INTRODUCTION
Mycobacterium tuberculosis (Mtb) is the main causative agent of human tuberculosis (TB), a disease that remains a serious public health problem, chiefly in low- to middle-income countries, and accounts for high mortality rates around the world. It is estimated that about one-quarter of the world's population is latently infected with Mtb, as residual mycobacteria may enter a dormant stage; such that ~ 10% of these individuals stand a risk of developing TB disease through a reactivation later in life, at least in part, due to possible immunodeficiency disorder [1-3]. In 2018, with 10 million new cases and ~1.2 million deaths at the global level, TB remained one of the top 12 world's deadliest diseases; and, before the current pandemic caused by the severe acute respiratory syndrome coronavirus-2 (SARS-CoV-2), this disease was the leading cause of deaths from a single infectious agent. In Brazil, a country with -211 million inhabitants, the estimates for TB published by the World Health Organization (WHO) in 2018 pointed out an incidence rate of 45 per 100 000 population (totalizing 95 000 new cases) and a mortality rate of -3 per 100 000 population. Moreover, a considerable burden of disability-adjusted life-years (DALYs) lost due to TB in Brazil has been observed, achieving a high total number of DALYs/100 000 population over the past 30 years [1, 2].
Currently, TB lacks new more powerful and safe antitubercular therapy (ATT), which is further aggravated for multi and extensively drug-resistant TB (MDR/XDR-TB). Furthermore, most ATT regimens currently recommended require patients to take at least four anti-TB drugs for a long time (for example, the four first-line drugs: isoniazid [INH], rifampicin, ethambutol and pyrazinamide), with serious side effects associated and levels of toxicity [1]. Of these, the controversial role of oxidative damage arising from drug metabolism/action and/or TB disease, with the participation of the immune system, frequently imposes the need for antioxidant-based therapy associated with ATT [4-7].
The innate immune response following Mtb infection plays a crucial role in preventing the onset of active TB and limiting its spread. In addition to routine functions including phagocytosis and degranulation, the phagocytes-derived reactive oxygen/nitrogen species (ROS/RNS) during activation of the oxidative burst can elevate the immune system at a most effective response against different strains of Mtb and other microorganisms [3, 8-11]. Among the phagocytes that combat Mtb, polymorphonuclear neutrophils (PMNs) are the immune system's key players, with an estimated lifespan of 5-6 days in the circulation, or much longer when exposed to anti-apoptotic cytokines or when these cells are in tissue [10, 12-14].
Neutrophils are one of the first host cell types to encounter Mtb in the lungs and during the acute phase of mycobacterial infection [14 ], they generate superoxides (O2•-) via the nicotinamide adenine dinucleotide phosphate (NADPH) oxidase complex, also referred to as neutrophil oxidase isoform 2 (Nox2; or gp91phox, 91-kDa glycoprotein ofphagocyte oxidase). Besides being direct active antimicrobials that can boost the killing of Mtb, ROS/RNS can activate and/or regulate other antimycobacterial mechanisms, such as the release ofproteolytic peptides/enzymes into the phagosomal space. Moreover, as recently discovered [15], the formation of extracellular traps (ETs, in neutrophils so-called neutrophil extracellular trap [NET]) can be a downstream event of Nox2 activation; thus, synergizing in the clearance of mycobacteria [14, 16-23]. Upon activation of Nox2 by Mtb stimulus, neutrophils release O2•-, allowing the formation of other oxidants, including hydrogen peroxide (H2O2), hydroxyl radical (HO•), hypochlorous acid (HOCl), and singlet oxygen (1O2). Meanwhile, concomitant activation of nitric oxide synthase (NOS) generates nitric oxide (NO). NO avidly reacts with O2•- to form peroxynitrite (ONOO-). Peroxynitrite is a potent antimicrobial agent [3, 16, 19].
The double-edged sword of high ROS levels from an exacerbated immune response in active TB and/or drug metabolism (during TB treatment), without concomitant antioxidant defense (in the so-called oxidative stress, OS), imposes to host status of oxidative tissue damage and aggravates lung diseases, as seen in TB, respiratory syncytial virus (RSV) induced acute bronchiolitis, and coronavirus disease 2019 (COVID-19) ; as well as in non-communicable diseases such as chronic obstructive pulmonary disease and cystic fibrosis [13, 14, 24-30]. These factors make antioxidant use a rational approach along with ATT. On the other hand, either the use of antioxidants supplementations in an indiscriminate manner, currently very common among the population, or the use of these supplementations as adjuvants during ATT could impair the immune response against Mtb (via modulation of the redox state) and potentially increase the host's susceptibility to Mtb/TB [31, 32].
Over the last few decades, nitroxide stable radicals have widely been investigated due to their potent antioxidant properties. These compounds can be reduced to hydroxylamines or oxidized to oxoammonium cations and react with various oxidants generated in the human body in a continuous recycle manner [33]. Cyclic piperidine nitroxide 4-hydroxy-2,2,6,6-tetramethylpi-peridine-N-oxyl (Tempol, 4-hydroxy-Tempo, or 4-OH-TPO) is an antioxidant with superoxide dismutase (SOD) mimetic properties (at lower concentrations) and low molecular weight. Tempol easily permeates biological membranes and acts as an intracellular scavenger of O2 •- and other ROS. Innumerous bioactivities are attributed to this nitroxide [34-39].
Therefore, this study investigated the effects of Tempol on the formation of ROS by human neutrophils and the associated mycobactericidal response of these cells. Moreover, a possible cytotoxic effect of Tempol on neutrophils was ruled out. We also demonstrated how this nitroxide can influence the intracellular killing of Mtb by neutrophils, but not the phagocytosis.
MATERIALS AND METHODS
Chemicals and reagents
The following reagents were purchased from Sigma-Aldrich, St. Louis, MO, USA: Leish-man's stain, Bovine serum albumin (BSA), Cytochrome c (from the equine heart), Phor-bol 12-myristate 13-acetate (PMA), N-formyl-methionyl-leucyl-phenylalanine (fMLP), 4-hydroxy-2,2,6,6-tetramethylpi-peridine-N-oxyl (Tempol, 4-hydroxy-Tempo, or 4-OHTPO, 97%), Thapsigargin [≥98% high-performance liquid chromatography (HPLC)], Diphenyleneiodonium chloride (DPI), horseradish peroxidase (HRP), 3-Aminophth-alhydrazide (Luminol 97%), 4-Aminophthalhydrazide (Isoluminol 97%), superoxide dismutase (SOD, from bovine erythrocytes), Dextran from Leuconostoc spp. 3-(4,5-dimeth-ylthiazol-2-yl)-2,5-diphenyltetrazolium bromide (MTT) was purchased from USB Corp. (Cleveland, OH), D-glucose from Vetec fina, Triton-X-100 1 % from Invitrogen, Dimethyl sulfoxide (DMSO) is from Neon Comercial and Ficoll-Paque Plus from GE Healthcare. All solvents were of HPLC grade from Pharmco (Brookfield, CT), unless otherwise indicated. All reagents and materials were sterilised in an autoclave (whenever possible) or by filtration (Millipore Corporation, hydrophilic Durapore® PVDF, 0.22 μm, 47 mm). Tempol was diluted in deionized water (purified through a Milli-Q system). Phosphate-buffered saline (PBS, pH 7.4): NaCl (120 mM), KH2PO4 (1.7 mM), Na2HPO4 (8.3 mM) e KCl (5 mM). Krebs-Ringer phosphate buffer (KRG): PBS plus CaCl2 (1mM), MgCl2 (1.5 mM), and glucose (1 mM). pH 11 water (H2O) was prepared by adding NaOH (1 mM) in H2O. Agar/broth Middlebrook (7H10/7H9, HiMedia Laboratories); 10% oleic acid-albumin-dextrose-catalase (OADC : 2.50 g bovine albumin V 1.00 g dextrose, 0.002 g catalase, 0.025 g oleic acid, 0.425 g NaCl, and 50.00 mL deionized water; HiMedia Laboratories).
Ethics statement
This study was performed in compliance with the relevant laws and institutional guidelines in accordance with the ethical standards of the Declaration of Helsinki (http://www.wma.net/en/30publications/10policies/b3/index.html). Human blood was collected in accordance with a University Federal of Alfenas Institutional Review Board-approved protocol #292274. The volunteers gave their written informed consent for the use of their blood.
Mycobacterium tuberculosis culture and stimulus preparation
Attenuated H37Ra strain of Mycobacterium tuberculosis was obtained from the American Type Culture Collection (ATCC 35177, Manassas, VA) and maintained in medium Löwenstein Jensen. Mycobacterium tuberculosis was grown in Middlebrook 7H9 liquid medium supplemented with 10% oleic acid-albumin-dextrose-catalase (OADC, plus 0.05% tween 80, 0.5% peptone, and 0.5% glycerol) for 21 days. After that, the pellet obtained by centrifugation (3000xg, 7 min) was resuspended in PBS and then adjusted at 3 x 108 colony forming units (CFU) of mycobacteria/ml and further diluted. Finally, non-heat-inactivated (viable) or heat-inactivated (60 min, 80 °C) Mtb was opsonized or not, with autologous human serum (20%) and incubation at 37 °C for one hour, followed by centrifugation (3000xg, 10 min), and the pellet resuspended in PBS and stored at -70 °C, or immediately used.
Isolation of Human neutrophils
Peripheral blood samples were harvested by venipuncture using lithium heparin-con-taining vacutainer tubes (BD Biosciences, San Jose, CA) from healthy volunteers, aged 18 to 40 years, and neither volunteer suffered from an acute infection or inflammation or was on anti-inflammatory or antibiotic use, at the time blood was drawn. Neutrophils were isolated by Ficoll-Paque density-gradient centrifugation [40]. Cells were purified from venous whole blood (1:1 in PBS) using Ficoll-Paque for initial separation (2000xg 15 min), followed by dextran (4%) sedimentation of the pale-red granulocyte layer. The contaminating erythrocytes present in the supernatant were further removed by hypotonic lysis in ice-cold H2O (with a prompt restoration of tonicity by adding an equal volume of 2.7% NaCl) and sequential centrifugation (600xg, 5 min), yielding a >94% pure neutrophil pellet of >90% viability, as attested by Leish-man staining and trypan blue dye exclusion (viable cells counted in an AO Spencer hemocytometer chamber). The isolated neutrophils were resuspended in KRG and immediately used. Optionally, neutrophils were opsonized with autologous human serum (20%) by incubation at 37 °C for one hour, followed by centrifugation (600xg, 5 min), and the pellet resuspended in KRG.
NADPH oxidase (Nox2) activity
Measurement of extracellular superoxide generation by superoxide dismutase (SOD)-inhibitable cytochrome C reduction assay
Superoxide (O2 •-) levels generated during the oxidative burst of neutrophils were measured using the membrane-impermeable substrate cytochrome C (cytC), which detects extracellular O2 •-. The tests were performed as previously reported [41], via a discontinuous system of the superoxide dismutase (SOD)-inhibitable cytC reduction assay. Neutrophils (1x106 cells/ml) in KRG were seeded into a flat-bottomed 1% BSA-coated 96-well plate and allowed to settle at 37 °C for 30 min, following or not Tempol (450 μM) exposure 15 min prior to stimulation. In the negative controls, previous treatment with 50 U/ml SOD (scavenger of O2 •-) or 32 μM DPI (blocker of NADPH oxidase) 15 min prior to stimulation, were performed. Next, opsonized, or non-opsonized Mtb stimulus (multiplicity of infection [MO I] from 1 to 100) and 25 μM cytC were added. In the positive control groups, neutrophils triggered with 40 μM fMLP or 100 nM PMA or 4 μM thapsigargin were included. Three hours after incubation at 37 °C, centrifugation was performed (3000xg, 10 min) and changes in optical density (OD) on both samples and their reference supernatants were spectrophotometrically registered at a wavelength of 550 nm (OD550), using a Biochrom Anthos Zenyth 200 Microplate Reader. The amount of O2 •- was calculated obeying a one-to-one molar stoi chiometry between O2 •- produced and cytC molecules reduced (s = 21.1 mM-1-cm-1). The results were expressed in nmoles of O2•- per 1x106 cells for 3 hours, as follows:
AOD550 = OD550 (sample) - OD550 (reference [SOD])
AOD550x12.64 = nmoles O2 •- /1x106 cells/3 hours
Quantification of total and extracellular ROS through the luminol and isoluminol amplified chemiluminescence assay.
Neutrophils in KRG (1x106 cells/ml) were pretreated or not with 450 Tempol or 32 μM DPI (control) for 15 min. Next, the stimulus was added: heat-inactivated and non-opsonized Mtb (MOI 10) or 40 fMLP, followed by addition of 50 luminol (to evaluate total ROS, intracellular and extracellular, including O2 •-, H2O2, HO•, HOCl, and 1O2) or 50 μM isoluminol (to evaluate extracellular ROS) plus 8 U/ ml horseradish peroxidase. The chemiluminescence signal was monitored over 60 min, using a Glomax 20/20 Luminometer (Promega, USA) and the results were expressed as relative light units (RLU) [41].
Analysis of neutrophil mycobactericidal activity
Using the two-step protocol proposed by Green et al. [42], neutrophils (1x106 cells) in KRG pretreated or not with Tempol (450 μM) were incubated with non-opsonized and non-heat-inactivated (live) Mtb (1x107 CFU/ml, MOI 10), for different times (10, 30, and 90 min) at 37 °C, under occasional agitation. At each one of these intervals, samples were taken, and treated with ice-cold PBS and then centrifuged (100xg, 8 min). Next, either the supernatant (which contains the extracellular mycobacteria that have not been phagocytosed) or the pellet (which contains the intracellular mycobacteria that have been phagocytosed) was diluted in pH 11 H2O, and after serial dilutions, dispensed on agar Middlebrook 7H10 supplemented with 10% OADC (plus 0.05% tween 80, 0.5% peptone, and 0.5% glycerol). Finally, the Petri plates were incubated at 37 °C in a humidified candle extinction jar with 5% CO2, for 21 days. Neutrophils pretreated with DPI (32 μM) were used as a control. After 21 days, Mtb viability was measured from the number of colonies (viable extracellular or intracellular mycobacteria) grown on agar, and the result was expressed in CFU/ml.
Assessment of cytotoxic effects
The cell respiration in human neutrophils was evaluated using the 3-(4,5-dimethylthi-azol-2-yl)-2,5-diphenyltetrazolium bromide (MTT) reduction assay that measures the mitochondrial-dependent reduction of MTT to formazan [43]. Neutrophils (1x106 cells/ml) in KRG in the presence or absence of Tempol were seeded into a flat-bot tomed 1% BSA-coated 96-well plate and allowed to settle at 37 °C for 30 min. Next, the plate was incubated at 37 °C for 4 hours in an atmosphere containing 5% CO2. Post incubation, 40 μL of MTT was added to the wells, and the plate was again incubated for 4 hours. Finally, dimethyl sulfoxide was added per well to solubilize the formazan and, after centrifugation, the supernatant OD570 was determined (Biochrom Anthos Zenyth 200 Microplate Reader). After 4hr of exposure, a percentage of relative survival was calculated (neutrophils 100% viability, control) and Triton-X-100 was used as a 100% lysis control (0% cell viability).
Data analysis
The data [mean values ± standard error of the means (SEM)] are representative of three independent experiments, at least in triplicate. Statistical comparisons were made via one-way analysis of variance (Anova) with posthoc pairwise multiple comparisons made by the Tukey's test (α= 5%; significant whenp < 0.05). To calculate the phagocytosis (Kp) and Killing (Kk) rate constants, data from the two-step protocol were analyzed using the "Microsoft Office Excel 97-2003" (available at: <http://www.otago.ac.nz>) [42].
extracellular O2 •- levels (figure 1). Positive controls PMA or fMLP-triggered oxidative burst augmented ROS generation, whereas previous DPI (negative control) treatment decreased O2•- levels, by directly inhibiting the Nox2 system causing a shunt of the electron flux (figure 1A). The induction of ROS in Mtb-stimulated neutrophils was dependent on the MOI (figure 1B), with an outstanding increase in ROS levels when the MOI rose from 1 to 10, but with no statistical difference at MOIs above 10. Even when Mtb was opsonized and neutrophils co-stimulated with thapsigargin, Tempol decreased O2 •- levels (figure 1C). As checked using luminol (total intracellular and extracellular ROS, figure 2A) and isoluminol (extracellular ROS, figure 2B), Tempol decreased ROS levels in Mtb-stimulated neutrophils.
RESULTS
Nitroxide Tempol decreases ROS levels during the neutrophil oxidative burst
The levels of ROS in Mtb-stimulated neutrophils were diminished by Tempol treatment (p < 0.05, figures 1 and 2). Specifically, Tempol down-regulated Mtb-induced extracellular O2•- levels (figure 1). Positive controls PMA or fMLP-triggered oxidative burst augmented ROS generation, whereas previous DPI (negative control) treatment decreased O2•- levels, by directly inhibiting the Nox2 system causing a shunt of the electron flux (figure 1A). The induction of ROS in Mtb-stimulated neutrophils was dependent on the MOI (figure 1B), with an outstanding increase in ROS levels when the MOI rose from 1 to 10, but with no statistical difference at MOIs above 10.
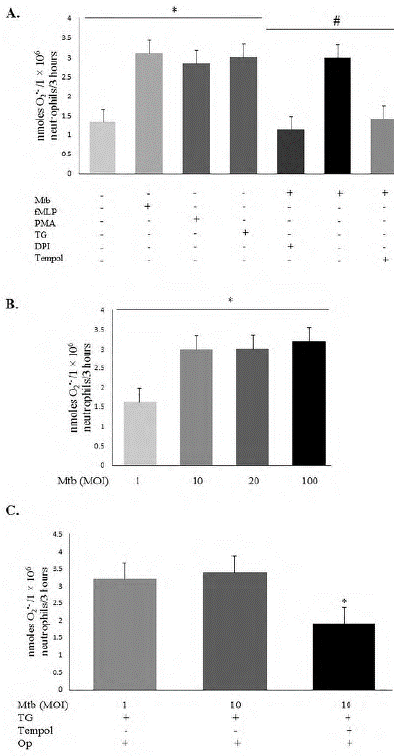
Figure 1 Amounts of extracellular superoxide (O2 •-) in the different conditions. Mtb: Mycobacterium tuberculosis (H37Ra strain); TG: thapsigargin (4 μM); fMLP: N-formyl-methionyl-leucylphenylalanine (40 μM); PMA: phorbol 12-myristate 13-acetate (100 nM); Op: opsonized Mtb; DPI: diphenyleneiodonium chloride (32 μM). A. Tempol (450 μM) significantly decreased O2 •-levels (nmoles per 106 neutrophils/3 hours) generated upon Mtb as a stimulus. TG- or PMA- or fMLP- stimulated neutrophils are positive controls (activators of oxidative burst), and DPI is negative control (inhibitor of the NADPH oxidase complex). *p < 0.05: TG- or PMA- or fMLP-stimulated neutrophils compared with the neutrophils without any stimulation; #p < 0.05: DPI- or Tempol-treated Mtb- stimulated neutrophils compared with the Mtb-stimulated neutrophils. B. Activation of Nox2 activity to release O2 •- was dependent on the MOI. *p < 0.05. C. Neutrophil response to opsonized Mtb. Even on the influence of opsonization and TG, Tempol (450 μM) significantly decreased the amount of O2 •-. *p < 0.05.
Even when Mtb was opsonized and neutrophils co-stimulated with thapsigargin, Tempol decreased O2•- levels (figure 1C). As checked using luminol (total intracellular and extracellular ROS, figure 2A) and isoluminol (extracellular ROS, figure 2B), Tempol decreased ROS levels in Mtb-stimulated neutrophils.
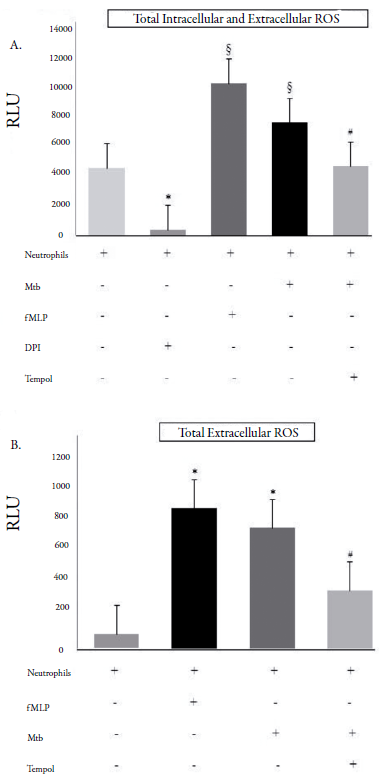
Figure 2 Amounts of total (intra and extracellular) and total extracellular reactive oxygen species (ROS). RLU: relative light units (60 min); Mtb: Mycobacterium tuberculosis (H37Ra strain); fMLP: N-formyl-methionyl-leucyl-phenylalanine (40 μM); DPI: diphenyleneiodonium chloride (32 μM). A. Total intra and extracellular ROS were decreased by Tempol (450 μM), when neutrophils were stimulated with Mtb (MOI 10). fMLP and DPI are control groups. *p < 0.05: Neutrophils without any stimulation compared with the DPI-treated neutrophils; §p < 0.05: fMLP- or Mtb-stimulated neutrophils compared with the neutrophils without any stimulation; #p < 0.05: Tempol-treated Mtb-stimulated neutrophils compared with the fMLP- or Mtb-stimulated neutrophils. B. Total extracellular ROS was decreased by Tempol (450 μM). fMLP is a control group. *p < 0.05: fMLP- or Mtb-stimulated neutrophils compared with the neutrophils without any stimulation; #p < 0.05: Tempol-treated Mtb-stimulated neutrophils compared with the fMLP- or Mtb-stimulated neutrophils.
Tempol impairs the mycobactericidal activity of neutrophils by altering the intracellular redox tune and ROS-mediated intracellular killing of Mtb
Tempol impaired the anti-Mtb response by shifting the ROS/RNS balance. First, we demonstrated that Tempol decreased the total microbicidal activity of neutrophils against Mtb, as CFU numbers of the mycobacteria were significantly higher as the untreated group (p < 0.05, figure 3A). Moreover, Tempol decreased the rate constant for killing (Kk) promoted by neutrophils, but it did not affect their rate constant for phagocytosis (Kp) (figure 3B). These results demonstrate the putative decrease in ROS levels, caused by Tempol, affecting the intracellular killing of Mtb. In the control group, DPI-treated neutrophils had the same mycobactericidal capability as Tempol-treated neutrophils (p > 0.05), including the effect on Kk•
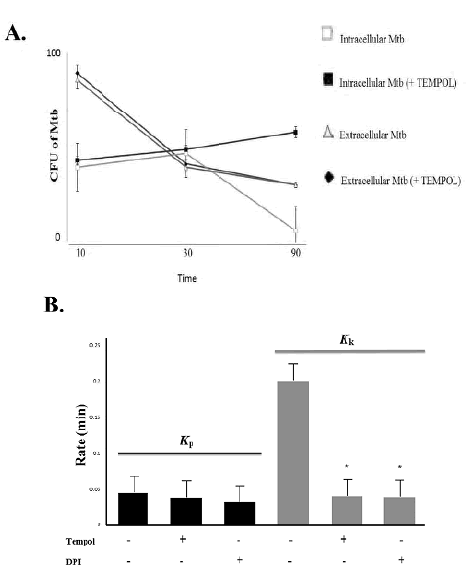
Figure 3 Phagocytosis and microbicidal response of neutrophils against Mycobacterium tuberculosis. Mtb: Mycobacterium tuberculosis (H37Ra strain); CFU: colony-forming units; DPI: diphenyleneiodo-nium chloride; *p < 0.05. A. After incubation of Mtb with neutrophils at an Mtb:-neutrophil ratio of 10:1 (MOI 10), the CFU of extracellular Mtb decreased over time (from 10 to 90 min, white triangles), due to a continuous phagocytosis of the mycobacteria. Paradoxically, within the first 30 min, the intracellular burden of Mtb (White Square at 30 min) increased, since at that moment neutrophils promote outstanding phagocytosis, but not promote yet, the efficient killing of the mycobacteria. From 30 min, increased intracellular killing is observed (decreasing CFU of Mtb, White Squares). At 90 min of incubation, the most pronounced decrease in CFU of intracellular Mtb was observed. In another set of experiments, Tempol (450 μM) exhibited a significant effect on the intracellular killing of Mtb (p < 0.05), increasing the CFU of Mtb over time (notoriously at 90 min, Filled Squares), as compared with the untreated groups (White Squares). Tempol did not interfere with phagocytosis, since the CFU of extracellular Mtb were quite similar between untreated and Tempol-treated Mtb-infected neutrophils (white triangles and filled lozenges,p > 0.05). B. Rate constants for phagocytosis (K p ) and Killing (Kk) in the presence and absence of Tempol. There was no significant difference between the Kp of untreated and Tempol (450 μM) or DPI (32 μM)-treated Mtb-infected neutrophils (p > 0.05). On the other hand, Kk was significantly decreased (p < 0.05) in the presence of Tempol (450 μM). DPI (32 μM) was equally effective in promoting this effect.
Tempol does not affect the viability of Mtb or human neutrophils
One and a half hours after incubation, 450 μM Tempol had no significant toxicity toward Mtb, as attested by counting CFU of the Tempol-treated Mtb group (figure 4A). Furthermore, four hours after incubation, there was no significant difference between the viability of 450 μM Tempol-treated human neutrophils and non-exposed neutrophils (p > 0.05, figure 4B), in which cells' viability was greater than 90%, after exposure. Control OD570 containing Triton-X-100-treated neutrophils was considered as baseline.
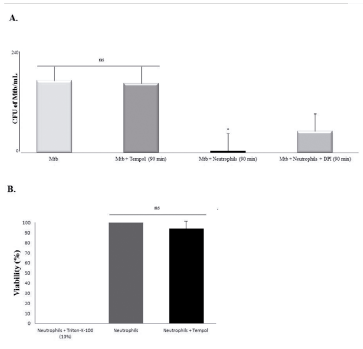
Figure 4 Effects of Tempol on Mtb and neutrophils viability. Mtb: Mycobacterium tuberculosis (H37Ra strain); CFU: colony-forming units. *p < 0.05; ns = non-significant (p > 0.05). A. Tempol (450 μM) had no influence on Mtb viability after 90 min of incubation (p > 0.05), whereas Mtb was efficiently killed by neutrophils after 90 min (p < 0.05) and DPI prevented the killing of Mtb by neutrophils. B. Four hours after incubation, 450 Tempol had no influence on neutrophils viability (p > 0.05).
DISCUSSION
In this study, first was demonstrated that Tempol decreased ROS levels during the oxidative burst of human neutrophils stimulated by different inducers, including Mtb H37Ra. The mechanisms of Tempol to decrease ROS levels are well-described in the literature, including its SOD mimetic properties to scavenge O2 •- [35, 38, 39]. Next, we investigated whether the decrease in ROS levels caused by Tempol could impair the mycobactericidal activity of neutrophils; because oxidants could synergize to such response to Mtb [11, 12, 16].
In this regard, using cytC reduction assay, Tempol decreased O2 •- levels. The literature states that Tempol can inhibit the oxidative burst with an EC50 ranging from ~50 to 450 μM [35]. Thus, 450 μM Tempol was adopted thereafter, which was observed a similar outstanding effect to decrease total (intracellular/extracellular) and total extracellular ROS (chemiluminescence assay). Furthermore, using cytC reduction assay, we detected that the opsonization of Mtb with autologous human serum (20%) did not significantly change the amount of extracellular O2 •- produced by neutrophils, so then only nonopsonized Mtb was used from then on. Studies have reported that non-opsonized Mtb interacts with the complement receptor type 3 (CR3) or mannose receptors of phagocytes in which these receptors can also act as pattern recognition receptors (PRR); thus, recognizing pathogen-associated molecular patterns (PAMPs) from Mtb and promoting neutrophil phagocytosis of mycobacteria [44-46]. Here, an ideal MOI (10) was also determined, which reflects the Mtb burden during TB disease in humans [47].
Highlighting the importance of ROS against Mtb, NADPH oxidase complex firstly form O2 •-, an oxidant that generates other potent antimicrobials ROS [48], and the O2 •- can be promptly removed by Tempol [36, 37, 39]. Moreover, we showed that thapsigargin, an inducer of unfolded protein response (UPR) via stressing of endoplasmic reticulum (ER), caused an augmentation in the steady-state levels of O2 •-, an effect probably attributed to its capability in mobilizing NADPH oxidase subunits, increasing the rate of assembly of this complex [49]. The role of endoplasmic reticulum stress-mediated apoptosis in M1-polarized macrophages (MO) during infection with Mtb (strain H37Ra) has been demonstrated, in that context, effectively removing intracellular Mtb and indicating that ER stress can be an important component of the host immune response against mycobacteria [50].
In the last analysis, at an indicated ratio of cell/Mtb (MOI 10) [42, 51], the neutrophils' mycobactericidal response was notoriously impaired by Tempol, as an extended effect of this nitroxide in decreasing ROS levels. Indeed, only the killing rate constant (Kk) was affected, as such mechanism is boosted by ROS, whereas the phagocytosis rate constant (Kp) was not affected [42]. Thus, Tempol impaired the intracellular killing of Mtb thanks to a putative diminution in ROS levels, without affecting phagocytosis.
The response of neutrophils to Mtb can involve ROS/RNS- and non-ROS/RNS-dependent mechanisms. Concerning the killing of Mtb in which ROS and RNS take part, it is noteworthy that NO (RNS) produced by MO is known to be the key contributor as an anti-Mtb agent [3, 52, 53]. Unlike MO, in neutrophils, at experimental conditions here established, a coordinated response involving NOS expression with NO production is apparently less pronounced than that involving ROS. Moreover, the killing of Mtb by neutrophils may also be via a nonoxidative process [54]. At the same time, the ROS here evaluated (O2•-, H2O2, HO•, HOCl, and 1O2) can also boost neutrophils' response against Mtb H37Ra, directly attacking mycobacteria and/or activating ETs, ETosis and apoptosis, unless those mycobacteria respond in a counteractive manner toward ROS [11, 12, 17-19, 23].
In this context, ROS can either directly destroy Mtb by damaging its DNA (e.g., oxidizing the nucleotide pool), oxidizing proteins (binding to amino-acid side chains like arginine, threonine, lysine, causing proline/histidine oxidation and converting tyro-sine into nitrotyrosine), and peroxidizing lipids (affecting cell membrane or cell wall-associated lipids) [53], or indirectly activate mycobactericidal mechanisms, including inflammatory response by undergoing ROS-dependent apoptosis [55]. In the last case, the mechanism of Mtb H37Ra (heat-killed)-induced apoptosis in neutrophils can be blocked by antioxidants [55].
The mechanism of mycobacterial killing via oxidants may occur in a strain-independent manner. Indeed, attenuated Mtb H37Ra can induce efficient neutrophil phagocytosis and lift oxidative burst, via specifics PAMPs present in this strain [8, 9, 56]. In this study, we did not pass Mtb suspension multiple times through a 27-gauge syringe, since Kalsum et al. related that neutrophil appears to respond more robustly to face aggregates of M. bovis than against a single cell [20]. In addition to the decreases in ROS levels and mycobactericidal response of neutrophils (as demonstrated in this study), Tempol (and other antioxidants) can decrease inflammatory cytokines [57], and skew T helper 1 (Th1) to Th2 response afforded by CD4+ lymphocytes [31, 58]. These factors further implicate potential risk, making the host more susceptible to Mtb (favoring active TB), since the main response against Mtb requires neutrophils N1/ MO M1 (mycobactericidal) and the priming of naive Th1 cells [59].
On the other hand, Tempol could be clinically useful in immune/inflammatory response-induced airflow obstruction and limitation, as seen in some pulmonary diseases [26-30, 61]. In these cases, the exacerbated immune response with high ROS levels can cause OS and ROS-dependent NET formation through which occur a thicker in mucus viscosity [60, 28, 61]. Moreover, mechanistically, NET activates the pathway of coagulation through electrostatic interactions between its histones and platelet phospholipids [13]. For example, in patients with severe COVID-19 associated pneumonitis and/or acute respiratory distress syndrome (ARDS), released NETs by neutrophils in response to SARS-CoV-2 can infiltrate the lung airway, interstitial spaces, and vascular compartments, as well as cause vascular occlusion [13, 28, 61]. The thick mucus secretions in the airways causing airflow obstruction and the deep vein thrombosis can also occur in TB patients, in which the response of neutrophils and/or MΦ to Mtb leads to high ROS levels and NET production relating to the clinical severity of TB [10, 13, 25, 26, 53, 62]. Therefore, unlike so far mentioned, would be desirable to prevent ROS formation, since OS can damage the host cells and Tempol showed an outstanding capability to scavenge ROS.
Regarding the redox balance, according to Yamatoa et al., Tempol caused an augmentation of NAD+ concentrations (due to recycling of redox states of this nitroxide), which could be considered during treatment with INH, because of an anti-Mtb efficacy of INH depend on NAD+ [34, 53]. For example, the combination of INH and an antioxidant, N-acetylcysteine (NAC), was more effective in combating intracellular Mtb than INH alone [63]. Furthermore, the redox balance in mycobacteria has been reported as an important target to control Mtb infection [53, 64]. Thus, these are positive arguments to justify antioxidant therapy during ATT (beyond antioxidants also prevent oxidative damage from hepatic metabolism of TB drugs). However, a caution with the use of antioxidants during ATT arises from the fact that these compounds may interfere with the mechanism of action of anti-TB drugs that promote oxidative stress in mycobacteria as part of their lethality, as demonstrated by Yano et al. [65], in which Tempol altered the intracellular redox cycling in mycobacteria and decreased clofazimine lethality. Meanwhile, Tempol, per se, had no anti-mycobacterial activity [65], a similar effect found in the present study.
Other effects of Tempol on neutrophils were here evaluated. Tempol did not induce cytotoxicity in neutrophils. Neutrophils were exposed to Tempol for 4 h, with no significant loss ofviability. Reportedly [66], besides showing minimal toxicity as reported in studies involving human and animal experimental models, Tempol at concentrations up to 500 μM do not present pro-oxidant effects [33]. On the other hand, during combinatorial therapeutic strategies, a pro-oxidant effect of Tempol (at high doses) could have anti-Mtb activity in addition to potentialize the action of antitubercular agents [53]. Also, of clinical relevance, there are potential medical applications of Tempol due to its extremely low toxicity [66], and the study of this nitroxide took place in clinical trials [67].
Recently, it was demonstrated that Tempol can inhibit protein disulfide isomerase (PDI) and, as this protein is coupled with the assembly of the NADPH oxidase complex, we did not rule out other activities of Tempol to down-regulate the levels of ROS [38]. Mechanistically, this pleiotropic effect can be owing to actions on mediators of NADPH oxidase activity, such as: up-stream (including activating factors like protein kinases and/or agents like PDI that mobilizing/folding granule components/subunits of Nox2) and/or downstream (directly scavenging ROS or blocking ROS-dependent apoptosis/NET formation) [38, 39].
As other considerations and perspectives, we found that Tempol can act to modulate the intracellular redox status of neutrophils and potentially interfere with microbicidal activity against Mtb; hence, influencing immune balance and health status. The production of several oxidants and their detoxification is a hallmark of the balanced confrontation between immune response and microorganisms, but this event may get out of the host's control. The mechanism by which Tempol acts can be harmful during a necessary immune response, since it decreases the concentration of oxidants and, consequently, the intensity of a microbicidal response. On the other hand, the same mechanism by which Tempol modulates the redox status of the cell can be useful to decrease the inflammatory process and OS that ultimately lead to tissue damage, as seen in exacerbated immune responses against some pathogens, as for example, SARS-CoV-2, a current public health problem responsible for a current relentless pandemic [68 - 71]. In this sense, this nitroxide is currently being clinically used and tested in patients with severe COVID-19, and our findings are in line with the current thoughts and trends of application for the various purposes for which the clinical uses of Tempol are intended [72, 73]. This study corroborates, in terms of concepts, and converges to justify the use of this nitroxide, since, mechanistically speaking, during the innate immune response to various pathogens, Tempol applications would have a wide field of applicability given the duality of the context of an immune response, as seen in the transition between innate and adaptive immunity and the associated MO1/MO2;N1/ N2;Th1/Th2 patterns, as well as the different phases in which this nitroxide could be useful with different associated outcomes [57 - 59, 68 - 80].
In summary, our findings support the concept that Tempol can interfere with the response of neutrophils to Mtb by modulating ROS levels and impairing the in vitro anti-Mtb activity of these cells (summarized in figure 5A and 5B). We highlighted the double-edged sword regarding the use of antioxidants during ATT (when used at lower dosages in which they present an effect purely antioxidant, as seen in this study), as Tempol skews the balance between oxidants and antioxidants in neutrophils. Nevertheless, the induction of a decrease in ROS levels could be desirable in those conditions in which the pathogenic roles of oxidants are highlighted, as also seen in TB cases. Unbalanced immune response with an augmentation of ROS levels worsens pulmonary diseases, and Tempol could halt further injury during the resolution of inflammation. The interplay between Mtb and phagocyte function on the influence of ambient factors (including exogenous antioxidants) is here considered, since they can manipulate the neutrophil oxidant/antioxidant balance and, under certain contexts, promote a counterbalanced host immune response, for the control and resolution of infectious diseases, having it impact to predict alterations that could further shed light on the pathogenesis of TB and, therefore, contribute to both prevention and therapeutic.
After evaluating the risk/benefit profile, the use of compounds with no side effects that increase the natural human antioxidant defense could be considered, as a co-therapy during ATT, rather than pleiotropic and non-selective antioxidants (that interfere with immune response). Therefore, this study provides insights regarding the role of antioxidants on the neutrophil response to Mtb, evidencing alterations in the balance of neutrophil immune response, so that clinical implications should consider these findings, and further studies should be conducted in vivo.
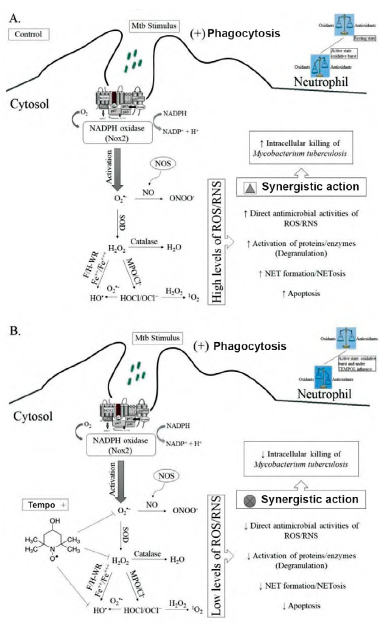
Figure 5 An overview of the effects of Tempol on the neutrophil response to Mycobacterium tuberculosis, with possible outcomes. Fe2+: Ferrous iron; Fe3+: Ferric iron; F/H-WR: Fenton/Haber-Weiss reactions; HO•: hydroxyl radical; H2O2: hydrogen peroxide; HOCl: hypochlorous acid; NOS: nitric oxide synthase; MPO: myeloperoxidase; Mtb: Mycobacterium tuberculosis; NADPH oxidase: nicotinamide adenine dinucleotide phosphate oxidase; NET: neutrophil extracellular traps; NO: nitric oxide; Nox2: neutrophil oxidase isoform 2; 1O2: singlet oxygen; O2•-: superoxide; ONOO-: peroxy-nitrite; RNS: reactive nitrogen species; ROS: reactive oxygen species; SOD: superoxide dismutase.
A. Control: After being stimulated by Mtb (active state), neutrophil Nox2 forms high levels of superoxide (O2 •-). Then, spontaneously or via SOD, O2 •- is converted into H2O2. The H2O2 can follow two ways: (i) The conversion into HO• through Fenton/Haber-Weiss reaction or (ii) The conversion into HOCl (in the presence of MPO/Cl-); The HOCl can spontaneously originate OCl- or be converted into HO• (in the presence of O2 •-) or into 1O2 (in the presence of H2O2). Optionally, O2 •- may react with NO to form ONOO-, but NOS expression/activation in neutrophils is low, so that these cells generate small amounts of NO. ROS and RNS present direct anti-Mtb activity, and/ or they can activate other anti-Mtb mechanisms, including degranulation (activation of peptides/ proteins/enzymes), NET formation and release/NETosis and apoptosis. These mechanisms induced by ROS/RNS synergize to Kill Mtb.
B. Tempol +: Decreased levels of ROS/RNS are observed in Tempol-treated neutrophils. When neu-trophils are treated with Tempol, this nitroxide acts by scavenging oxidants formed through Nox2; thus, preventing oxidative burst-generated augmentation of ROS/RNS and blocking synergetic mechanisms, ultimately, decreasing Mtb killing. Noteworthy, phagocytosis is not affected by Tempol.