INTRODUCTION
Carbon dioxide (CO2), is the most harmful greenhouse gas (GHG) produced by anthropogenic activities such as deforestation and the burning of fossil fuels; currently, the emissions of these gases have increased steadily, the most recent emissions estimate worldwide are for 2018, where the average concentration of CO2 reached 407.8ppm, methane (CH4) 1.869ppm and nitrogen oxide (N2O) 331.1ppm, of which emissions from land-use changes such as deforestation have not yet peaked, although they amounted to 5.5 GtCO2 equivalent (WMO, 2019).
Colombia emits 240 million tons of CO2, 0.46% of the world total, caused mainly by the agricultural sector with 54.2%, secondly, the energy sector with 29.2%, followed by waste and industrial processes with 16.7% (IDEAM et al., 2015).
In the department of Nariño, the majority of municipalities develop agricultural and livestock activities. In recent years, the concentration of GHGs from enteric fermentation, inadequate manure management, consumption of fossil fuel, the use of agrochemicals and nitrogen fertilizers for crops has increased; however, there is no research or regional theoretical references on the subject that allow a real picture of this issue (León et al., 2012).
According to the Conference of the Parties (COP 21) to the United Nations Framework Convention on Climate Change (UNFCCC, 2015), an agreement was reached to avoid an increase in average global temperature of more than 2°C. Colombia has agreed to address the climate change by reducing 20% of its GHGs by 2030, taking concrete adaptation measures such as increasing protected areas, formulating national plans and implementing water resource management instruments, seeking to initiate agricultural extension oriented towards efficiency in the use of resources (water, soil, and fertilizers), promoting alternative production systems through the implementation of agroforestry systems (i.e. silvopastoral systems) (Arbeláez et al., 2015).
For this reason, it is necessary to mitigate climate change and its possible effects on present and future generations, which is a major challenge for the economy and the science dedicated to environmental conservation. One way to counteract this phenomenon lies in the sequestration, storage, replacement, and reduction of atmospheric CO2 through agroforestry systems (Agudelo, 2016), which fix carbon above and belowground, making them one of the main alternatives as carbon sinks (Forero et al., 2018).
Based on the above, the main objective of this research was to determine the organic carbon stored in four land uses.
MATERIALS AND METHODS
Location. This study was conducted in the Mijitayo micro-basin, located in the municipality of Pasto at 1º 11'19.49"N and 77º 18'26.12"W at an altitude of 2850 meters above sea level, average annual precipitation of 840 mm, temperature of 12 to 13°C and relative humidity of 87.4%, on an andisol soil, with clay to loamy texture, pH of 5.6, organic matter of 9.9%, and nitrogen (N) 0.40% (Mera and Zamora 2007).
Selection of farms. The selection of the farms was done through field visits, taking into account some criteria such as authorization by the owners, accessibility for sampling, similirity of species, trees older than five (5) years with the same age.
Farms´ background. The characteristics found in the different land use helped to maintain a more uniform pattern of study, thus reducing experimental error and giving more evident and comparable results. As a result, four farms in San Felipe district, in Mijitayo micro-basin, were selected (Table 1).
Table 1. Description of land uses for the determination of carbon stored at two depths (30 and 60cm).
Location (Coordinates) | Land use | Species | Age | Spacing | Density |
---|---|---|---|---|---|
Agrosavia CI Obonuco (1°11'52.08"N - 77°18'11.57"O) | Fodder bank | Acacia (Acacia decurrens Willd) | 10 - 12 years | 1 * 1m | 10,000 trees/ha |
San Calletano Farm (1°12'14.4" N -77°18'28.8"O) | Potato monoculture | Parda variety (Solanum tuberosum L.). | 4 to 5 months | 30 * 50cm | 6.666 plants/ha |
Finca El Arrayán (1°12'18" N - 77°18'28.8"W) | Shelterbelts | Alder (Alnus acuminata H.B.K) | 7 years | 1.5 * 1.5m | 267 trees/ha |
Lida Meneses Farm (1°12'15"N -77°18'27"W) | Natural pasture | Kikuyo (Pennisetum Clandestinum Hochst. ex Chiov) | 3 years | Scattered | Undetermined |
Experimental Design. A Complete Randomized Blocks design (RCBD) with bifactorial arrangement (Factor A: different land uses (fodder banks, potato crops, shelterbelt and natural pastures), Factor B: different depths (0-30cm and 30-60cm) was carried out. The combination resulted in 8 treatments with three (3) repetitions.
T1: Fodder bank at 30 cm depth
T2: Fodder bank at 60cm depth
T3: Potato monoculture at 30 cm depth
T4: Potato monoculture at 60 cm depth
T5: Shelterbelts at 30 cm depth
T6: Shelterbelts at 60 cm depth
T7: natural pasture at 30 cm depth
T8: natural pasture at 60 cm depth
Determination of soil organic carbon storage (COS)
Field sampling phase. The selection of plots was determined for each land use (Natural pastures, shelterbelts, fodder banks, and potato (S. tuberosum) crops, where each was considered as a homogeneous and independent land (sampling unit). Randomized samples were taken in twelve (12) different areas at two (2) depths with (Moreno and Lara, 2003). To determine the organic carbon in the soil (COS), the adjusted methodology of Moreno and Lara (2003), was carried out. A 50 m2 plots were set in each land use, then three subsamples were taken. These samples were homogenized to obtain a final sample of 500g of soil. Subsequently, they were stored in hermetically sealed bags, duly labeled and sent to the Laboratories of the University of Nariño.
Laboratory phase
The following aspects were taken into account in this phase:
Bulk density. It was determined by measuring the volume of a known mass of powder sample, that have been passed through a sieve, into a graduated, obtaining the weight of the solids and the pore space. Then the formula (e1) by IGAC (1979) was used:
Where:
Da: Bulk density
Pvs: test tube weight + soil: 16.23 g
Pv: empty test tube weight: 15.09 g
Pw: apparent volume occupied by the soil (ml)
Percentage of organic carbon in the soil (%C). It was estimated by the method of Wet Digestion or method MacDicken, (1997) proposed by Walkley and Black (1934); obtaining the total organic carbon of a complete soil sample or some of its fractions through the following formula (e2):
Where:
CO: Soil organic carbon (%)
Lm: Sample reading in ppm of the calibration curve Vf: Final solution volume
Pm: Sample weight in grams
Pw: percentage of moisture in the dry soil at 105°C (moisture correction factor) 10,000: correction factor to express the result as a percentage
Carbon stored in the different land uses. The storage of organic carbon in the soil with the methodology proposed by Andrade and Ibrahim (2003);Holdrige,(2000), where the depth of the soil, the percentage of organic carbon and the apparent density were taken into account (e3):
Where:
CA: Stored Carbon
%CO: Percentage of carbon in the soil.
da: Bulk density
Ps: Soil depth l
Statistical analysis
To establish which type of land use and depth presented the greatest capacity for organic carbon storage analysis of variance, ANDEVA, was carried out. For the treatments that presented significant statistical differences, a Tukey means comparison test was performed with a 95% probability, using the Statistical Analysis Software (SAS ®).
RESULTS AND DISCUSSION
Carbon stored in the soil. In the organic carbon stored in the four land uses (Natural pastures, Shelterbelts, fodder banks, and potato crop) at two depths (30 and 60cm), when analyzing the different land uses, marginal statistical differences were found (Pr = 0.0573), and at the depths of 30 and 60cm the differences were significant (Pr <0.0061). However, in the interaction of land use by depth, no significant statistical differences were found (Pr < 0.0659) (Table 2).
Table 2. ANOVA of meanings obtained for the quantification of carbon in different uses and soil depths.
Effect | Num DF | Den DF | F-V | Pr > F |
---|---|---|---|---|
Land use | 3 | 14 | 3.18 | 0.0573 |
Depth | 1 | 14 | 10.42 | 0.0061 |
Land use*depth | 3 | 14 | 3.01 | 0.0659 |
Significant differences (p<0.05).
When analyzing the different land uses, significant differences were found (P<0.0061), showing that the processes of carbon accumulation may be different in each of the uses, due to environmental conditions associated with the type of soil, vegetation, precipitation, temperature, which over time presented lower carbon accumulations (Lorenz and Lal, 2015).
On the other hand, the fodder bank was had the greatest amount of carbon with 97.89tC.ha-1 (Figure 1); however, these values differ from the study carried out by Giraldo et al. (2008) who verified that in a silvopastoral system (SSP) with acacia (Acacia decurrens Willd) and Kikuyo grass (Pennisetum clandestinum Hochst. ex Chiov) species the amount of carbon was 251 tC.ha-1. This discrepancy is probably due to the tree species, spacing, organic matter in the soil, age of the components, soil types, site characteristics, climatic factors, and silvicultural management, which allow greater accumulation of carbon in the soil profile (Carvajal et al., 2012).
In this sense, Lorenz and Lal, (2015), assure that in forage banks established in Canada with approximately 13 years, the deposits of carbon in the soil was 1.25tC ha-1 while in Costa Rica, in systems of 10 to 15 years, had of 173tC ha-1. These authors state that environmental conditions directly affect soil carbon storage.
Ibrahim et al. (2007) determined that in fodder banks in Nicaragua and Costa Rica, a greater amount of soil carbon was obtained with 88.46tC.ha-1, and 84.2tC.ha-1 respectively. Contrary in Colombia, lower values were reported (52.34tC.ha-1). Concerning the above, the results obtained in this study are close to those found in Nicaragua.
According to the above, Post and Kwon (2000) state that there are several factors influencing the COS, such as the inputs and outputs of organic matter from the system, the history of land use, and land management; this can determine the rates of change of organic carbon under the soil when vegetation and management practices have changed, as in the case of forest clearing to establish pastures.
In this context, SAF´s help to prevent the depletion of existing natural carbon stocks or storage. Furthermore, if higher concentrations of COS are achieved, they increase the amount of biomass returned to the soil, strengthen the stabilization of organic matter, and conserve animal and plant biodiversity, helping to avoid the depletion of existing natural resources, in practices such as pruning and incorporation of system residues into the soil (Lorenz and Lal, 2015).
Potato crop (S. tuberosum) also shows a high content of carbon stored in the soil of 78.84tC.ha-1 (Figure 1). This value is in line with studies conducted by Paz et al. (2012), who found that in andisol soils potato crops stored higher carbon content (78 to 144tC.ha-1) compared to maize crop (45 to 128tC.ha-1)
According to Verhulst et al. (2015) crop rotation combined with intensified production can generate an increase in soil carbon, due to the change in the quality of the harvest residues input. This is reflected in areas where potato crops(S. tuberosum) are managed in rotation with pastures, maintaining two cycles for potato crops and two to three years for pastures, which effect can be seen in the high carbon content of this land use system.
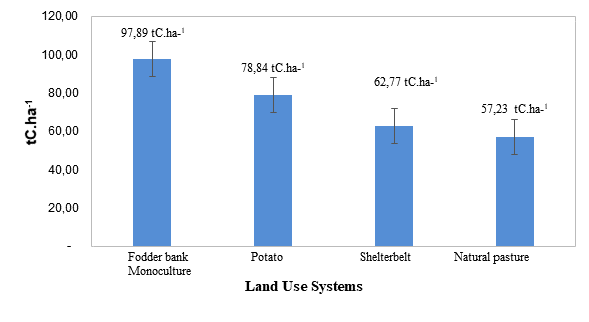
Figure 1. Carbon stored in different land uses (fodder bank, potato monoculture, shelterbelt and natural pasture).
In a shelterbelt with alder (A. acuminata); values of 62.77tC.ha-1 were obtained (Figure 1); being higher than those found by León et al. (2012) in the same arrangement with native and introduced species with values between 1.13tC.ha-1 and 1.73tC.ha-1. This is possibly due to the type of species established, age, and management of the system. Likewise, the values found in this study do not agree with those reported by Burbano et al. (2009), in a silvopastoral system with alder (Alnus jorullensis H.B.K), alfalfa (Medicago sativa L.) and white clover (Trifolium repens L.), reporting greater quantities of carbon stored in the soil ranging from 93.96tC.ha- 1 to 153,495tC.ha-1. It is necessary to consider, that the rates of carbon storage depend on the age, the density of plants, type of established species, irrigation, fertilization, type of soil, characteristics of the site (climatic factors), and the silvicultural management (Orozco et al., 2014).
Concerning the amount of carbon found in natural pastures, which was 57.23tC.ha-1 (Figure 1), it is consistent with those reported by Giraldo et al. (2008) in pasture-only areas, where amounts of 54tC.ha-1 of carbon stored in the soil were obtained.
In contrast, this study does not agree with Salinas and Hernández, (2007) who state that when the soil is used as pasture, increases in organic carbon can be perceived due to the high density of roots in the superficial layers of the soil, which are of greater reserve in interaction with the environment and the use of the soil with the amount of organic carbon.
Likewise, research carried out by Céspedes et al. (2012) do not coincide with the present study since they found that in the yellow grass (Sorghastrum setosum Griseb) and the meadow (Cynodon nlemfluencis), quantities of carbon were found between 10.5tC ha-1 and 19.5tC ha-1.
This is possibly due to certain factors that influence the organic carbon content of the soil as stated by Ibrahim et al. (2007), which are the history of land use, the biological and physical conditions of the soil, and the history of organic material input that help to determine the rates of change of carbon under the soil when vegetation and management practices have changed, such as deforestation, tillage and land use.
Carbon stored in the soil at different depths (30 and 60cm). In the analysis of the different depths evaluated (30 and 60cm) significant statistical differences were found (Table 2). The table below shows the values concerning the carbon stored at two (2) depths (30 and 60cm) in the different land uses (Table 3).
In this sense, it was determined that the carbon contents in the soil were greater at a depth of 60cm with an average of 90.73 tC.ha-1 while, at a depth of 30cm, a lower amount was attained with 57.63 tC-ha-1 (Figure 2).
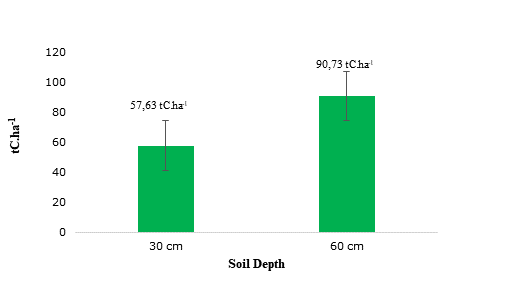
In comparison with other land uses, these results are consistent with Burbano et al. (2009) who states that the silvopastoral system in scattered trees, the alder (Alnus jorullensis H.B.K.) accumulates more carbon at depths of 45cm (153.49tC.ha-1) decreasing to 30cm (93.96 tC.ha-1).
Figure 2. Carbon stored in the soil at two depths (30 and 60cm).
These results do not coincide with that reported by Delgado et al. (2016) in a silvopastoral system in the pasture in alleyfarming with wax laurel (Morella pubescens (Willd.) Wilbur), in San Pablo, Nariño, who found greater amounts of C at 0 - 15cm depth (4.2t.ha-1), decreasing progressively at depths of 15 - 30 and 30 - 45cm.
On the other hand, in studies carried out by Fernández et al. (2019) in a pine plantation and native vegetation of Páramo de Rabanal in Boyacá, Colombia, values of 51.4tC.ha-1 and 108tC.ha-1 were reported at depths of 0 - 15cm and 15 - 30cm, indicating that at the shallowest depth a lower value was reported compared to this study with 57.63tC.ha-1 at 30cm and at the greatest depth, a higher value was reported compared to 60cm depth with 90.73tC.ha-1, respectively.
This also contrasts with studies by Carvajal et al, (2009) in Andean landscapes, with volcanic soils, where they found that the depth of the soil influenced the contents of carbon stored in the surface layer and it decreased towards the lower layers, presenting C contents between the depths of 0 to 10cm and 20 to 30cm with 5.8tC ha-1 in the upper zone, 10.4 tC ha-1 in the middle zone and 3 tC ha-1 in the lower zone of the region; with highly significant differences (p<0.05).
The present study differs from Lok et al. (2013), where the largest carbon deposit was found in soils at depths of 0 to 15cm in three tropical livestock systems in exploitation (silvopastoral based on Panicum maximum and Leucaena leucocephala, the monoculture of Panicum maximum and association of grasses with a mixture of creeping legumes). Thus, it can be concluded that the contents of carbon stored in the soil are a function of the use and management of the soil and directly related to the content of organic matter.
In general, the stored carbon values found in this study, with ranges between 63.32 and 54.45tC-ha-1, were much lower than those obtained by Alvarado et al. (2013) in the first 30 cm of depth in andisol soils (114tC-ha-1). This could be indicating that soils have been inadequately managed in past years, which has caused losses of organic carbon in the soil (COS) and possible CO2 emissions to the atmosphere.
For this research, the depth was a determining factor in the accumulation of carbon in the soil, obtaining the greatest amount from 30 to 60cm. This is pointed out by Gutiérrez (2015), who states that carbon storage increases as the soil profile get deeper, due to the radical depth and the extraction or removal of carbon in more superficial layers due to natural and anthropic phenomena, where the differences that can occur concerning the quantities of stored carbon, can vary from one place to another.
Activities such as tillage, pruning, logging, fertilizer application generate a direct intervention in the dynamics of the COS through the vegetation cover, changes in use and management practices; for example, the intensive use of the plow, shortens the "life cycle" of a macro-aggregate, causing a decrease in the formation of new micro-aggregates and therefore the capture of carbon within them, promoting the release of this element into the atmosphere, while conservationist use favors its accumulation in organic forms in the soil (Martínez et al., 2008). Therefore, the lower carbon content found at a depth of 30cm is not necessarily due to a natural condition, but rather to work that increases or decreases these values that can affect the content of the sink.
According to the results obtained, at the depth of 60cm, the soil use that stored the most carbon was the fodder bank with 139 tC.ha-1, followed by potato monoculture with 94.36 tC.ha-1, the live fence with 68.7 tC.ha-1, as opposed to natural pasture which presented a lower amount with 60.02 tC.ha-1 (Figure 3).
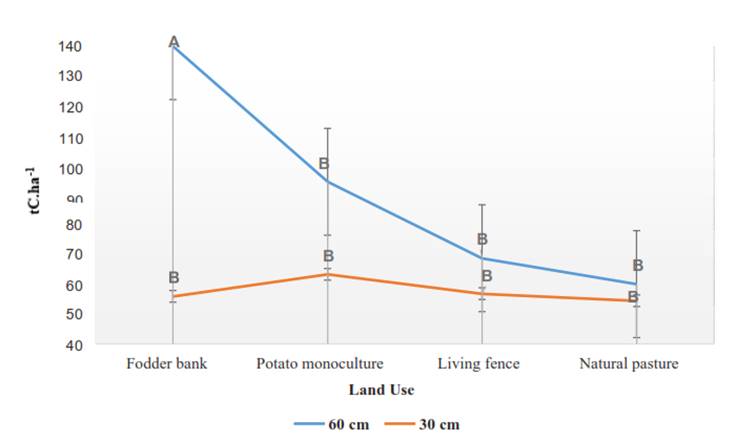
Figure 3. Carbon stored in soil use interaction by depth. Different letters indicate significant differences (p<0.05) and means with the same letter are not significantly different (p>0.05).
On the other hand, at a depth of 30 cm it was found that the soil use that presented the highest carbon content was potato crop (S. tuberosum) with 63.32tC.ha-1, live fence with 56.84tC.ha-1), fodder bank with 55.94tC.ha-1, natural pasture also reported lower values with 54.45tC.ha-1 (Figure 3).
CONCLUSIONS
The soil uses showed statistical differences in carbon storage, with the fodder bank storing the most carbon in the soil, unlike natural pasture, which had less carbon storage.
In all land uses, soil depth of 60cm showed greater carbon storage compared to a depth of 30cm.
In the interaction between soil use and depth, no significant statistical differences were found at 30cm; however, at 60cm the fodder bank did present a significant difference concerning natural pasture.