Introduction
In recent years, the development of chemical compounds has yielded a large number of substances that are used to protect crops, among them, pesticides, which are classified based on some of their main characteristics, such as acute toxicity, half-life, chemical structure and use. 1
Pesticide poisoning by compounds such as organophosphates (OP) and carbamates is a global health issue that causes thousands of deaths each year. A report from the World Health Organization (WHO) shows that about three million cases of OP poisoning have a deadly outcome every year around the world. 2 In 1978, the same organization classified these compounds based on their hazard level or degree of acute toxicity, defined as highly toxic chemicals that cause acute health effects as a result of a single exposure or exposures of short duration. 3
Pesticide toxicity is measured using median lethal dose (LD50) or median lethal concentration (LC50) and is classified into four classes: IA, IB, II and III (Table 1). 3 Some of these compounds are promptly absorbed after entering the body either by inhalation or ingestion. Once absorbed, they accumulate in fat, liver, kidneys and salivary glands. 4 Pesticides are classified as non-persistent, moderately persistent, persistent and permanent in terms of half-life (Table 2) 5 and as synthetic, natural, organic, chemical and biopesticides according to their chemical nature (Table 3). 6
Table 1 Classification of pesticides by hazard.
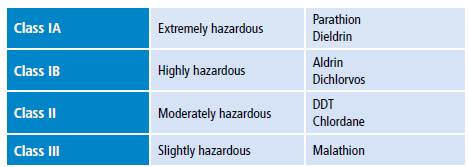
Source: Own elaboration based on Eddleston & Phillips. 3
Table 2 Classification of pesticides by half-life.
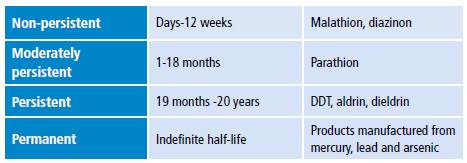
Source: Own elaboration based on Briggs & Carson. 5
These substances are used according to the activity in which pest control is required: agriculture, livestock, care of domestic animals, treatment of structures, maintenance of green areas, maintenance of water reserves, industry, home, public health, etc. 7
In Colombia, according to the Instituto Nacional de Salud (National Institute of Health), 24 748 cases of poisoning by chemical substances were reported in the public health surveillance system (SIVIGILA by its acronym in Spanish) until the epidemiological week 39 of 2016. Although a large number of reported poisoning cases are related to the drugs group (7 918 cases), the pesticides group comes second with 6 535 cases. 8
The main action mechanism of OP and carbamate pesticides is the inhibition of carboxyl ester hydrolases, in particular acetylcholinesterase, which leads to acetylcholine accumulation.
Acetylcholinesterase is an enzyme found in the synapses of the central and peripheral nervous tissue, in neuromuscular junctions 9 and in red blood cells. There are two types of this enzyme in the body: acetylcholinesterase and pseudocholinesterase.
On the one hand, acetylcholinesterase (AChE) or true cholinesterase has an almost specific affinity with the natural substrate of acetylcholine, breaking it down into its two component parts, acetic acid and choline (ACh). On the other hand, pseudocholinesterase (BChE) or non-specific enzyme hydrolyses many different synthetic and natural esters, including acetylcholine.
Multiple BChE isoenzymes are found in the liver, blood plasma and nervous tissue, which are inhibited by OP compounds in a similar way to AChE. Organophosphates inactivate AChE by phosphorylation of the hydroxyl group of serine located in the active site of AChE. Phosphorylation occurs due to the loss of a leaving group of the organophosphate and the establishment of a covalent bond with AChE. Once AChE is activated, acetylcholine accumulates in the synaptic space, resulting in overstimulation of the muscarinic and nicotinic receptors, causing neurotoxic alterations (neuropsychological diseases, delayed neuropathy and peripheral neuropathy). Clinical effects are evident through the activation of the autonomic and central nervous system and nicotinic receptors in skeletal muscle. 10
Patients with pesticide poisoning show a broad spectrum of toxic effects, ranging from gastrointestinal symptoms to cardiac, immunological or neurotoxic diseases. The mortality rate depends on the type of compound used, the amount ingested, the patient's health state and the opportunity for diagnosis and treatment. 11
López-Guarnido 12 has suggested that, besides inducing the neurotoxic alterations described above, when these compounds come into contact with the organism, another molecular mechanism -lipid peroxidation- is involved with oganoclorates, carbamates and OP toxicity, since reactive oxygen species (ROS) may increase intracellular oxidative processes, producing lipid peroxidation in the membranes of certain cells.
The main objective of this review is to highlight the implication of oxidative stress as a toxic action mechanism and its association with different diseases.
Free radicals and oxidative stress
Free radicals are defined as chemical species that have one or more unpaired electrons in their outermost orbital; for a chemical compound to become a free radical, gaining or losing electrons is necessary. Unpaired electrons in the outer orbital of the molecule are highly reactive 13; by interacting with several chemical compounds free radicals give up an unpaired electron and easily take the electron from a stable molecule and join to it. 14
It is well known that oxygen is an essential element for the survival of living organisms. This element is used by cells for organic compounds oxidation, cellular respiration, energy production, among others. Under normal conditions, molecular oxygen can accept up to four electrons in its external orbital, resulting in two water molecules. 12 However, when there is a leak of electrons, oxygen may undergo a univalent reduction by accepting an electron, divalent by accepting two electrons or trivalent by accepting three electrons, forming the so-called ROS. The products of these reactions are, respectively, superoxide anion radical, hydrogen peroxide and hydroxyl radical. ROS can react easily to cellular endogenous substrates. 12
These endogenous substrate reactions with ROS can cause oxidative damage to different biomolecules such as lipids, proteins, nucleic acids, carbohydrates, etc. Since blood cell membranes are rich in fatty acids, naming the action of these species on the phospholipid membranes is of particular interest, as will be seen later on. 15
The action of reactive oxygen species on lipid chains is the most frequent oxidative process in the organism and occurs when a free radical is fixed to a carbon of the alkyl chain (a group formed by carbon and hydrogen atoms) of a fatty acid, thus beginning lipid peroxidation during a process called initiation. 12
Fatty acids of the cell membrane are susceptible to attacks, since they contain methylene groups separated by double bonds. Once the radical lipid is generated, it reacts with oxygen forming a peroxy radical, which can give rise to endoperoxides or take a hydrogen atom of a methylene carbon from another nearby polyunsaturated fatty acid and form a new free radical lipid and a hydroperoxide. The former is combined with an oxygen molecule to produce a chain of peroxidative damage propagation. 16
Antioxidant systems
The human body has mechanisms that counteract the harmful effects of reactive oxygen species. It is important to bear in mind that not only the human species has these mechanisms. An antioxidant is defined as a substance capable of inhibiting oxidative damage in a target molecule. 15
Given the destructive potential of free radicals, the body has strong endogenous antioxidant mechanisms to avoid the damage they cause. This activity includes superoxide dismutase (SOD) enzymes, glutathione peroxidase (GPx), catalase (CAT) and thioredoxin reductase. There are also preventive antioxidants that are metals sequestrants such as transferrin, ceruloplasmin, albumin, among others. 17
Other substances with antioxidant capacity can be observed, such as reduced glutathione, a tripeptide found in high concentrations in cells that have sulphydryl groups, and plays an important role in the body. Other substances such as ubiquinone (coenzyme Q), which is obtained mostly in an exogenous manner through food, vitamins E and C and carotenoids are also important antioxidants. 17 Furthermore, defense mechanisms such as superoxide dismutase (SOD), catalase (CAT), glutathione reductase (GR), among others, can exert a protective role against the effects of reactive oxygen species, induced by certain xenobiotics. 18 There are also physiological antioxidant systems such as the microvascular system, which is responsible for maintaining adequate levels of O2 in tissues by regulating blood vessel pressure. 12
The imbalance between the production of reactive oxygen species and the compensation mechanism of antioxidant systems, both physiological and biochemical, results in reactive oxygen species, which in turn cause some processes such as lipid peroxidation in cell membranes.
Oxidative stress induced by pesticides
Often, pesticide poisoning occurs in agricultural workers such as mixers, loaders and applicators, who are in direct contact with pest control areas. Low molecular weight and high liposolubility give pesticides properties such as high absorption and high toxicity. 19
Some studies have corroborated that acetylcholinesterase enzyme inhibition is significantly associated with the increase in reactive oxygen substances, not only in workers who are exposed to OP pesticides, but also in those exposed to bipyridyl herbicides such as paraquat, which proves oxidative stress induction through an increase in lipid peroxidation and a decrease in antioxidant capacity. 20,21
As previously stated, ROSs increase oxidative processes within the cell and produce lipid peroxidation in cell membranes. 12 A biological effect has been suggested as a possible action mechanism of lipid peroxidation caused by pesticides on blood cells by means of an electrophilic attack on certain cellular components, process during which ROSs are generated. 22
A study conducted by Praksam et al.21 indicates that greater erythrocyte fragility and alterations in erythrocyte membrane fluidity, secondary to lipid peroxidation and generated by the oxidative stress caused by pesticides, have been observed in workers who apply them.
López et al.23 indicated that different pesticides, including OPs, were reported as oxidative stress inducers due to the generation of free radicals and alteration in antioxidant defense mechanisms. This study showed that farmers exposed to pesticides had low levels of superoxide dismutase (SOD) and glutathione reductase (GR) compared to the control group. Individuals with a decrease in AChE >15% showed decreased SOD and CAT activity during the same period. Glutathione peroxidase and glucose-6-phosphate dehydrogenase remained unchanged in the exposed population.
Another study conducted by Gultekin et al.24 evaluated oxidative stress in workers who used OP, synthetic pyrethroids and carbamate pesticides. The results indicated that chronic exposure to these compounds was associated with an increase in the activity of catalase, SOD and lipid peroxidation in erythrocytes (p<0.05). It was concluded that chronic exposure of humans to pesticides can result in stimulation of antioxidant enzymes.
Multiple studies provide evidence of the tendency of OPs to disturb oxidative balance, which leads to oxidative stress. In their study, Soltaninejad et al.25 reviewed 127 articles, including 112 experimental studies, and found 15 relevant studies on humans. The appearance of lipid peroxidation in cell membrane, the alteration of antioxidant capacity levels and the protective effects of natural and synthetic antioxidants against histopathological and biochemical alterations induced by OP are the most important evidences of the implication of oxidative stress in toxicity. It was concluded that the assessment of oxidative stress parameters in blood can be useful for monitoring exposed persons.
Hematological alterations and lipid peroxidation induced by pesticides
The lipid peroxidation observed in blood cell membranes caused by chronic exposure to OP pesticides and carbamates leads to think that, in fact, it is possible to observe morphological and numerical alterations in said cells, as some studies have suggested.
Díaz et al.26 studied 71 individuals exposed by accident to OP pesticides and determined the levels of cholinesterases, blood count and platelet count. The hematological assessment showed a tendency to a decrease in platelet count (p<0.05) with values below 109/L.
Bhatti et al.27 described that the erythrocyte membrane is an excellent model for studying the interaction with prooxidants. Results of rat erythrocytes exposed to said insecticide showed damage of the erythrocyte membrane caused by oxidative stress, with an increase in lipid peroxidation and decrease in its phospholipid content.
Parrón et al.28 described a decrease in the mean corpuscular hemoglobin concentration in 38% of the workers studied, without any clear anemia. This same study found that 37% of fumigators had some type of symptom or sign of toxicity, such as spontaneous abortions, depression, headache, tremor or paresthesias.
Wafa et al.29 evaluated hematological alterations in workers with chronic exposure to pesticides; the results showed that leukocytes, lymphocytes, monocytes and platelet counts were significantly higher in workers exposed to pesticides than in controls. In contrast, hemoglobin and hematocrit were significantly lower in the farmer group compared to controls. This study demonstrated that long-term exposure to pesticides leads to the alteration of some hematological parameters.
Hundekari et al.30, in a study conducted in 2013 in the north of Karnataka (India) including 150 patients diagnosed with OP poisoning admitted to the hospital and 30 subjects without poisoning as controls, estimated erythrocyte cholinesterase, superoxide dismutase, erythrocyte catalase, glutathione erythrocyte peroxidase activity, as well as total plasma antioxidant capacity and hematological parameters. The study showed a significant decrease (p <0.001) in total plasma antioxidant capacity at all levels of OP poisoning compared to controls.
In acute OP poisoning, there is an accentuated overproduction of reactive oxygen species leading to lipid peroxidation. Ranjbar et al.31 reported that significant lipid peroxidation is accompanied by decreased total plasma antioxidant capacity and decreased erythrocyte cholinesterase activity. Due to the consumption of non-enzymatic antioxidant agents that the body cannot compensate in a short period of time, a lower total antioxidant capacity is observed.
The susceptibility of erythrocytes and lymphocytes to oxidative stress due to exposure to pesticides is a function of the general balance between the degree of oxidative stress and the antioxidant defense capacity. With all of this in mind, the compounds may directly or indirectly modify the antioxidant defense capacity of the exposed subjects and, therefore, affect their susceptibility to oxidative stress. 32
Wesseling et al.33 showed that cells continuously suffer oxidative stress, despite the increase in the activity of the antioxidant defense mechanism, as reflected in the increase of erythrocyte SOD, CAT and GPx activity. The production of free radicals is so high that it even exceeds the antioxidant defense system, thus producing lipid peroxidation. This study revealed that the white blood cell count increased significantly (p <0.001) in all cases of OP poisoning and leukocytosis showed mild neutrophilia compared to controls. The results indicated a significant decrease in the mean hemoglobin value of the OP poisoning group compared to the control group, perhaps as a result of the decreased synthesis of hemoglobin. Another possible interpretation was the lack of iron incorporation in hemoglobin, which leads to a decrease in the size of red blood cells. The significant increase in the leukocyte count observed in patients intoxicated by OP meant that the immune system is activated, which could be a positive response for survival.
Alterations in neuronal cells and oxidative stress induced by pesticides
Several authors have studied the generation of ROS and their role in dopaminergic cell death. Parkinson's disease (PD), for example, is a chronic and progressive neurological disorder associated with loss of dopaminergic neurons in the substantia nigra of the brain. It is believed that oxidative stress plays an important role in dopaminergic neurotoxicity. On the other hand, respiratory chain inhibition in complex I has been described in most processes related to neuronal degeneration in PD. Environmental factors such as neurotoxins, pesticides and genetic mutations in associated proteins contribute to the mitochondrial dysfunction that precedes ROS formation. 34 Rodríguez et al.35 carried out an extensive analysis to establish the role of superoxide radical, oxidative stress and their behavior in dopaminergic cell death induced by Parkinsonian toxins. The researchers demonstrated that paraquat induces an early increase in mitochondrial oxidative stress, which precedes the increase in cytoplasmic ROS levels. This herbicide also induced the transcriptional activation of son- and NF-kB reporters and lipid peroxidation. The loss of dopaminergic neurons induced by toxins such as paraquat, rotenone and 1-methyl-4-phenylpyridinium (MPP+) is associated with oxidative stress.
Nakamura et al.36 indicated that 1-methyl-4-phenylpyridinium or cyperquat [MPP (+)] is selectively toxic to dopaminergic neurons and has been widely studied as an etiological model of PD because MPP (+) is involved in both mitochondrial dysfunction and its pathogenesis. MPP (+) can inhibit mitochondrial complex I activity and its toxicity has been attributed to mitochondrial depolarization and subsequent ROS generation. MPP (+) induced death in rat fetal dopaminergic neurons selectively. Clarifying the effects of MPP (+) on energy metabolism can provide information on the dopaminergic neuronal degeneration mechanism in PD.
Zawada et al.37 found that excess superoxide production in the brain predicts the loss of neuronal cells, suggesting that the death of dopaminergic neurons in the substantia nigra in PD can be an example of this condition. ROS production induced by 1-methyl-4-phenylpyridinium (MPP) (active neurotoxic metabolite of 1-methyl-4-phenyl-1,2,3,6-tetrahydropyridine MPTP), a herbicide related to paraquat, activates in the dopaminergic neurons of the substantia nigra in response to superoxide-induced neurotoxicity. The results of this study led to conclude that the production of superoxide generated by NADPH oxidase present in the neurons of the substantia nigra contributes to the loss of such neurons in PD.
Colovic et al.38 studied the toxic effects of the diazinon, diazoxon and 2-isopropyl-6-methyl-4-pyrimidinol degradation products and compared them with the toxic potential of the original compound. The toxicity induced by different concentrations of the investigated compounds was evaluated in vitro by analyzing acetylcholinesterase, ATPases, defense antioxidant enzymes and lactate dehydrogenase activity and the malondialdehyde level in rat brain synaptosomes. It was found that diazinon inhibits acetylcholinesterase and Na (+)/K (+) ATPase in a dose-dependent manner. Prooxidative properties were demonstrated by causing an increase (up to 10%) in the activity of antioxidant enzymes and malondialdehyde levels as a marker of lipid peroxidation. The increase in diazoxon concentrations activated CAT (up to 30%), SOD (up to 50%) and GPx (up to 30%) and significantly increased the malondialdehyde level (up to 50%).
The investigated hydrolysis product of diazinon 2-isopropyl-6-methyl-4-pyrimidinol did not alter noticeably the activity of acetylcholinesterase, Na (+)/K (+) - ATPase, CAT, GPx and the peroxidation level of lipids (up to 10%). Although this metabolite (diazinon) is known to be non-toxic, it stimulates SOD up to 30%. High concentrations of diazinon and its metabolites affect the activity of lactate dehydrogenase as a marker of synaptosomes integrity. Changes in the biochemical parameters studied in rat brain synaptosomes can serve as indicators of toxicity from exposure to OP or its byproducts. 38
Mehta et al.39 studied the effect of chlorpyrifos (O,O'-diethyl-3,5,6 phosphorothionate-trichloro-2-pyridyl, CPF), an organophosphorus insecticide, on acetylcholinesterase (AChE) activity, lipid peroxidation and different activities of ATPases on rats. The researchers found that CPF caused a significant inhibition of synaptosomal AChE activity in different regions of the brain and that the inhibition varied between 36% and 82%, depending on the dose received. Oxidative stress also occurred, which resulted in a marked increase of membrane lipid peroxidative damage in a dose-dependent manner. The levels of malondialdehyde (MDA) and 4-hydroxy-2-nonenal (4-HNE), two major end products of lipid peroxidation, increased significantly in all regions of the brain. Na+ / K+, Mg 2+ and Ca (2+) - ATPases were inhibited to different degrees in the forebrain, midbrain and hindbrain of the treated rats.
Metabolic alterations and oxidative stress induced by pesticides
According to Calderón-Salinas et al.40, "using different models to study the effects of increased glucose concentration has allowed to propose that oxidative processes are involved with the pathogenesis, progression, complications and poor prognosis of diabetes mellitus".
In this regard, there is growing evidence that OP pesticides alter glucose homeostasis causing insulin resistance and type 2 diabetes. The formation of advanced glycosylation end products, the accumulation of lipid metabolites, the activation of inflammatory pathways and oxidative stress are involved in the pathogenesis of insulin resistance. All these molecular processes activate a series of stress pathways that involve a family of serine kinases, which in turn have a negative effect on insulin signaling. Experimental and clinical data suggest an association between these molecular mechanisms and OP compounds. Furthermore, it has recently begun to be understood that OPs promote insulin resistance and increase the risk of type 2 diabetes. 41
Acute pancreatitis is another well-known complication of OP poisoning 42. Epidemiological findings indicate that the incidence of this pathology is high, based on several physiopathological reports. 43 The precise underlying mechanisms are not yet well defined, although it is believed that pancreatic ductal obstruction or ROS production are involved. 44-46 Oxidative stress due to acute exposure to OP has been reported 47, and it has been unequivocally demonstrated that lipid peroxidation is one of the molecular mechanisms involved in the cytotoxicity induced by them. 31,48
In a study conducted by Joshi et al.49 -in which repeated oral doses of dimethoate (DM) (20 and 40 milligrams per day for each kilogram of body weight for 30 days) were administered to rats in order to cause alterations in glucose homeostasis and biochemical alterations in the pancreas-, different signs of glucose intolerance were observed among the rats that were administered DM after an oral glucose load (3 grams per kilogram of body weight). It was also observed that DM caused a significant increase in blood glucose levels with the concomitant inhibition of acetylcholinesterase activity and the depletion of reduced glutathione contents in the pancreas. In addition, DM caused a significant pancreatic damage reflected in the increase of amylase and lipase. Likewise, a dose-related elevation was observed in ROS levels in the pancreas of the treated rats.
Genotoxicity and oxidative stress induced by pesticides
Shadnia et al.11 conducted a study to evaluate genotoxicity and oxidative stress in workers who used OP. Blood leukocytes and erythrocytes were examined in a group of 21 workers who handled pesticides and an equal number of control subjects for genotoxicity and oxidative stress parameters. The tail length of the comet assay was used to measure DNA damage. Regarding lipid peroxidation, CAT, SOD and GPx activity in erythrocytes was measured and analyzed as biomarkers of oxidative stress. In addition, acetylcholinesterase activity was measured as a biomarker of toxicity. The seniority in the position of the workers in the factory was 97 months.
The results of Shadnia et al.11 showed that chronic exposure (>6 months) to OP pesticides was associated with an increase in CAT, SOD and GPx activity in erythrocytes. Acetylcholinesterase activity showed no significant differences between both groups. The results also indicated that chronic exposure to OP pesticides was associated with an increase in DNA damage. It was concluded that chronic exposure to OP pesticides can result in stimulation of antioxidant enzymes and increased DNA damage in the absence of decreased levels of acetylcholinesterase. This study proposed conducting routine monitoring of genotoxicity, concomitant with acetylcholinesterase activity, in workers occupationally exposed to OP insecticides.
In the Sultana study 50, endosulfan+ chlorpyrifos, chlorpyrifos+ profenofos and endosulfan+profenofos were used. These mixtures were evaluated in human peripheral blood lymphocyte cultures using cell viability and genotoxicity assays, and using the chromosomal aberration assay and the comet assay. The LC50 values for cytotoxicity were 3.50μM, 4.18μM and 10.5 μM for profenofos, endosulfan and chlorpyrifos, respectively. When combined in equimolar concentrations, LC50 values for cytotoxicity were 1.4μM, 1μM and 2.0M for chlorpyrifos+endosulfan, chlorpyrifos+profenofos and endosulfan+profenofos, respectively. High concentrations of individual pesticides (0.5-4.0μM) and low concentrations of pesticide mixtures caused significant DNA damage. These findings indicate a synergistic effect of toxicity by chlorpyrifos+endosulfan combination. The mixture of chlorpyrifos+profenofos showed additive toxicity, while an antagonistic effect was observed for the endosulfan+profenofos combination. Said synergy may be caused by complementary pesticides that act simultaneously in different ways, with an increase oftheir effectiveness, while an additive interaction would imply that the chemical products are acting because of the same mechanism and the same objective. According to this study, the toxicity analysis of pesticide mixtures can serve as an important biomarker of occupational exposure. 50
Table 4 shows the main damage mechanisms found in the literature review.
Conclusions
After conducting an exhaustive literature review, it is possible to conclude that oxidative stress caused by OP pesticides, carbamates and some organochlorines is a highly relevant toxicodynamic mechanism in toxic damage. This damage mechanism is related to multiple diseases that involve systems, such as the hematopoietic, endocrine and nervous systems. Those compounds are also capable of producing genotoxicity.
Although most studies were conducted in experimental animals such as rats, some of them were made in humans, showing very interesting aspects that require more documentation and research, since oxidative stress plays an important role in the development of multiple diseases.
In Colombia, research has not yet been carried out regarding the impact of these substances on hematopoietic tissue, oxidative stress induced by pesticides or alterations caused by these compounds in cell membranes of different tissues or organs, which is why it is necessary and of great importance for the health of Colombians to undertake this challenge.