INTRODUCTION
Climate change is reflected in the increase of mean temperature of the planet, which rose close to 1ºC over the last century. This has caused new patterns of winds, marine currents, rains, and droughts, which also result in the proliferation of disease vectors, the appearance of new diseases, and impacts on ecosystem services (ES) (Rogelj et al., 2019). ES are complex and dynamic natural mechanisms that guarantee the availability of raw materials, energy, elements, and their cycling, to satisfy the needs and conditions for the development of various life forms (Balvanera et al., 2017). Among the most important regulatory ES is carbon capture, which is defined as the absorption of CO2 from the atmosphere and subsequent storage in biomass, necromass, or soil (Oliva et al., 2017).
Native forests, which are considered strategic ecosystems for the world (Vicuña et al., 2018), represent 52.6% of the continental surface of Colombia (DANE et al., 2015). Agricultural systems generate serious impacts on the quality of the ES. These are a result of deforestation and degradation due to the introduction of pastures for extensive cattle ranching and the change to other land uses (Lavelle et al., 2016; Ribeiro et al., 2020). This explains how livestock contributes 7.1 Pg CO2e/year, which represents 14.5% of total global emissions (Rojas-Downing et al., 2017). Deforestation and the consequent expansion of the agricultural frontier contribute to reducing forest capacity to store carbon (Segura-Madrigal et al., 2019; Mena-Mosquera et al., 2020; Segura-Madrigal et al., 2020). In Colombia, 42.3 million hectares are destined for agricultural use: 80% for grasslands and 20% for crops (DANE et al., 2015).
The most updated green-house gas (GHG) inventory for Colombia was registered in 2012, with 258 Mt CO2 (Yáñez et al., 2020). The total above-ground biomass in the natural forests of Colombia in 2012 contained about 7.1Pg C, with an average of 121.9Mg ha-1 (Álvarez et al., 2012; Phillips et al., 2016). The Colombian Amazon has an area of 30 million ha with an estimated mean above-ground biomass of 254.5Mg ha-1, equivalent to 3.8Pg CS. About 83% of the Amazon is covered by forests; around 8% are pastures, while the crops dedicated to the production of food, fiber, and raw materials cover less than 0.3% (SIAT-AC, 2018). The 65.5% of the national deforestation is concentrated in the Amazon region (IDEAM and MinAmbiente, 2020). Caquetá has 63.472 km2 of forest area (Murcia et al., 2014), which are being lost at a rate of 145,000 ha yr-1 mainly by timber extraction, mining and the agricultural (Pardo et al., 2016).
This research aimed to estimate the carbon stored in above-ground biomass of forests, rubber plantations (Hevea brasiliensis Muell Arg.), and trees in pastures in the Colombian Amazon piedmont and their effect on the potential land use changes. The results provide an important estimation of the current carbon storage at a landscape scale, CO2 emissions or capture, with potential land use changes and reduction of CO2 emissions if deforestation is totally controlled. The study provides information for planning and making decisions about land use and environmental policies that lead to sustainable development.
MATERIALS AND METHODS
Study area. The research was developed in the rural area of Belén de los Andaquíes at 1º20'93.3”- 1º12'8.9”N and 75º50'30”- 75º44'21.5”W, in the Amazon piedmont of Caquetá (Colombia). This area presents an undulating topography between 250 and 530m of altitude, 3758mm of annual precipitation, average temperature of 25.8oC, and 4.3 h day-1 of solar brightness (IDEAM et al., 2018).
Sampling design. A total of 174 temporal sampling plots of 250 m2 each were established following recommendations by Andrade and Segura (2017): 80, 40, and 54 in forests, rubber plantations and pastures with trees respectively. All trees with trunk diameter at breast height (dbh) ≥ 10 cm, located in the plots, were measured and taxonomically identified, according to Gentry (1996). A semi-structured survey about the land uses distribution and the crops grown was carried out to the farm owners.
Estimation of biomass and carbon. In forests and pastures, the allometric model of Álvarez et al. (2012) developed for the humid tropical forests of Colombia was used and a wood density of 0.6 g cm-3 was considered, as recommended by IPCC (2006) and Álvarez et al. (2012) (Equation 1). The above-ground biomass of rubber trees was estimated with the local allometric model developed by Hernández (2014) in Caquetá (Equation 2). The use of these allometric models ensure good estimates because they were developed in the same zone or life zone.
Where, Ba: Above-ground (kg tree-1); DBH: Diameter of the trunk at breast height (cm); ρ: Density of the wood (g cm-3)
Where, Ba: Above-ground (kg tree-1); dbh: Diameter of the trunk at breast height (cm).
The carbon stored was estimated by multiplying the biomass by 0.47 and transformed to CO2 using a stoichiometric factor of 3.67 (Marín et al., 2016; Andrade and Segura, 2017). The reduction in emissions from deforestation stop was estimated for 20 years (2021 to 2041) (Equation 3), proposed by Segura-Madrigal et al. (2019), using a deforestation rate of 0.41% year-1, estimated by Torrijos (2020) between 2016 and 2017 in Caquetá, and an initial area of forests of 72032.3 ha (Alcaldía Municipal de Belén de los Andaquíes, 2016).
Where, RE: Reduction of GHG emissions (Mg CO2e year-1); CS: carbon storage (Mg C ha-1); TA: Total area of natural forest (ha); DR: Deforestation rate (% year-1).
The impact of potential land use changes on carbon in above-ground biomass was simulated, thus identifying additionality of carbon capture (mitigation) or CO2 emission. This variable was estimated per unit of area and for the total area of each of the land uses: 72032 ha in forests (dense, intervened and gallery), 39903 ha of pastures with trees and 745 ha of rubber plantations (Alcaldía Municipal de Belén de los Andaquíes, 2016).
RESULTS AND DISCUSSION
Land uses. The 40 farms in the sample had a total area of 1823.5 ha, whose land uses were discriminated by cover (Table 1).
Table 1. Land use and total area of 40 farms used for the evaluation of carbon storage in trees of the Amazon piedmont, in Belén de los Andaquíes (Colombia) in 2018.
Coverage | Number of Farms | Total area of the 40 farms in ha (%) | Total area of sampling plots in ha (#) |
---|---|---|---|
Forests | 40 | 242 (13,3) | 2.0 (40) |
Pastures | 27 | 955.2 (52,4) | 1.3 (54) |
Crops* | 40 | 275 (15,0) | N/A (0) |
Rubber plantations | 20 | 100 (5,5) | 1.0 (40) |
Fallows, other uses | 251.3 (13,8) | ||
Total | 1.823,5 (100,0) | 4.3 (40) |
*Includes area of the rubber plantations (H. brasiliensis Muell Arg.). N.A.: not applicable.
The plantations of H. brasiliensis and African palm (Elaeis guineensis Jacq.) stand out with about 100 and 25 ha in the study area, respectively. The main agricultural activities were cocoa (Theobroma cacao L.), cassava (Manihot esculenta Crantz), banana (Musa AAB), sugar cane (Saccharum officinarum L.), and fruit trees such as arazá (Eugenia stipitata Mc Vaugh), copoazú (Theobroma grandiflorum (Willd. ex Spreng.) K. Schum), pineapple (Ananas comosus (L.) Merr.), and borojó (Borojoa patinoi Cuatrecasas).
Main woody perennial species. Several of the woody perennial species found are native and have a specific function for these ecosystems. Other species have been introduced for economic purposes. Some of these species present some degree of threat (Table 2). Fabaceae was the family with the greatest diversity of species (Table 2).
Table 2. Floristic identification of the forest in rural production systems of the Amazonian piedmont, Belén de los Andaquíes (Caquetá, Colombia).
Common Name | Species | Family | |
---|---|---|---|
Palma guajo* | Bactris simplicifrons Mart. | Arecaceae | |
Abarco** | Cariniana pyriformis Miers. | Lecythidaceae | |
Comino** | Aniba perutilis Hemsl. | Lauraceae | |
Cedro*** | Cedrela odorata L. | Meliaceae | |
Roble**** | Quercus humboldtii Bonpl. | Fagaceae | |
Achapo | Cedrelinga cateniformis (Ducke) Ducke. | ||
Carbón | Zygia cataractae (Kunth) L. Rico. | ||
Guamo | Inga marginata Kunth. | Fabaceae | |
Granadillo | Platymiscium pinnatum (Jacq.) Dugand. | ||
Guamo colorado | Macrolobium acaciifolium (Benth.) Benth. | ||
Guamo piedra | Inga edulis Mart. | ||
Ahumado | Vitex excelsa Moldenke. | ||
Amarillo real | Ocotea argyrophylla Ducke. | Lamiaceae | |
Laurel comino | Ocotea sp. | ||
Tehobroma de monte | Theobroma glaucum H. Karst. | ||
Balso | Ochroma pyramidale (Cav. ex Lam.) Urb. | Malvaceae |
*: Threatened plant in LC (least concern); **: plant threatened in CR (critical danger); ***: plant threatened in EN (danger); ****: plant threatened in VU (vulnerable).
Some important timber species were found in the forest cover of the farms. This is the case of oak (Quercus humboldtii Bonpl.), colombian mahogany (Cariniana pyriformis Miers), comino tree (Aniba perutilis Hemsl), cedar (Cedrela odorata L.), and guajo palm (Bactris simplicifrons Mart). Cárdenas and Salinas (2006) and Pardo-Rozo et al. (2020) argued that these species are within the threat categories, according to the International Union for Conservation of Nature (IUCN).
Dasometric variables and carbon storage. The forests had the highest tree abundance followed by rubber plantations (859 vs 588 individuals ha-1, respectively). Rubber plantations have a pre-stablished distance between trees, while the trees in the forest grow freely. The lowest tree abundance in the pastures could be due to the livestock systems management used in the region, where only some scattered trees are left standing to provide shade (Álvarez et al., 2020). The estimated CS in the forests was, on average, 15.2 Mg ha-1 greater than in rubber plantations (Table 3); however, the CS in each rubber tree was 34.2% higher than in the tree of the forests, due to its greater size. The greater abundance of trees in the forests explains their greater CS.
Table 3. Dendrometric measurements and above-ground biomass and carbon in the main land use systems in the Amazon piedmont, Belén de los Andaquíes (Caquetá, Colombia) in 2018.
Variable | Forests | Pastures with trees | Rubber Plantations |
---|---|---|---|
dbh (cm) | 20.5 (0.4) | 15.5 (0.9) | 25.8 (0.8) |
Ba (Mg individuo-1) | 0.38 (0.03) | 0.30 (0.06) | 0.51 (0.04) |
Ba (Mg ha-1) | 327.9 (9.1) | 3.0 (0.3) | 295.4 (20.4) |
Cs (Mg ha-1) | 154.1 (9.1) | 1.4 (0.05) | 138.9 (9.5) |
CO2 (Mg ha-1) | 565.1 (9.1) | 5.2 (0.19) | 509.1 (34.8) |
Abundance (individuals ha-1) | 859 (37) | 10 (1.0) | 588 (0.0) |
dbh: diameter of trunk at breast height; Ba: above-ground biomass; Cs: carbon storage; Values correspond to the mean and the standard error in parentheses.
The mean carbon storage for the forests in this study was 154.1 Mg C ha-1, which significantly higher than the reports by Álvarez et al. (2012) for the forests of Colombia (67.7 Mg C ha-1) but much lower than the 288.4 Mg C ha-1 in tropical forests of the Colombian Amazon estimated by Phillips et al. (2016). Similarly, the mean carbon storage was also lower than 249.0 Mg C ha-1 reported in the Amazon piedmont, according to Rojas-Vargas et al. (2019). These results were also higher than those estimated in tropical forests of the Amazon by IPCC (2006) and Brown et al. (2005) with 141.0 and 126.0 Mg C ha-1, respectively. However, the estimates of this study are within the range of 48.1 and 147.5 Mg C ha-1, reported by Phillips et al. (2016) for the Colombian Amazon.
Although forests have a much higher density of carbon in above-ground biomass than pastures with trees, the latter are important carbon sinks because of the magnitude of their extension. According to Oliva et al. (2017) and Álvarez et al. (2020), changes in pastures can alter the global carbon cycle, and thus, it would be necessary to study how to enhance these efforts towards ecosystem service in the Amazon piedmont. The tree abundance in pastures with trees in this study was 10 trees ha-1, which was extremely close to 9 trees ha-1 reported in Trujillo et al. (2012) for farms in the same zone and 5-9 trees ha-1 for pastures in Ecuadorian Amazon (Congo et al., 2018); however, this contrasts with the 17 trees ha-1 estimated by Rojas-Vargas et al. (2019) for traditional pastures in the same area.
The results of carbon in the present study were slightly higher than reports of Rojas-Vargas et al. (2019) and Rico et al. (2016) in the Caquetá Amazon (0.69 and 0.82 Mg C ha-1, respectively). Trujillo et al. (2012)mentioned that trees can be established in pastures as a strategy to mitigate GHG emissions in livestock systems.
Carbon storage in rubber plantations (H. brasiliensis Muell Arg.) was close to the 146.2 Mg C ha-1 reported by Hernández (2014) but higher than that estimated by Durán et al. (2011), Orjuela et al. (2014), and Moreno et al. (2005) with 6.9, 57.2, and 58.4 Mg CS ha-1, respectively, in Colombian Amazon. However, the implementation of rubber agroforestry systems with copoazú, and cocoa or with Amazonian fruit trees could increase carbon sequestration and generate other ES, such as soil improvement, increased productivity, and adaptation to climate change and those derived from biodiversity conservation (Rosas et al., 2016; Marín et al., 2016; Sterling et al., 2019).
Only 81.7% of the farms studied has productive pastures for livestock and crops: 376.4 ha has in natural regeneration or fallows and thus, could accumulate a significant amount of carbon when transitioning to secondary forests (Vicuña et al., 2018), and 298 ha in degraded pastures could be recovered. According to Liebig et al. (2017), pastures and other covers can be used to increase natural carbon deposits in addition to a possible response to local environmental management policy. Additionally, Soussana et al. (2010) and Rotz et al. (2020) also suggested that pastures could increase soil organic carbon, which generate other environmental benefits, such as improvements in water retention and erosion, and it would improve the nutrient cycle.
Reduced emissions (RE). It was estimated that the initial 72032.3 ha of forests stored 11.1 Tg C in above-ground biomass, and if deforestation stopped immediately, the emissions avoided would reach 3.2 Tg of CO2 over the next 20 years (2021 to 2041) (Figure 1).
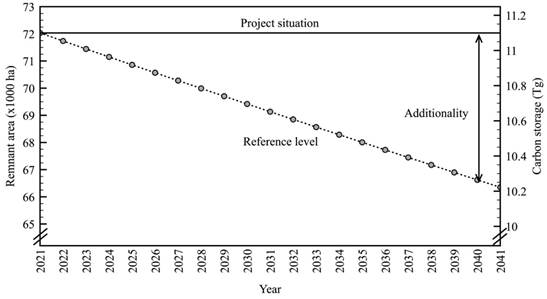
Figure 1. Additional carbon storage in above-ground biomass in 20 years under the scenario of a constant deforestation rate of 0.41% year-1 in Belén de los Andaquíes, Caquetá, Colombia. The additionality arrow indicates the reduced CO2 emissions if deforestation were eliminated in the 20-year simulation.
The main changes in carbon sequestration are observed when areas of pastures with trees are converted to forests, which implies a gain or additional carbon sequestration of 560 Mg CO2 ha-1 (Figure 2). In the same way, converting pasture with trees to rubber plantations would also have positive effects, presenting an addition of 505 Mg CO2 ha-1 (Figure 2). At the landscape level, the most favorable change would be to convert the entire pasture with trees into forests, which would entail an additional carbon capture of 22 Tg CO2. The least convenient situation, in terms of carbon balance, would be the transition from the natural forest to pastures with trees where 40 Tg of CO2 would be lost. Rubber plantations are seen as an economically viable and environmentally favorable opportunity as a C sink, representing a transition from pasture to forests (Figure 2).
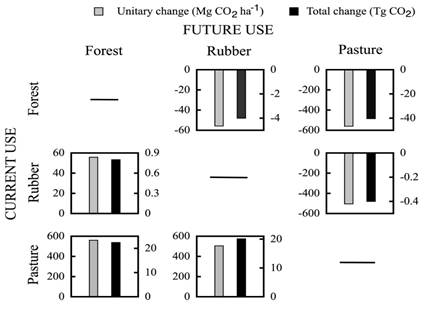
Figure 2. Impact of land use changes on carbon storage in above-ground biomass in Belén de los Andaquíes, Caquetá, Colombia.
In the rubber plantations, an accumulation of 103.5 Gg of carbon was estimated in the 745 ha and close to 55.9 Gg in the 39903 ha of area pastures. The mean carbon storage in forests was 154.1 Mg ha-1, and this indicates that when 1 ha of forest is deforested in the Colombian Amazon piedmont, about 565.5 Mg CO2 are emitted.
The reduced emissions by stopping deforestation in the area would add up to about 160.7 Gg CO2 per year, even though the deforestation of the study sample is much lower than that of Caquetá (Colombia).
The addition of such efforts is important to slow down GHG emissions. The global objective against climate change mitigation could be achieved if the forest systems increase their GHG sinks capacity or at least maintain their current levels of carbon storage. This action requires stopping the loss of primary forests, creating rural production systems with less impact on the forests, soil, and water, and both monitoring and controlling land use (Carvajal and Andrade, 2020; Sousa et al., 2018).
Payments for Environmental Services (PES) is one of the environmental policy instruments to encourage conservation, reforestation, and/or avoid deforestation (Decreto 1007 del 2018). In the case of a potential establishment of a PES program to stop deforestation in the study area, a reduction in emissions of 3.2 Tg of CO2 could be achieved, which is equivalent to 16% of the current emission of Caquetá (IDEAM et al., 2018). In turn, a positive outcome of the policy establishment would be to guarantee other associated environmental services (Börner et al., 2017), such as water and microclimatic regulation.
The impact analysis of land use on carbon storage suggest that the value of environmental services could be incorporated into the land market through mechanisms such as PES (Pardo et al., 2016). This would improve land use conflicts by encouraging forest conservation, since Caquetá presents an overuse of agricultural land. Livestock occupied 100% of the soils suitable for this activity and the additional overcrowding of 13% of soils that were used for agricultural and forestry activities in about 1'192,000 ha (UPRA, 2018).
CONCLUSIONS
Carbon capture in above-ground biomass is an important ecosystem service to climate change, but at the same time it is vulnerable to land use changes attributed to agricultural activities in the Amazon piedmont. The forests presented the highest carbon storage, followed by rubber plantations. The most convenient hypothetical conversion scenario for carbon storage is the transition from pastures to forests or rubber plantations, which increase carbon capture in 560 y 505 Mg CO2e ha-1 respectively. On the other hand, the worst scenario for the ecosystem service would be the conversion of forests to pastures, which causes an emission of 40 Tg CO2e ha-1. The rubber (H. brasiliensis Muell Arg.) plantation is an alternative to enhance carbon sinks since it fixes more carbon than pastures with trees.