Introduction
Cataracts are the leading cause of blindness worldwide; in Colombia, based on data presented by Furtado et al.,1 it is estimated that about 24 000 patients lose their vision as a result of this condition. One of the strategic components for treating cataracts and reducing the number of cases of blindness is surgery.
The calculation of the intraocular lens (IOL) power that is implanted in the patient during cataract surgery is a determining factor for the success of this surgical procedure, which is measured from uncorrected distance visual acuity2,3 and is assessed based on the need for glasses.
With the development of technologies that allow for a better eye examination and the improvements in materials and design of intraocular lenses, the calculation of IOL power has become more important, as its high predictability makes patients and surgeons have high expectations of the refractive outcome.4
In this sense, IOL power calculation is an essential part of preoperative examination of cataract surgery and several factors have helped improve its effectiveness, such as the development of surgical technique with small incisions that do not affect astigmatism and the emergence of non-contact optical biometry by partial coherence interferometry and optical biometry by swept source method. Thus, the fact that optical biometry provides more accurate, reproducible, and operator-independent results means that the refractive status information established by surgeons can be compared, adjusted, and corrected to refine the outcome.2
Cataract surgery is the most performed outpatient surgical procedure in developed countries, and the refractive outcomes that directly affect vision depend on the accuracy of many biometric variables. The axial length (AL) of the eyeball, the corneal curvatures (KM), the size of the lens and cornea, and the anterior chamber depth (ACD) are the most significant biometric parameters used in current formulas to calculate the implanted IOL power in cataract surgery. In this regard, the study by Olsen, cited by Lee et al.,5 shows that imprecision in ACD, AL and KM measures contributes to 42%, 36% and 22%, respectively, of the error in predicted refraction after implantation of the IOL in cataract surgery, which affects uncorrected visual acuity with glasses.
The distribution of AL and other ocular biometric parameters follows a Gaussian distribution; however, their values are different depending on the race of the patient.6 At this point, it should be noted that there are very few population-based studies on biometric parameters measured with optical methods,6-12 and they are even more scarce in Colombia.13-17
In 1996, Holladay et al.18 showed that AL should not be used only to guide the IOL power calculation formula, as it is independent of other biometric factors of anterior segment dimensions such as ACD. According to Holladay's classification, nine clinical conditions can be found based on the interaction between AL (short, normal, and long eyes) and ACD (small, normal, and large anterior segment), which are: nanophthalmos, microcornea, microcornea + axial myopia, axial hypermetropy, normal, axial myopia, megalocornea + axial hypermetropia, megalocornea and buphthalmos. Most current formulas work well in eyes described as normal in this classification; however, the prediction is inaccurate for all other clinical conditions.
Thus, as a first step to improve the results of cataract surgery in the country, the present study aimed to characterize the ocular biometric parameters of Colombian patients over 40 years of age who were candidates for cataract surgery and to determine the prevalence of the 9 clinical conditions proposed by Holladay according to the interaction between AL and ACD.
Materials and methods
Study population
For the selection of the study population, a non-probabilistic sampling was performed for convenience and the ocular biometry results of all patients over 40 years of age who underwent cataract surgery at the Clínica Santa Lucía, a reference institution in Medellín (Colombia), between January 2014 and January 2015 (781 patients, 831 eyes), were reviewed. The results of those patients with signal-to-noise ratio <2 in AL were not taken into account, nor were left eyes in the case of bilateral results due to the high correlation of contra-lateral eyes. Consequently, the final sample consisted of 716 eyes.
Procedures
Data were analyzed based on the ocular biometry records of all patients, which were obtained using the non-contact partial coherence interferometry IOLMaster device (Carl Zeiss AG, Oberkochen, Germany). In addition, the averages of the five AL samples taken and the three repetitions of the other parameters were included.
Variables
Data on age, sex, AL, flat and steep KM (K1 and K2), ACD and white-to-white distance (WTW) were collected from the optical biometer database. Astigmatism was determined as the difference between K1 and K2.
Data analysis
Data were collected in a spreadsheet created in Excel (Microsoft Corp., Redmond, WA, USA). Measures of central tendency (mean and median) and dispersion (Standard deviation and interquartile range (IQR)) were used for analysis and established according to the data distribution of each variable, which was determined by the Kolmogorov-Smirnov normality test.
The t-Student, Chi-square or Mann-Whitney U tests were used to compare the variables AL, K1 and K2, ACD and WTW according to age and sex, considering the normal or non-normal distribution of the variable. All statistical analyzes were carried out using the EPIDAT 4.2 software (Sergas, Santiago de Compostela, Spain).
Data were then grouped according to the 9 eye types suggested by Holladay based on ACD (small, medium, or large) and AL (short, normal, or long) and using the <5% percentile (P<5) for small and short eyes, the percentile 5-95% (P5-P95) for medium and normal eyes, and the percentile >95% (P>95) for large and long eyes. This determined the percentages to observe the distribution that makes up each group.
Ethical considerations
The study took into account the ethical principles for medical research involving human subjects established by the Declaration of Helsinki19 and the scientific, technical and administrative standards for health research of Resolution 8430 of 1993 of the Ministry of Health of Colombia.20 The research protocol was approved by the Ethics Committee of the Universidad Pontificia Bolivariana (Medellín, Colombia) according to Minutes number 1 of February 11, 2015.
Results
The median age of the participants was 70 years (IQR: 71.70-76.90) and most of the eyes were female (62.3%). Regarding ocular biometric parameters, the median AL, K1, K2, ACD and WTW were 23.37±1.51mm, 43.52±2.06, 44.63±2.22, 3.03±0.41mm and 11.76±0.45mm, respectively.
Mean AL in men was 23.62±1.37mm and in women, 23.21±1.67mm. Mean values for ACD and WTW were also higher in men (3.10±0.42mm vs. 2.98±0.40mm and 11.85±0.47mm vs. 11.71±0.42mm respectively), although the most curved KMs were found in women (Table 1).
Table 1 Distribution of biometric parameters by sex.
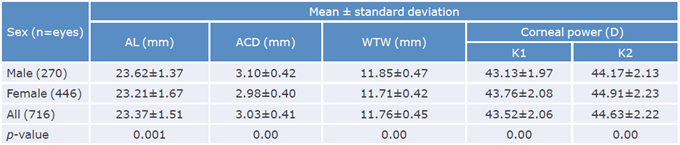
AL: axial length; ACD: anterior chamber depth; WTW: white-white distance; K1 : flat keratometry; K2: steep keratometry.
Source: Own elaboration.
After grouping the eyes by age, changes in AL were observed, as it decreased as age advanced. Thus, the mean for people under 50 was 23.84±2.41mm and for those over 80 years, 22.96±1.03mm; the same happened with K1, which had mean values of 42.60±3.29 and 43.73±1.63, respectively. These changes were greater in women across age groups (Table 2).
Table 2 Distribution of biometric parameters according to age and sex.
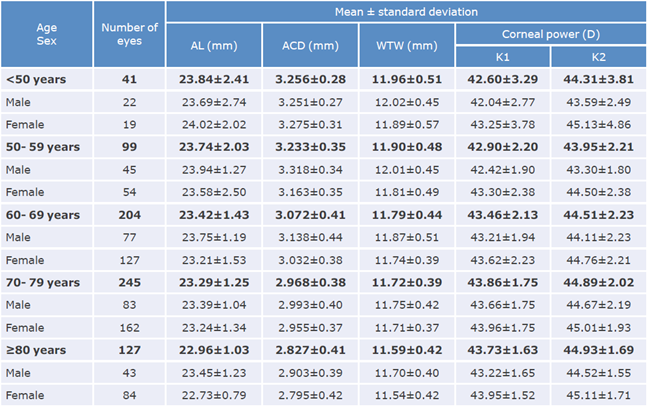
AL: axial length; ACD: anterior chamber depth; WTW: white-to-white distance; K1: flat keratometry; K2: steep keratometry.
Source: Own elaboration.
When establishing the normality ranges for AL, it was determined that P5 corresponded to 21.51mm and P95 to 25.86mm. Thus, 33 eyes had an AL <P5, 648 an AL between P5 and P95, and 35 an AL >P95, which allowed to establish that 4.61% of the eyes were short, 90.50% were normal, and 4.89% were long.
As for the normality ranges for ACD, it was established that P5 corresponded to 2.33mm and P95 to 3.74mm. Thus, 34 eyes were found with an ACD <P5, 650 with an ACD between P5 and P95, and 32 with an ACD >P95, so it was established that 4.75% of the eyes were small, 90.78% were medium, and 4.47% were large.
When adding the eyes with normal AL and mean ACD, it was found that 599 (83.65%) were between P5 and P95 and classified as normal, and that the remaining 117 (16.34%) were outside the normal range. These results were combined to classify the eyes according to clinical conditions suggested by Holladay (Table 3).18
Table 3 Percentage distribution according to the 9 eye types suggested by Holladay based on anterior chamber depth and axial length in patients who were candidates for cataract surgery.
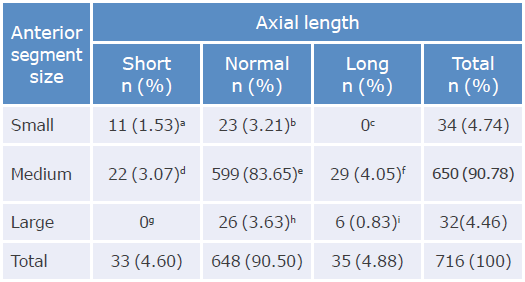
a: nanophthalmos; b: microcornea; c: microcornea + axial myopia; d: xial hypermetropia; e: normal; f: axial myopia; g: megalocornea + axial hypermetropia; h: megalocornea: i: buphthalmos.
Source: Own elaboration.
Finally, astigmatism >1.50 diopters was found in 151 eyes, corresponding to 21.08% of all eyes evaluated.
Discussion
According to the literature, the mean AL varies depending on the race of the patient. For example, the average in Australia and Iceland is 23.44mm;6,21 in China, 23.23mm,7 and 23.13mm in Mongolia.12 Therefore, it seems logical that the mean established in the present study (23.37mm) is between these anthropomorphic extremes.
Similarly, it was established that the mean AL found here is similar to those of other studies with a Latino population such as Shufelt et al.,11 in which the mean was 23.38mm. However, this result differs slightly from that found in Mexico by Morales-Avalos et al.,17 who reported a value of 23.09mm, which could be explained by the fact that the average height is lower in Mexico than in Colombia.
Changes in AL depend not only on race, but also on variations within each race, as evidenced in population studies conducted in different regions of the same country. For example, the mean found in Australia in the suburban population included in the Blue Mountains Eye Study (BMES) is 23.44mm,6 while the mean is 23.12mm in the rural population of Myanmar.10 Moreover, in the south of China, the mean value is 24.07mm,22 while in Singapore it is 23.03mm.7 In Colombia, the mean found in Bogotá is 23.46mm,15 which is slightly higher than the mean found in the present study in population of Medellin (23.37mm).
These racial differences have been explained by the correlation between AL and height and other anthropometric parameters that change in each country, and even in each region within the same country. These differences are also affected by the correlation between AL and myopia, which is more prevalent in countries and areas with greater educational development.10,11,21 It is worth noting that this correlation may be associated with the fact that people living in countries with more educational development are more exposed to factors that can cause damage to their visual health, such as computer screens, tablets, or smartphones.
AL was greater in men than in women, 23.62mm and 23.21mm, respectively, which is consistent with previous studies.6,11,22 These differences appear to be caused by men being taller21 and flatter KM.
There was also evidence of a reduction in AL in people older than 60 years.6 This change could be explained by emmetropization to compensate for the increase in the refractive power of the eye in older people, or by a change in future generations, although a biological change is unlikely, that involves increased myopia as the country's educational development rises, as explained above and described in other studies comparing urban and rural areas with low educational development.6,7,10,22
The decline in ACD as age increases has also been documented in other research.7,16,21,22 In the present study, this phenomenon was more evident in women over 70 years of age, which could be explained by the increase in lens size with age; this reduction would also explain the higher prevalence of angle closure reported in this population.23
Since Ridley's reports24 and his initial calculations for the implantation of the first intraocular lenses, significant advances have been made because, before that, a standard lens was placed in all patients. However, it was soon observed that myopic patients were under-corrected and hyperopic patients were overcorrected; then, with the appearance of ultrasonography and the possibility of measuring AL, the calculations began to be corrected and different formulas were developed to make these measurements.
Even today, it is still debated which formulas best predict refractive outcomes. Multiple studies have been done in different scenarios and it has been concluded that they have different performances depending on AL. Thus, in short eyes, the Hoffer Q and Holladay 1 formulas are more predictable, while the SRK-T has better results in long eyes.25,26 It has also been established that, in general, fourth generation formulas (Holladay II, Haigis and Barret) and optimized formulas work better outside normality ranges.5
In this sense, knowledge of the normality and distribution of eyes in Colombia regarding these variables could improve the performance of surgeons. Therefore, it should be noted that the present study found that only 84% of the eyes were classified with AL and ACD within the normal ranges and that, consequently, 1 out of 10 patients should have the IOL power calculation formula modified and adjusted to their type of eye according to the normality ranges: short eyes with AL <21.50mm and long eyes with AL >25.85mm. Most formulas deduce the final position of the lens, but if the anterior segment and ACD are out of normal ranges, even taking AL into account, the prediction will be affected, and errors will occur using the classic formulas.
Similarly, it is important to stress that more than 20% of patients who are candidates for cataract surgery have corneal astigmatism ≥1.5 diopters, which should be considered for correction in the same procedure, as this is a factor that directly affects the final outcome.
It should be noted that one of the limitations of the present study, due to its cross-sectional nature, was that it was not possible to know with certainty the changes in the biometric parameters that occur with age, but they had to be assumed with the different generations.
Conclusions
The present study found that 85% of participants had normal biometric parameters. Therefore, for the remaining 15%, it is necessary to take precautions when calculating IOL power, such as the use of fourth generation formulas, like Holladay 2 in long eyes, since the same refractive behavior will not be obtained in this type of eye with traditional prediction formulas.
On the other hand, according to Holladay's classification, it was found that, excluding normal eyes, the most frequent eyes were those with myopia and axial hypermetropia, and the least common, those with microcornea and megalocornea. In addition, it was established that 1 out of 5 patients requires planning the correction of astigmatism to achieve a better result.