INTRODUCTION
Food-borne diseases (FBD) are a major public health problem worldwide, causing approximately 600 million diseases and 420,000 deaths per year (Mendonca et al., 2020). In general, all the food consumed is considered a potential vehicle for FBD. Pollution originates from different foci distributed in the environment, such as earth, dust, water, insects, and feces of birds, reptiles, and mammals, and they can affect food production, processing and preparation processes, so FBD reaches the final consumer through fruits, vegetables, meat, fish, sausages, ready-to-eat food, milk, and its derivatives. When contaminated food enters the gastrointestinal tract, there are clinical symptoms such as fever, fatigue, nausea, vomiting, abdominal cramps, diarrhea, joint aches, and backaches. In pregnant women, children, and elderly people, symptoms increase in severity (Espinosa-Mata et al., 2021).
Food-borne diseases are closely related to biological factors and are characterized by saprophytic or ubiquitous microorganisms. The bacteria involved are often Li. monocytogenes, Escherichia coli, Salmonella, and Staphylococcus aureus, which have demonstrated and developed the ability to survive and grow under various conditions and food matrices (Maggio et al., 2021). One of the pathogenic bacteria of concern in food safety and harmlessness is Li. monocytogenes, a Gram-positive bacterium responsible for human listeriosis, which is widely distributed in the environment. It can survive in dry environments and shows stability at low storage temperatures and resistance to pasteurization processes (Taylor & Zhu, 2021).
Advances in biotechnology have demonstrated the efficiency of Lactic Acid Bacteria to biologically control pathogenic strains responsible for FBD, including Li. monocytogenes (Zhao et al., 2020). The Food and Agriculture Organization (FAO) and the World Health Organization (WHO) list these bacteria as probiotics, which are described as living microorganisms that, when administered in adequate quantities, confer a benefit to the health of the host (El-Enshasy & Yang, 2021). Probiotics have been widely used for the production of fermented food and other food matrices such as fruits, different types of juice, meat, sausages, fish, and freeze-dried and functional food (Hutkins, 2019).
The probiotics of Lactobacillus genera have the ability to regulate intestinal microbiota, stimulate the immune system, control pathogenic bacteria, improve zootechnical parameters in animals, hydrolyze proteins, and preserve food through antimicrobials, antioxidants, peptides, lactic acid, exopolysaccharides, and other metabolic end products resulting from fermentation (Hutkins, 2019; Thatoi et al., 2020). However, its application is limited by different adverse conditions (environmental, gastric, enzymatic, pH, and industrial processes), which affect the viability and survival of Lactic Acid Bacteria (LAB). Microencapsulation is an alternative that is based on the formation of a physical barrier (packaging and sealing) that offers protection to microorganisms. This technique seeks the minimum concentration necessary for microorganisms to express their probiotic qualities (El-Enshasy & Yang, 2021).
In microencapsulation, matrices or encapsulant materials are used in order to improve storage quality and protection against the various factors that may affect the viability of the LAB (Marefati et al., 2021). They must be compatible with probiotics and be categorized as Generally Recognized As Safe (GRAS) (Hutkins, 2019). These components are called prebiotics, and several studies have shown the health advantages of their consumption combined with probiotics (El-Enshasy & Yang, 2021). The aim of this study was to determine the effect of microencapsulation by spray drying technique on the probiotic viability of La. reuteri ATCC on Li. monocytogenes ATCC 35752 under simulated gastric conditions.
MATERIALS AND METHODS
This research was carried out in the PROBIOTEC-FORAPIS Research Group laboratory located in the teaching laboratory block and in the specialized laboratories of the University of Nariño in the city of Pasto, located in the department of Nariño, Colombia. La. reuteri and Li. monocytogenes reconstitution, sowing, and adjustment of inoculum were obtained through the statements by Jurado-Gámez et al. (2014). Once the La. reuteri strain was reconstituted, the method described by Montes (2013) and Rodríguez et al. (2016) was used to microencapsulate, and an inoculum of 500 ml was prepared at 5 %p/v (25 g of Maltodextrin and 25 g of Inulin at 450 ml).
The spray drying equipment Dryer Spray Bilon 6000s® was used at an inlet temperature of 160°C and an outlet temperature in a range of 65-67°C, with a complete cycle of 3 hours and 30 minutes. The microencapsulated material was packed in metallized ziploc containers, previously sterilized, and stored at room temperature (19±2°C) for 90 days. The variables viability, efficiency, humidity, water activity, solubility, wettability, morphology, and particle size were evaluated for the study and characterization of the microencapsulated material. These parameters were determined at 80 days of storage using the methods described by Montes (2013) and Rodríguez et al. (2016).
After the storage period of the microencapsulated material, the fermentation kinetics of La. reuteri was performed in MRS and PRO medium (White sugar 10g/L; soy milk 15g/L; powdered milk 150g/L; inulin 15 g/L). Samples were taken at 3-hour intervals to determine viable microorganisms in CFU/ml (Santander, 2021), sugar consumed (mg/L) (Dubois et al., 1956), protein produced (mg/L) (Lowry et al., 1951), pH and lactic acid percentage (%). In addition, specific growth rate, cell duplication time, generation time, and maximum harvest were obtained. Finally, the peptide and amino acid content were determined by HPLC-DAD of a sample of La. reuteri supernatant.
The production of exopolysaccharides (EPS) was determined using the methodology described by Zhang et al. ( 2020) , which was modified by the authors at 28°C, 35°C, and 42°C temperatures for seven days, 48 hours and 24 hours, respectively. The growth of La. reuteri at different temperature levels was determined according to what was proposed by Cai et al. (1999), at 37 °C and 45 °C, during 24 hours in MRS agar. Finally, a plate count was performed. The exposure of microencapsulated La. reuteri to simulated gastric conditions was carried out according to what was mentioned by Brodkorb et al. (2019) .
To achieve this, 2g of microencapsulated were taken and added to 18g of distilled water. Then, they were subjected to lysozyme activity (0.01%) at 37°C/10min in constant agitation (85rpm). After that, 3% pepsin and 0.5% NaCl were added by adjusting the pH to 2.0 with HCl 5M, and it was kept at 37°C/90min in constant agitation (60rpm). Subsequently, 1% pancreatin, 0.3% bile, and 0.5% NaCl were added by adjusting the pH to 6.8 with NaOH 1.5 M, and it was maintained at 37°C/150min in constant agitation (60rpm). Finally, it was inoculated onto Petri dishes for 48 hours at 37°C, and a plate count of Colony Forming Units (CFU) was performed.
The antibiogram of La. reuteri and Li. monocytogenes was performed under the methodology of Bauer et al. (1966) by using the antibiotics amoxicillin (AMC 30µg), cefquinome (CEQ 30µg), penicillin (P 10µg), gentamicin (GN 10µg) and tetracycline (TE 30µg). The inhibitory effect of La. reuteri on Li. monocytogenes was evaluated using the methodology described by Jurado-Gámez et al. (2013). Four methods were evaluated: the first one was impregnated agar discs; the second one was the Pads method with supernatant; the third one was diffusion in plastic cylinder with supernatant; and the last one was diffusion in plastic cylinder double layer with supernatant. They underwent the following conditions: pH 6, filtrate (F), unfiltered (SF), heat treatment at 80°C for 10 minutes (80°C), and without heat treatment.
For the evaluation of the physicochemical variables of microencapsulation, gastric simulation, antibiogram and inhibition, descriptive statistics were used at a significance level of 5%. For the fermentation kinetics, five samples were taken for each parameter every 3 hours, and a design of measures repeated in time was used with two treatments, medium MRS and medium PRO, as fixed factors and time as a random factor. It was carried out at a level of significance of 5%. The results were organized in an Excel® sheet, and the analyzes were carried out in the statistical software (4.0.0, 2018).
RESULTS AND DISCUSSION
The results of the microencapsulation of La. reuteri using the spray drying technique in a binary matrix composed of inulin and maltodextrin, packed in a metal ziplock container, and stored for a period of 90 days at room temperature (19±2°C) are described below. Morphological characterization by scanning electron microscopy allowed to observe circular, agglomerated, and individual microcapsules with sizes ranging from 2.42 µm to 9.33 µm (Figure 1).
The following results were obtained with the study and characterization of microencapsulation: Viability 100%; efficiency 89.76%; solubility 99.10%; wettability 153 seconds, and humidity 4.4%. Water activity was evaluated three times: 45 days, 70 days, and 90 days with values of 0.639, 0.665, and 0.670, respectively. The results show an increase in water activity between 45 and 70 days, which stabilizes at 90 days (p<0.05).
Authors studied microencapsulation of L. plantarum and obtained values for viability of 83.3%; efficiency of 88.4%; humidity of 7.97% and 5.23%; water activity of 0.4%; wettability of 1 min with 56 seconds; solubility of 96%; and microcapsule dimension between 35,68µm and 3.47µm (Sinsajoa-Tepud et al., 2019; Jurado-Gámez et al., 2021).
The conditions of the microencapsulate mentioned above vary in the concentration of encapsulant material, microencapsulation temperatures, and storage periods; however, they are similar values to those obtained in the present investigation, with the exception of the value for water activity, which in the present study is higher. Nevertheless, the microencapsulation presented adequate levels during the storage period, demonstrating a good interaction between the microencapsulated material and lactic bacteria.
Fermentation kinetics of La. reuteri allowed the establishment of the exponential phase in the MRS medium at 12 hours with 22.01Ln CFU/ml (3.6x109 CFU/ml) and in the PRO medium at 18 hours with 28.33 Ln CFU/ml (2.0x1012 CFU/ml). This parameter presented a statistical difference at the mentioned times (p>0.05).
Sugar consumption (Figure 2.a) for hour 12 (exponential phase) in the MRS medium was 8.85 mg/L, close to that obtained in the PRO medium at 18 hours (exponential phase) with a value of 8.78 mg/L without statistical differences for sugar consumption in the two periods mentioned (p>0,05). The production of proteins (Figure 2.b) of La. reuteri in MRS medium (12 hours) and PRO medium (18 hours) is 1.76mg/L and 5.23mg/L, respectively, with statistical difference in the mentioned times (p<0.05).
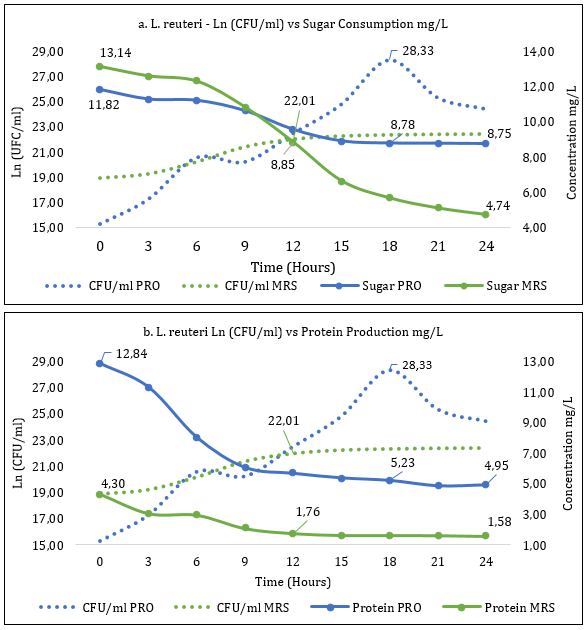
Figure 2 a. Determination of consumption of La. reuteri sugar in PRO and MRS culture media. b. Determination of production of La. reuteri proteins in PRO and MRS culture media.
Data has been reported for protein production of L. gasseri strain in MRS and PRO culture media of 0.66 mg/L at 20 hours and 3.12 mg/L at 16 hours (Romero-Benavides et al., 2016), while L. plantarum strain in culture media MRS and PRO has reported protein consumption of 1,61 and 1.47mg/L at 16:00 (Jurado-Gámez et al., 2016). On the other hand, the consumption of sugar for L. gasseri in MRS and PRO media in the exponential phase with 1.79 mg/L (20 hours) and 2.043 mg/L (16 hours), respectively (Romero-Benavides et al., 2016), and the bacterium L. plantarum reported a sugar consumption of 6,98mg/L in the exponential phase (11:50 hours) in culture medium MRS (Fajardo-Argoti et al., 2021).
The determination of pH and acidity in the exponential phase for each culture medium corresponds to 4,37 pH and 1,01 lactic acid in % for the culture medium MRS; moreover, in the PRO culture medium, 3,9 pH and 1,49 lactic acid were obtained with statistical difference in each of the values mentioned between cultivation times and medium (p<0.05) (Figure 3).
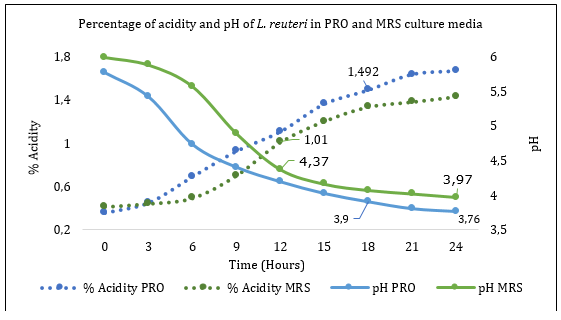
Figure 3 Determination of the percentage of acidity and pH of La. reuteri in PRO and MRS culture media.
Research indicates that in L. plantarum, the percentage of lactic acid evaluated in MRS medium ranges from 0.17% at zero-hour to 0.41% at 24-hours (Sinsajoa-Tepud et al., 2019). These results are far from those obtained in the present study. However, it shows that the percentage of lactic acid increases as the bacterial population increases. Similarly, the authors highlight that lactic acid bacteria can tolerate wide pH ranges from 2.0 to 6.0 (Fang, 2020).
Several probiotic effects are attributed to the production of lactic acid (organic acids) and low pH following homofermentative and heterofermentative bacterial metabolism, and, as shown in Figure 3, the increase in the concentration of organic acids is directly related to the decrease in the pH of the medium (Kanauchi, 2019). Finally, the kinetic parameters presented in Table 1 are obtained.
Table 1 Summary evaluation of kinetic parameters of La. reuteri.
Parameter | MRS Medium | PRO Medium | Unit |
Specific Growth Rate | 0.303 | 0.973 | µmax h-1 |
Cell Replication Rate | 2.287 | 0.712 | Hour |
Generations Per Hour | 0.437 | 1.404 | g |
Maximum Harvest | 3.37 | 10.05 | Ln CFU |
Growth values by fermentation kinetics may vary in the same species and by the effect of culture media. Thus, results of cell duplication reported for L. plantarum vary from 14.47 min in MRS medium (Fajardo-Argoti et al., 2021) to 64.38 min (MRS medium) and 48.41 min (PRO medium) (Jurado-Gámez et al., 2016), and 0.98 hours and 42.42 min for L. platarum in MRS and PRO medium (Gúzman-Insuasty et al., 2015). Crespo (2019) determined a specific growth rate of 0.4 for La. reuteri with a time for the exponential phase of 14 hours in the TSB (Tryptic Soy Broth) culture medium. Cultivation at a temperature of 37°C showed a maximum growth rate of 0.4. For the same BAL, different authors record different values; however, some of the studies referenced enunciate values close to those obtained in the PRO medium but lower ones for the MRS medium obtained in the present research.
The kinetic data mentioned above are within acceptable and recommended ranges. Although the MRS medium was considered a specific culture medium for bacteria of the genus Lactobacillus, the parameters evaluated for this medium were lower in several aspects compared to the PRO medium. Cheng et al. (2019) emphasized that the properties of lactic acid bacteria are expressed under sub-optimal growing conditions so that a good carbon source will affect the cell replication rate to reach a high population and synthesize high levels of bacteriocins, and a higher concentration of nitrogen will maximize biocine production and inhibitory activity. It is necessary to mention that the source and nature of each of the nutrients included in the formulation of the MRS medium vary with respect to the PRO medium. Powdered milk and soy milk were used as nitrogen sources in the PRO medium, and inulin, powdered milk, and white sugar were used as carbon sources.
The supernatant of La. reuteri indicated a VAL-TIR-VAL amino acid chain-like peptide with a concentration of 0.47 mg/ml, and the presence of lactic acid in the supernatant was determined by duplicate with values of 11.2g/L and 11.7g/L. Table 2 shows the amino acid composition of La. reuteri and Li. monocytogenes.
Table 2 Identification of amino acids of La. reuteri and Li. monocytogenes.
N° | Amino acids | La. reuteri | Li. monocytogenes |
1 | Aspartic acid | 5.2 | - |
2 | Serine | 8.3 | 7.9 |
3 | Arginine | 7.8 | 8.2 |
4 | Tyrosine | 48.7 | 39.9 |
5 | Valine | 13.2 | - |
The number of peptides and amino acids product of the fermentative bacterial process can be altered by variations in the compounds of proteolytic metabolism, where the type of nutritional needs, intracellular peptidases, and their regulatory mechanisms influence the release to the environment and whose quantitative and qualitative profile have an effect on the characteristics of fermented dairy products (Rosales-Bravo et al., 2020). Some of the important peptide compounds produced by LAB are bacteriocins, thanks to their antimicrobial activity to inhibit the growth of pathogenic microorganisms (Zhao et al., 2020).
The production of exopolysaccharides was positive for the temperatures and times evaluated. Exposure to different temperatures (37 and 40°C) reported bacterial growths of 1.95x1013 CFU/ml and 2.16x1012 CFU/ml respectively. There is also a higher growth at 37°C (p<0.05). The bacterial concentration after subjecting the microencapsulation of La. reuteri to gastrointestinal conditions is presented in Table 3.
Table 3 Bacterial concentration of microencapsulated La. reuteri under simulated gastric conditions.
Conventional continuum | Conventional discontinuous | ||
CFU/ml | Lysozyme - 10 min | Pepsin+NaCl+ HCl 90 min | Pancreatin+bile+bile salts +NaCl+NaOH 150 min |
5.4 x 1011 | 1.5x1012 CFU/ml | 1.24x1012 CFU/ml | 4.98x1011 CFU/ml |
Rojas (2020) mentions that most EPS are secreted or remain weakly attached to the cell wall by electrostatic interactions (ions, hydrogen bonds, or hydrophobic interactions) and mentions the relationship between EPS production, environmental conditions, and EPS production regulation. As reported by Sørensen et al. (2022) , it has been detected that the exopolysaccharides (EPS) of the studied strains have an immunoregulatory effect on macrophages; additionally, it was found that the cytokine profile induced by the EPS depended on the bacteria in question. Therefore, the same authors state that the diversity in the structure of exopolysaccharides produced by BAL can be translated into different inhibition mechanisms against pathogenic bacteria.
According to Crespo (2019), the optimal growth temperature for La. reuteri is 37°C. This is a determination that the author made by evaluating three temperature levels (37, 20 and 4°C) during 24 hours in TSB, similar to what was mentioned by Reuben et al. (2019), for La. reuteri. Thermal treatments are an effective way to control microorganisms, which is why it is important to know the comfort temperatures for bacterial growth of probiotics and minimize CFU/ml losses during industrial processes.
There are multiple variables that can impact the viability and safeguarding of probiotics that are encapsulated and pass through the stomach, including the probiotic strains' acid resistance properties, the materials and concentrations used for encapsulation, the techniques for encapsulation, and the types of polymers that are integrated into the matrix (Kowalska et al., 2022). Sinsajoa-Tepud et al. (2019) evaluated pH (1, 2, and 3), bile salts (0.3% and 1%), and bovine bile (0.5%) against microencapsulated L. plantarum and showed growth in the range of 3x107 to 6.4x109 CFU/ml. The referenced values, as well as the values obtained in the present investigation, exceed the recommended minimum, 1x107 CFU/ml (El-Enshasy & Yang, 2021) (Table 3, Figure 4.a.), for probiotic products after undergoing simulated gastrointestinal tests. In this way, the expression of probiotic characteristics of microencapsulated microorganisms is ensured.
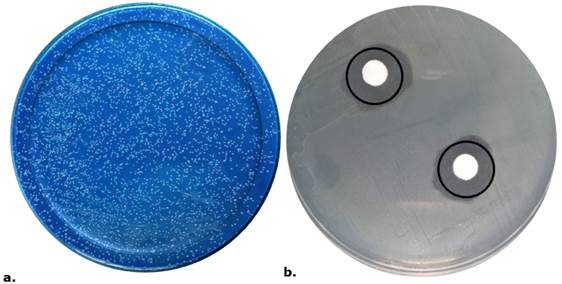
Figure 4 a. Plaque count of La. reuteri colonies after simulated gastrointestinal conditions. b. Inhibition Halo by Pads Method with La. reuteri supernatant versus Li. monocytogenes; 6.7mm.
Using antibiogram, La. reuteri presented resistance to amoxicillin (AMC 30µg), cefquinome (CEQ 30µg), and penicillin (PEN 10µg); Li. monocytogenes expressed resistance to penicillin PEN 10 µg. Moreover, La. reuteri was sensitive to gentamicin (CN 10µg) and tetracycline (TE 30µg), and Li. monocytogenes was sensitive to tetracycline (TE 30 µg) and cefquinome (CEQ 30µg). Popović et al. (2021) submitted a strain of La. reuteri against antibiotics. The lactic bacteria expressed sensitivity against ampicillin and cefalotin, resistance against vancomycin, and intermediate values against amoxicillin and tetracycline. It is claimed that some strains of LAB can act as reservoirs of antimicrobial resistance genes and transfer them to pathogenic bacteria. In this way, LAB can transmit resistance to the target ecosystem or niche through mobile elements (transposons or plasmids).
That is why antibiotic behavior has to be evaluated to determine whether resistance is acquired and expressed by mobile elements, or by chromosomal factors that do not represent risk (May-Torruco et al., 2020). Vázquez-Ortiz et al. (2022) state that, as a general rule, the bacteria of the genus Lactobacillus present intrinsic resistance against the following antibiotics: ciprofloxacin, gentamicin, vancomycin, and trimethoprim. They also mention that several studies show differences in the resistance or sensitivity of different antimicrobials. It is necessary to combine the resistance mechanisms of La. reuteri against antimicrobials to define its range of application and use.
Espinosa-Mata et al. (2021) indicate the antimicrobial sensitivity of Li. monocytogenes isolated from food against the following antibiotics: trimethoprim/sulfamethoxazole, penicillin, ampicillin, erythromycin, and meropenem. Listeriosis caused by Li. monocytogenes is associated with serious complications such as abortion, meningitis, sepsis, and encephalitis, with a high mortality rate. Li. monocytogenes is an optional intracellular pathogen that is widely distributed in the environment and has the ability to thrive in thermal treatments (Taylor & Zhu, 2021). Although Li. monocytogenes still exhibits antibiotic resistance, its distribution in various pollutant factors is decisive in considering its role as an FBD.
The inhibition effect of La. reuteri against Li. monocytogenes reported inhibition halos in different methods. Impregnated agar discs (i), statistical analysis showed that, on average, the concentrations of 75µl and 115µl are similar, and at 95µl, a higher halo was presented (p<0.05) with 1.66 mm, 1.76 mm, and 2.59 mm, respectively. 2) Pads method with supernatant (ii), an increase in inhibition halos was observed as an effect of increasing the concentration of La. reuteri in this way 40µl with 4.93 mm; 75µl with 5.54mm; and 100µl with 6.7 mm (p<0.05) (Figure 4. b.). Similarly, higher inhibition halo of unfiltered pads was found at room temperature (6.14 mm halo) compared to other conditions (temperature and filtering) (p<0.05), but there was no interaction between the two levels (p>0.05).
Diffusion in a plastic cylinder with supernatant (iii), and Diffusion in a double-layer plastic cylinder with supernatant (iv), the results showed similar inhibition halos between concentrations (90µl, 125µl and 150µl) and conditions. No significant differences were observed between the levels evaluated (p>0.05); however, inhibition halos between 2.1 mm and 2.63 mm were recorded for the two methods.
Mejía (2022) defines a halo of inhibition as zones that form around colonies and are measured in mm, which is an indication of biological control of a microorganism. In this study, the antagonistic relationship of La. reuteri to Li. monocytogenes allows for inferring that the concentrations and conditions evaluated have an inhibitory effect on the pathogenic bacteria. La. reuteri is a heterofermentative LAB with the ability to grow under anaerobic conditions and colonize the gastrointestinal tract of the host with many antibacterial properties that result from the production of substances such as organic acids, ethanol, and reuterin (El-Enshasy & Yang, 2021). It inhibits the growth of Gram-positive, Gram-negative, fungal, protozoan, yeast, and virus pathogenic bacteria and optimizes the intestinal composition of the host.
Besides, it reduces the production of proinflammatory cytosines, improves the immune system, and promotes regulatory T cells (Popović et al., 2021). La. reuteri synthesizes reuterin and reutericin 6, or gassericin A, a circular, small and hydrophobic bacteriocin, which is responsible for providing stability. Among the bacteria responsible for FBD that inhibit La. reuteri are Li. monocytogenes, E.coli, Salmonella sp, and S. aureus (Vázquez-Ortiz et al., 2022).
CONCLUSIONS
Based on the findings described here, it can be determined that the probiotic La. reuteri, which was microencapsulated in a binary matrix composed of inulin and maltodextrin, expresses probiotic properties against Li. monocytogenes (responsible for FBD), and shows great stability after undergoing simulated gastric conditions. The fermentation kinetics allowed to establish its behavior in two culture media. However, the PRO medium was the one with the highest biomass production, indicating a greater quantity of bacteria related to the production of lactic acid exopolysaccharides and other fermentation-derived products that provides beneficial properties to the consumer. In addition, the microencapsulant material contributed to the stability of the evaluated lactic acid bacteria, which is equivalent to good interaction.