameters of B. orellana L. leaves (annatto).
EO | Density | Refractive index | Color | Yield |
EO 35°C | 0.9998 (g mL-1) | 1.4200 (n D 25 °C) | Yellow | 0.21 % |
EO 45°C | 1.0779 (g mL-1) | 1.5300 (nD 25 °C) | Yellow | 2.23 % |
EO 55°C | 1.0500 (g mL-1) | 1.4900 (nD 25 °C) | Yellow | 0.38 % |
A small change in density and refractive index was observed, with the increase in temperature. Unlike the other parameters, the color did not present visual differentiation of the extracted EOs. The drying temperature mainly influenced the yield of the applied process, and a yield of 2.23% was observed for the EO obtained from the dry leaves at 45 °C, being an extremely significant increase. Martins et al. [44] reports that temperatures above 45 °C damage plant organs and their contents, as they cause a "cooking" of plants and not a drying. Thus, reflecting that even with the temperature of 55 °C processing the product faster, part of it is quantitatively lost.
EO's are heat-sensitive substances, so increasing the temperature of the drying air can volatilize these compounds, resulting in lower extractive yield [45, 46]. In relation to the yield of the EO obtained from the leaves dried at 45 °C, according to what was also reported by Souza et al. [30] the grinding facilitated the extraction, because it exposed the structures that contained the EO to the process and increased the contact surface of the leaves with the water used hydrodistillation. Giwa-Ajeniya et al. [47] found similar results when they evaluated the yield of EO through hydrodistillation ofB. orellana leaves in the Alimosho area, Lagos State, Nigeria.
Schimitbertger et al. [48], Ahmed et al. [46], Memarrzadeh et al. [49] and Sefidkon et al. [50] made a visual evaluation of the staining of EOs obtained in their studies. Regarding the color, it was visually verified that the color of the extracted EO presented pale yellowish coloration. This difference in color was justified by differences in chemical composition. Comparing the values for the EO studied with those of the literature, it can be observed that there was a similarity in the temperature range 35-55 °C, with regard to the parameters analyzed. The small differences in the values found can be attributed to factors such as collection time, different soil types, conditions and storage time.
Antimicrobial activity of EO's
The Disc Diffusion Method was evaluated for each of the EOs obtained their bactericidal potentials. The result of this assay is visualized by an inhibition halo formed by the ability of EO to inhibit microbial growth. The diameters of the inhibition halos for the action of The EO's against the bacteria are presented in table 7.
Table 7 Diameter of inhibition halos (mm) for EO action against the tested microorganisms.
Microorganisms | GEN (30 µg) | EO 35°C | EO 45 °C | EO 55 °C |
E. coli (ATCC 25922) | 25 mm | NI | 12 mm | NI |
S. aureus (ATCC 25923) | 27 mm | 7 mm | 21 mm | NI |
Note: *NI: there was no inhibition of the microorganism by the action of the EO tested.
According to Moreira et al. [51], bacteria are considered sensitive to the action of EO's when they present inhibition halos greater than 9 mm in view of the action of these natural products. From this classification, it was possible to verify that E. coli was sensitive only to the action of EO extracted by drying B. orellana L. at 45 °C. For S. aureus only the EO of 55 °C showed no bactericidal activity and the temperature of 35 °C was not sensitive.
The results observed in the disc diffusion test presented in table 7 show that EO obtained by drying B. orellana L. at 45 °C was more efficient in inhibiting S. aureus bacteria, revealing a 21 mm inhibition halo, comparing to EO obtained using the temperature of 35 °C where it inhibited the same bacterium by only 7 mm, while the EO obtained from the dry plant at 55 °C did not present bactericidal biological potential. Therefore, the bactericidal potential was definitive in the choice of drying temperature.
For the Bacterium E. coli, the drying temperature again influenced the biological potential of the EO. The EO obtained from the dry leaves at 45 °C presented the best bactericidal performance with a halo of 12 mm and again the EO of 55 °C did not present bactericidal potential as well as the EO of 35 °C.
Studies have already reported the inhibitory action of annatto on S. aureus. Gonçalves et al. [52] performed tests with commercial annatto seed extract on Gram-positive bacteria including S. aureus and proved that it presented antimicrobial activity inhibition halos of 15 mm. In another research done by Silva et al. [53] confirmed antimicrobial activity of mature seed extract on the bacterium S. aureus and E. coli with halo of 18 mm and 12 mm in diameter, respectively.
Several authors have analyzed the bactericidal activity of leaf EO for the bacteria S. aureus and E. coli. Enciso-Díaz et al. [54] using the extract of Eucalyptus globulus (eucalyptus), observed that the inhibition halos were similar to that of annatto at 45 °C, being 22.5 mm for S. aureus and 8 mm for E. coli. Moreover, with the aqueous extract of Eucalyptus globulus González-Burgosa et al. [55] reported that there was no longer inhibition for E. coli, having only inhibition in the growth of S. aureus with a halo of inhibition of less than 15 mm. The potential for inhibition in annatto is justified by Majolo et al. [56], which explains that the potential of the plant is due to the presence of artemisin (sesquiterpene), known for its anti-malarial properties, which have the ability to complex proteins and bacterial cell wall, causing its lysis.
Toxicity of Eos
The results obtained in the toxicity assay of The EO's are presented in table 8, respectively, where it is possible to observe that the increase in lethal concentration increases with increasing temperature. At all temperatures, the EO of B. orellana leaves showed nontoxic.
Table 8 LC50 for EOs action against Artemia salina.
EO | LC50 | Classification |
EO 35 °C | 261.11 mg L-1 ± 5.10 | Nontoxic |
EO 45 °C | 280.25 mg L-1 ± 2.30 | Nontoxic |
EO 55 °C | 315.44 mg L-1 ± 4.44 | Nontoxic |
The in vitro toxicity assay against Artemia salina is a fast and low-cost bioassay and has been one of the most used tools for preliminary toxicity assessment and can be used in the screening of new antispasmodic drugs and antimalarials [57, 58]. Toxicity is classified from obtaining LC50 (Lethal Concentration 50%) for the action of The EO against larvae of Artemia salina Leach. A LC50. Many studies have shown that the toxicity of a compound against Artemia salina correlates with cytotoxic activity against human tumors, McLaughlin et al. [59] reported that this bioassay led to the discovery of a new class of agents active antitumor (Annonaceous acetogenins), and is also related to activity against Trypanosoma cruzi, a protozoan causing chagas disease [60, 61], with viruscidal, antifungal and antimicrobial activity [62].
In a study conducted by Kumar et al. [63] when using extracts from the leaves of B. orellana showed a non-toxic effect against Artemia salina, demonstrating that extracts from other parts of the same plant may contain different active ingredients, which can toxically effect against the same tested species. Vilar et al. [64] found that the roots showed negative results for toxicity for Artemia salina.
Chemical EO profile of dry leaves at 45 °C
Figure 7 shows the chromatogram obtained for EO from the leaves of B. orellana L at 45 °C.
Twenty compounds were identified in the EO of the dry leaf at 45 °C, listed in table 9, of which are predominantly sesquiterpenes and hydrocarbons. The major components were 1R-α-pinene (26.5%), β-bisabolene (19.71%), caryophyllene (10.42%) and β -pinene (9.23%).
Table 9 Chemical constituents identified in EO of dry leaves at 45 °C.
Order | RT(min) | % | Compounds NIST08 | Ret. Index |
---|---|---|---|---|
1 | 3.569 | 26.50 | 1R-α-pinene | 948 |
2 | 5.777 | 9.23 | (-)-β-pinene | 943 |
3 | 9.431 | 2.55 | 2-trans-hexenal | 814 |
4 | 10.141 | 1.55 | β-cis-ocimene | 976 |
5 | 13.670 | 2.83 | α-chamigrene | 1512 |
6 | 13.944 | 4.28 | copaene | 1221 |
7 | 15.197 | 0.60 | α-bergamotene | 1430 |
8 | 15.260 | 1.20 | β-elemene | 1398 |
9 | 15.309 | 10.42 | caryophyllene | 1494 |
10 | 15.443 | 0.61 | allo -Aromadendrene | 1386 |
11 | 15.876 | 3.28 | γ-gurjunene | 1461 |
12 | 16.127 | 0.59 | β-Cedrene | 1398 |
13 | 16.207 | 4.05 | α- Caryophyllene | 1579 |
14 | 16.686 | 2.92 | isoledene | 1419 |
15 | 16.923 | 19.71 | β-bisabolene | 1500 |
16 | 16.965 | 3.95 | α-chamigrene | 1512 |
17 | 17.238 | 0.93 | δ-cadinene | 1469 |
18 | 17.399 | 3.26 | β-cedrene | 1398 |
19 | 20.241 | 0.86 | Nerolidol | 1564 |
20 | 21.058 | 0.68 | Spathulenol | 1536 |
Pino and Correa [65] analyzed the chemical constitution of EO from annatto seeds by GC/MS, 73 compounds were detected, of which 65 compounds (80.1%) were identified, ishwarane was the main component, constituting 18.6% of the composition of The EO, followed by geranylgeraniol (9.1%), bicyclogermacrene (8.4%), germacrene D (6.9%) and δ-selinene (6.2%).
Discordant results were found by Giwa-Ajeniya et al. [47], who verified in EO samples of B. orellana leaves, from Nigeria, higher α-guaiene contents (49.3%), together with guaiol (8.1%), valenceno (7.7%) and β-elemeno (5.9%). As well as Silva et al. [66] under study with the EO of the roots. The major constituents were α-guaieno (25.0%), espathulenol (12.0%) and β-cayophyllene (9.7%). However, several monoterpenes were found in the annatto extracts of the seeds, with a higher amount of monoterpene hydrocarbons (45.0%). Among the major constituents α-pinene (29.1%), β-pinene (14.5%), germacreno D (10.0%) and espatulenol (9.0%) compounds [67].
It is noteworthy that ishwarane, one of the main compounds of leaf oils and seeds of B. orellana was not present in the study sample. This can be explained by environmental factors such as location of the species and climate of the region. In the literature there are still few studies published, with the need for further studies of the EO of the leaves of B. orellana L.
Through the results obtained, it is possible to conclude that the increase in drying temperature promoted a reduction in dehydration times. The Verma and logarithm models presented the highest coefficients of determination (R2 > 0.98) and the lowest mean quadratic deviations (DQM < 0.10), however, the Verma model was the only one with random distribution of the residues of the studied temperatures. The drying temperature at 45 °C is the most indicated for the species Bixa orellana L., in this study. Since, at this temperature, it presented the best yield, the best bactericidal activity of the essential oil obtained, thus encouraging its application potential.