INTRODUCTION
Accurate prediction of foetal weight has been of great interest in obstetrics due to its significant impact on the course and outcome of labour and delivery 1,2 Incorrect estimation of foetal weight can result in multiple and often dangerous complications for the pregnant mother and the foetus 2. It has been suggested that accurate estimation of foetal weight may contribute to successful management during labour and care of the newborn in the neonatal period, and help avoid complications associated with foetal macrosomia or low-birthweight newborns, thereby decreasing perinatal morbidity and mortality 3,4. Unfortunately, birth weight is unknown until birth takes place 5. As foetal weight cannot be measured directly, it must be estimated from foetal and maternal anatomical characteristics 3.
Methods for accurate prediction of birthweight prior to delivery are required to establish strategies designed to reduce adverse pregnancy outcomes 5,6. The tools currently used to estimate foetal weight include the evaluation of foetal growth assessment, and can be broadly classified as maternal methods, clinical methods, and imaging methods like ultrasonography 7,8. Ultrasound estimation (USS) is more expensive and complicated than maternal or clinical estimation, but it is currently expected to provide a more accurate prediction of birthweight 6. In practice, the most common equations for calculating estimated foetal weight (EFW) by USS are reported to be the Shepard and Hadlock formulae 9. The currently used Hadlock formula for foetal weight estimation has an error rate of 20%, which may fluctuate depending on the skills of the examiner, equipment base, conditions of the examination, as well as the stage of pregnancy or labour 10-12. Regardless of the formula used, the accuracy of the sonographic estimate of the EFW is affected by suboptimal imaging and biological variation 13,14. In addition, the accuracy of the sonographic estimate decreases with increasing birthweight 15, and tends to be over-estimated in pregnancies suspected of being large for gestational age (LGA) and underestimated in pregnancies with preterm premature rupture of membranes (PPROM) and suspected foetal growth restriction (FGR) 16. The sensitivity and specificity of the Hadlock formula in the detection of foetal macrosomia are 62% and 93%, respectively 4,12,16,17. The level of intra/inter-observer variability in foetal measurement, and the impact of errors on growth assessment and discrepancies within study designs, exceed 14% with 95% confidence intervals 18-20.
Two large studies have compared foetal weight prediction (clinical method vs. ultrasonography) and found that USS was more accurate than clinical estimation of birthweight in the lower range (<2,500 g) 21. However, this was not the case in the 2,500-4,000 g ranges, where clinical estimation was more precise 22. Finally, both methods were equally adequate in the higher range of birthweight (>4,000 g) 23. On the other hand, Chauhan et al. 4 found that ultrasound estimation of foetal weight was more accurate than clinical estimation in preterm pregnancies, but not so in term of post-term pregnancies. Due to such limitation, researchers have explored other sonographic or clinical parameters or a mix of them, correlating with foetal weight, with a higher predictive value. We therefore aimed to derive a reliable equation based on maternal clinical parameters and third trimester ultrasound biometry (combined method) and compare it with the ultrasound-estimated foetal weight (EFW) calculated using the Hadlock 2 formula.
MATERIAL AND METHODS
Design and population
We performed a prospective cohort study in women seen at La Ribera University Hospital (LRUH) in Valencia (Spain) for pregnancy follow-up and delivery between January and December 2016. We included women with a first prenatal appointment and USS between 5 and 12 weeks of pregnancy, single-foetus pregnancy with no foetal abnormalities, and birth between 38 and 40 weeks. Data on maternal pre-pregnancy weight, symphysis-fundal height measurement (SFH), and USS examination at third trimester (34 weeks) by a gynaecologist had to be documented in the electronic health record. Pregnancies complicated by polyhydramnios, hypothyroidism, preeclampsia, gestational diabetes and oligohydramnios were excluded from the study. The LRUH is a public 300-bed tertiary-level healthcare centre which provides health services to 250,000 people approximately. It is the sole hospital providing maternity services to pregnant women in the area, with an average of 3,000 births per year.
Sample size and sampling
During the study period,2,017 women consented to be included in the study. A representative sample size was calculated. The null hypothesis was that there were no statistically significant differences in birthweight accuracy between the clinical and sonographic methods. Assuming an expected difference between both estimates of weight (EFWmr and EFWa) greater than or equal to 200 g to consider statistically significant differences, accepting an alpha risk of 0.05 and beta of 0.2 in a two-sided test, a common standard deviation of 450, and a drop-out rate of 30%, then the necessary sample would be 39 participants within each group. However, all pregnant women who agreed to participate during the study period (one year) were included.
Procedure
The opportunity to participate in the study was offered before the assessment. An informed consent was requested by the gynecologist in charge. At the time of recruitment in the third trimester, demographic variables such as maternal age and country of origin were gathered from electronic maternity records at discharge. Self-reported tobacco use in the third trimester was also obtained from the electronic medical records at the health centres by community midwives during the prenatal control period. All of these data were collected by the researcher in charge. Measurements obtained and recorded on the prenatal charts at LRUH by the community midwife responsible for the women’s routine prenatal visits were also evaluated by the researchers at 34 weeks and the delivery date, in order to reduce biased measurements. Data on obstetrical variables were also collected. SFH was measured in centimeters with nonelastic measurement tape from the upper border of the symphysis pubis to the top of the uterine fundus, or reversed direction 13. Ultrasound examinations at 34 weeks were performed by sonographers with appropriate training on the SONOLINE G60 model (SIEMENS). Ultrasound estimated foetal weight (EFW) was calculated using the Hadlock 2 formula 8,20, using four foetal indices: Biparietal diameter (BPD), femur length (FL) and abdominal circumference (AC), recorded at 34 weeks at LRUH (Log 10 weight = 1,326 - 0.00326 AC x FL + 0.0107 HC + 0.0438AC + 0.158 x FL). Birthweight was recorded in the delivery room by midwives following clamping and umbilical cord separation, using a digital scale (SECA®, Vogel & Halke GmbH & Co. Hamburg, Germany) within a 10 g accuracy. Weight was documented in the electronic medical record together with all other birth-related data.
The gestation-adjusted projection (GAP) method was applied to each pregnancy by calculating the ratio between the EFW, at the time of the remote ultrasound, and the median foetal weight for that gestational age by foetal gender 19,24. This ratio was then multiplied by the median birthweight for the gestational age by foetal gender at delivery resulting in the GAP-predicted birthweight. The ultrasound estimations (EFWa) were each compared to the actual birthweight. Finally, methods were compared: Estimated foetal weight by GAP method (EFWa) (USS at third trimester with the Hadlock 2 formula adjusted by gestational age at birth and foetal gender), and estimated foetal weight with the combined clinical and GAP method (EFWmr) (multivariate lineal regression method) were calculated to compare with the actual birthweight. Variables to be measured: Predictive variables were age, gestational weight gain and parity, foetal gender, gestational age at birth (GA, in complete weeks), smoking in 3rd trimester (as dichotomous variable). Gestational weight gain (GWG) was calculated after applying the difference between weight on the day of delivery and weight on the first consultation documented in the clinical record. Pre-pregnancy BMI was calculated taking into account the initial weight (5-8 weeks of pregnancy) and the maternal height squared (kg/m2). Result variables: Birth-weight, gestation-adjusted projection (GAP).
Statistical analyses
Descriptive statistics data are presented as mean and standard deviation for continuous variables, or median and interquartile range for non-normally distributed and categorical variables provided as range and percentage. Normality of continuous variables was assessed using the Kolmogorov-Smirnov test. In the event that variables did not adjust to normality, a non-parametric test was used. In the bivariate analysis, correlations between the dependent (birthweight) and independent variables collected were studied using the Student t-test to compare mean quantitative variables.
All variables with statistical significance (p<0.05) and clinical value were included in a multivariate analysis to identify the most accurate birthweight prediction equation (EFWmr). To analyse the relationship between birthweight and different covariables (clinic, demographic and obstetrical variables), an adjusted multiple linear regression model was applied using a stepwise method for variables shown to have an effect on birthweight. In the linear regression model, the partial F was used to compare the different models obtained. The principle of parsimony was established in order to select the simplest model with the smallest number of variables.
The accuracy of the different methods (EFWa/EFWmr) for estimating foetal weight was then evaluated by calculating the Pearson correlation R coefficient between the estimated foetal weights obtained using each equation and actual birthweight. Intraclass Correlation Coefficient (ICC) was used to evaluate the degree of agreement of both methods with the actual neonatal weight. Absolute error was defined as the absolute value of EFWa//EFWmr minus the actual birthweight, and the relative error value as the absolute EFWa//EFWmr error divided by the birthweight multiplied by 100.
Mean error differences between both methods were assessed by the paired t-test for Gaussian continuous data. The mean error represents the sum of the positive (overestimation) and negative (underestimation) deviations from the actual birth-weight, approximating zero in a method with very low or no systematic error. A threshold of relative error within ±10% of actual birthweight was chosen as the cut-off value for examination accuracy. The percentages of birthweight predictions within 10% of the actual birthweight were calculated and compared using the McNemar test. Each outcome measure was then assessed for overall foetal weight and for three categories of weight <2,500 g, 2,500-<4,000 g, and ≥4,000 g. The overall correlation coefficients of ultrasound-based, and clinically determined estimates were also compared. All statistical tests were performed using the SPSS Version 23 software package (IBM SPSS Inc., 2008 Chicago, IL, USA; www.spss.com); p values of <0.05 reflect statistical significance.
Ethical considerations
The study was conducted in accordance with the basic principles for all medical research (Declaration of Helsinki), respecting the applicable legal precepts regarding the protection of personal data. The study was approved by the Research Ethics Committee of La Ribera University Hospital (Reference no. #441-14). Considerations such as confidentiality, voluntary participation, and full information on the nature of the study were extended to all participants. The attending gynaecologist recruited the women after the third trimester USS and obtained their informed consent to participate in the study.
RESULTS
Of the initial 2,017 pregnant women delivered at the LRUH during the study period, 458 women (22.7%) did not meet the inclusion criteria: 36 (7.9%) twin pregnancies, 27 (5.9%) <35 weeks, 334 (72.9%) >40 weeks at birth, 22 (4.8%) poly-hydramnios, 34 (7.4%) oligohydramnios, and five (1.0%) foetal deformities. Missing data from antenatal maternity records resulted in 335 women (16.6%) subsequently excluded from the final analysis:108 (32.2%) without documented third trimester US, and 227 (67.8%) without SFH recorded.
Therefore, a total of 1,224 (60.7%) pregnancies were finally included for analysis. The mean age of the participants was 31.0 ± 6.0 years (median 32.50, range 18-42), mean gestational age at delivery was 39.14 ± 1.5 weeks (range 35-40), and 48.6% (595/1224) women were primiparous. The mean actual birthweight of the study population was 3,254 ± 448.4 g. Forty-nine (4.0%) had a birth-weight of <2,500 g, 1,118 (91.3%) weighed between 2,500-3,999 g, and 57 (4.7%) weighed >4,000g. The demographic and clinical characteristics of the study population are depicted in Table 1.
Table 1 Demographic and obstetric characteristics of 1,224 women seen for pregnancy follow-up and delivery at La Ribera University Hospital (LRUH) in Valencia (Spain), 2016.
Value | ||
---|---|---|
Maternal national origin (n (%)) | Spain | 993 (81.1) |
European countries | 83 (6.8) | |
Other European countries | 19 (1.6) | |
North Africa | 83 (6.8) | |
Central-South America | 32 (2.6) | |
Asia | 14 (1.1) | |
Foetal gender (n (%)) | Male | 620 (50.7) |
Female | 604 (49.3) | |
Birthweight (n (%)) | <2,500 g | 49 (4.0) |
2,500-3,999 g | 1118 (91.3) | |
>4,000 g | 57 (4.7) | |
Pre-gestational BMI | <18.5 | 36 (2.9) |
18.6-24.9 | 765 (62.5) | |
25.0-29.0 | 273 (22.3) | |
>30.0 | 150 (12.3) | |
Smoker in 3rd Trimester (n (%)) | Yes | 96 (7.8) |
No | 1128 (92.2) | |
Maternal age (mean ± SD) | 31±6.0 | |
Parity (mean ± SD) | 0.65±0.8 | |
Gestational age (mean ± SD) | 275.6±7.6 | |
Birthweight (g) (mean ± SD) | 3254±448.4 | |
GWG (kg) (mean ± SD) | 11.9±5.3 |
BMI: body mass index; GWG: gestational weight gain; SD: standard deviation
The variables that showed statistical significance with birthweight in the bivariate analysis were: country of origin (p=0.007), parity (p<0.001), maternal age (p=0.007), pre-gestational BMI (p<0.001), SFH (p<0.001), smoker status (p=0.0012), gestational weight gain (p=0.005) and EFWa (p<0.001).
A multivariable model was performed. The predictive variables and coefficients in the multivariate analysis are shown in Table 2. The following equation was derived: EFWmr (g) = -560.4 + (SFH x 51.6) -(smoker [0=no, 1=yes) x 74.6) + (GAP x 0.59).
Table 2 Multivariate linear regression analysis predicting birthweight by clinical method in 1,224 newborns of pregnant women seen at La Ribera University Hospital (LRUH) in Valencia (Spain), 2016
Standardized coefficient | 95% CI | |||||
---|---|---|---|---|---|---|
B | SE | p-value | Lower limit | Upper limit | ||
(Constant) | -560.417 | 125.336 | <0.001 | -806.314 | -314.519 | |
SFH | 51.602 | 3.692 | <0.001 | 44.360 | 58.845 | |
Smoker 3rdTrimester | -74.638 | 33.621 | 0.027 | -140.599 | -8.676 | |
EFWa* | 0.593 | 0.023 | <0.001 | 0.547 | 0.639 |
R:710; R2 adjusted: 503 95% CI: 95% confidence interval; B: unstandardized regression coefficient; SE: standard error of the estimated; SFH: symphysis-fundal height at 35-40 weeks; EFWa: estimated foetal weight by ultrasound scan at 33-35 weeks with the Hadlock 2, by GAP method (adjusted by gestational age at birth and foetal gender).
Differences for the weight of the newborn between EFWmr and EFWa are shown in Table 3. Actual birthweight had a strong positive Pearson two-tail correlation with both the combined method (EFWmr) and ultrasound (EFWa) estimated foetal weights (R=0.91, p<0.001 vs. R=0.87, p<0.001, respectively), as shown in Figure 1. The difference in birthweight prediction between both methods (EFWa and EFWmr) and the actual birth-weight was analysed. For the EFWa, the difference with the actual birthweight was 187.4g ± 361.4 (95% CI: 167.1-207.7), whereas for the EFWmr the difference was -0.68g ± 315.6 (95% CI: -18.3-17.1), differences being statistically significant, respectively (paired t-test p<0.001; p<0.001). The difference in weight estimation between the two methods, based on the difference between the estimated weight and the actual final weight, was 188.1g ± 361 (95% CI: 178.2-197.9; paired t-test p<0.001). The mean relative value error for EFWmr was lower than for EFWa (0.97% ± 10.1 vs. 6.67% ± 12.1), and the differences were statistically significant by paired t-test (5.7% ± 2.0, 95%CI: 5.4-6.0, p<0.001).
Table 3 Accuracy of combined method and ultrasound estimated foetal weights of 1,224 newborns of pregnant women seen at La Ribera University Hospital (LRUH) in Valencia (Spain), 2016 (n=1,224).
ABW prediction (95% CI) | Mean relative error (95% CI) | Prediction within ±10% (%) | ICC | |
---|---|---|---|---|
EFWa | 3442.17 | 6.67% | 65.3 | 0.743 |
(3419.08-3465.27) | (5.99-7.35) | (p<0.001)* | ||
EFWmr | 3254.08 | 0.97% | 82.7 | 0.803 |
(3236.22-3271.93) | (0.41-1.55) | (p<0.001)* |
ABW: Actual Birth weight; EFWa: estimated foetal weight by third-trimester ultrasound scan using the Hadlock 2 formula adjusted by gestational age at birth and foetal gender; EFWmr: estimated foetal weight by multivariate linear regression; 95% CI: 95% Confidence interval; ICC: intraclass correlation coefficient with the actual birthweight.
*p-value: obtained by reliability analysis using a two-way mixed model with absolute agreement type.
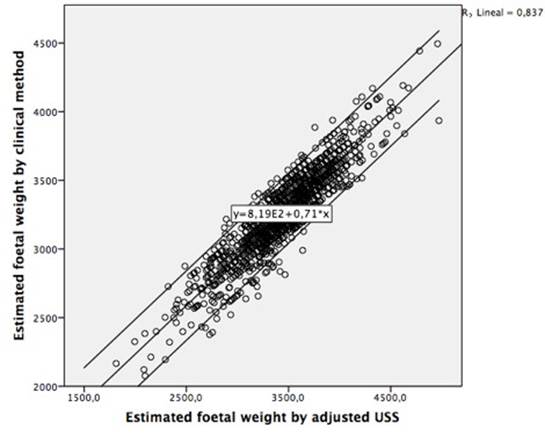
Figure 1 Correlation between multivariate linear regression and ultrasound foetal weight estimation of 1,224 newborns of pregnant women seen at La Ribera University Hospital (LRUH) in Valencia (Spain), 2016
The proportion of ultrasound estimated weights (EFWa) within <10% of the actual birthweight was significantly lower than that obtained with the combined method (EFWmr) (65.3% vs. 82.7%) the difference being significant (McNemar test, p<0.001). The ICC was significantly higher in the case of the combined method estimation versus actual weight, when compared to ultrasound estimation versus actual weight (0.803 vs. 0.743, p<0.001).
There were statistically significant differences between weight estimation methods by birth-weight categories. For newborns with <2,500 g birthweight, the proportion of estimates within <10% of the actual birthweight for the EFWmr was significantly greater than for EFWa (20.4% vs. 16.3%, p=0.005). For babies with normal birth-weight (2,500-3,999g), the combined method was significantly greater than the ultrasound method (84.5 vs. 65.7%, p<0.001). And finally, in macrosomic newborns (>4,000g) the proportion of estimates within <10% of the actual birthweight for the combined method were lower than the ultrasound method (100.0 vs. 98.2%), however the differences were not statistically significantly (p=0.238). Table 4 presents the differences in error for both estimations’ methods and for different birthweight categories.
Table 4 Comparison predictions within 10% between the accuracy of combined method and ultrasound estimated foetal weights of 1.224 newborns of pregnant women seen at La Ribera University Hospital (LRUH) in Valencia (Spain), 2016
Birth weight categories | EFWmr Prediction within <10% n (%) | EFWa Prediction within <10% n (%) | p-value* |
---|---|---|---|
<2,500 (n=49) | 10 (20.4%) | 8 (16.3%) | 0.005 |
2,500-3,999 (n=1,118) | 945 (84.5%) | 735 (65.7%) | <0.001 |
>4,000 (n=57) | 57 (100.0%) | 56 (98.2%) | N/A |
EFWa: estimated foetal weight by third-trimester ultrasound scan with the Hadlock 2 formula adjusted by gestational age at birth and foetal gender; EFWmr: estimated foetal weight multivariable regression; N/A: insufficient cell number to perform analysis.
*p-value: McNemar test
DISCUSSION
In this paper, we report a novel multivariable model based on maternal characteristics capable of predicting neonatal weight in a large population of normal pregnancies. Only a few studies have previously compared the predictive capacity of birthweight by clinical and ultrasonic measurements 3,22,23,25,26. Our results suggest that the EFWmr model that takes into account SFH, smoking and EFWa can adequately estimate neonatal weight at term (mean relative error and prediction within 10%). Additionally, our study shows a statistically significant difference in explaining birthweight when it is compared with EFW by adjusted USS (EFWa).
When the result was compared with actual birthweight, the EFWmr prediction increased its accuracy to within 5.7% of actual birthweight (± 188g), a result improving the findings reported by Emechebe et al. (9.2%; 299 g), and resembling best reported values for clinical methods described by others authors 3,27,28. For the ultrasonographic method alone, our results (around 7%) are also consistent with other studies where the mean relative error of predicted birthweight varied from 6% to 12% of actual birthweight 9,15. Based on our results, EFWmr yields a prediction within 10% of actual birthweight, around 83% in line with the report by Curti et al., (84%) and outperforming the data by Shittu or Kayem et al. (70% and 58%, respectively) 13,26.
Our study has shown that EFWmr is as accurate as EFWa within the normal birthweight range in accordance with other researches that have shown accuracies (mean relative error) between 7-19% 3,16. Regarding the category under 2,500 g, however, the accuracy of the clinical method is lower. Only 20% of birthweights below the 2,500 g threshold are estimated properly by the clinical method within 10% of actual birthweight, in line with Scioscia or Dudley et al. 8,29 who reported a mean relative error ranging between 13-19%. In the high birth-weight (≥4,000 g) group, the difference in the means was not statistically significant in predicting macrosomic newborns, in line with other studies 3,19, suggesting that both ultrasound and the clinical method are equally accurate in predicting foetal macrosomia 22.
Overall, the mean relative error for the clinical method was lower than the ultrasound method (1%). This suggests that the clinical method of foetal weight estimation is generally more accurate than the ultrasound method. Whilst our findings are consistent with those reported elsewhere 23,24, the small proportion of low birthweight and macrosomic newborns in our sample warrants caution in the interpretation of the results and suggests that further studies with larger samples and centred on these subpopulations would be necessary. Interestingly, the mean absolute error can be misleading because it is the sum of positive and negative deviations from actual birthweight, thus artificially reducing the difference between actual birthweight and estimated birthweight. It is a measure of systematic error in each method rather than a variation from birthweight. On the other hand, the mean relative error reflects the variability noted regardless of its direction and, as such, being a more accurate predictor of differences in relation to actual birthweight. Thus, for practical clinical purposes, the variation between predicted birthweight and actual birthweight is best expressed as mean relative error 3,22.
This study has several limitations. First, it might be argued that the knowledge of ultrasound measurement by the clinician measuring fundal height or the suboptimal record completion identified in our clinical health records would influence later measurements. However, the correlation coefficients relating USS and SFH measurements to birthweight did not depend on the order in which these measurements were carried out (data not shown), and only available data were analysed. This suggests that the order of measurement did not significantly impact accuracy in our study. Second, extreme birthweight values (<2,500 and >4,000 g) were infrequent due to the exclusion criteria used in the study. At present, absolute error in birthweight prediction using USS (EFW) varies from 6% to 12% 3,5.
Accuracy can be improved in two different ways: first, by controlling the limitations of the technique and second, by adding maternal variables from the multivariate model to the ultrasound measurement. We recommend that further research determine the accuracy of the clinical approach we present here in situations, which can alter the evaluation of birth-weight in women with different obstetrics’ risk. This study had a strength, such as a representative and large sample size and we used a standardized method of clinical estimation that had been found previously to correlate well with birthweight.
CONCLUSIONS
The EFWmr model outperformed the gestation-adjusted projection (GAP) method, and predicted birthweight within 1% relative error, suggesting that our mathematical model improves the routine ultrasound measurement in the third trimester of pregnancy to estimate the neonatal weight at term.