INTRODUCTION
Whey is a co-product of cheese or casein manufacture; it is of great interest to the dairy industry due to its large volume and nutritional composition (Gutiérrez-Hernández et al. 2022), especially the content of proteins of high biological value such as β-lactoglobulin (3.2g/L), α-lactalbumin (1.2g/L), bovine serum albumin (0.4g/L), and immunoglobulins (0.7g/L) (Sánchez-Obando et al., 2020). The production of one-two kg of cheese can generate between eight and nine kg of whey. Whey contains the whey proteins of milk, which represent 20% of the total content (Baldasso et al., 2011). It also contains 50% of the total solids of milk, so it is a viable source of nutrients for humans (Masotti et al., 2017). The yield of this co-product has been growing around the world, thus creating important environmental and health problems (Yadav et al., 2015; Gutiérrez-Hernández et al., 2022). Therefore, society requires viable methodologies for its treatment.
The filtration process, specifically ultrafiltration (UF), evolved technologically in the dairy industry due to whey treatment, particularly the recovery of its proteins (Kelly, 2019; Macedo et al. 2021). Thanks to this process, it is possible to obtain whey derivatives such as whey protein concentrates (WPC), which result from removing non-protein constituents from concentrates by UF until they represent more than 25% of the total solids of the final product. WPCs are produced by separation techniques such as precipitation, filtration, or dialysis (Carter et al. 2021)
Microparticulate whey protein (MWP) is obtained by the aggregation and mechanical stress of whey proteins from WPC with low pH adjustments (<5). The resulting spherical particles have been reported with sizes ranging from 0.1 to 10 μm and have been used as fat substitutes (Singer & Dunn, 1990; Ipsen, 2017; Kew et al. 2020) and cheese yield enhancers (Yadav et al., 2015). The particle sizes in the MWP are specific according to their target application. When they are intended to produce cheese, they must be trapped in the protein matrix formed in the coagulation, then, sizes between 0.5 and 10 µm are ideal to achieve retention (Sánchez-Obando et al., 2020). Their use has generated an increase in cheese yield due to the greater retention of moisture and proteins (Yadav et al., 2015; Stankey et al., 2017). Commercial MWPs such as Simplesse® have been commonly used as fat substitutes because their non-aggregated protein microparticles simulate fat properties in reduced or fat-free products (Karaman et al., 2012).
Since the initial developments, the potential use of MWP as a fat substitute has been studied in products such as yogurts (Celigueta et al., 2011; Celigueta et al., 2012; Hossain et al., 2020; Li et al., 2021), ice-cream (Ohmes et al., 1998; Prindiville et al., 2000; Yilsay et al., 2006; Hossain et al., 2021), desserts (Vidigal et al., 2012), and cheeses (Schenkel et al., 2013; Di Cagno et al., 2014; Masotti et al., 2017; Giroux et al., 2018). Moreover, adding MWP to the production of cheeses represents another advantage: the increase in cheese yield and the content of proteins of high biological value, such as whey proteins (Masotti et al., 2017).
“Campesino” cheese, traditional white cheese, is a Colombian fresh cheese made from fresh milk. It has a semi-soft texture and a high-fat content, which classifies as medium fat with a dairy flavor according to Colombian regulations, and has a yield between 13 and 15% (Ministerio de Salud y Protección Social, 1986). The dairy industry seeks for alternatives to improve manufacturing efficiency. Currently, to the authors’ knowledge, there are no research reports including an MWP in the preparation of this variety of cheese. Hence, the aim was to evaluate the development of an MWP from sweet whey and the effect its application has on the yield, texture, and physicochemical characteristics of white cheese.
MATERIALS AND METHODS
Optimization of the MWP preparation process
Obtaining whey protein concentrate (WPC). The filtration process was conducted in an ultrafiltration pilot plant with a capacity for 130L/h of permeate (Perinox, Villa Robledo, Spain), equipped with a polyether sulfone spiral configuration membrane with a ten kDa molecular weight cut-off (Koch Membrane System, Wilmington, MA, USA). A volumetric concentration factor (VCF) of 18, a transmembrane pressure (TMP) of two bars, and a temperature of 48°C were used, as determined by Bejarano-Toro et al. (2021). The sweet whey (pH>6.0) was obtained from the production of white cheese and was provided by a local company. It had a content of 6.98% (w/w) of total solids, 0.85% (w/w) of protein, 4.6% (w/w) of lactose, 0.08% (v/v) of titratable acidy 0.08% (w/w) of fat, and a pH of 6.52. This process used 800 L of whey, which yielded 44 L of concentrate.
Development of the MWP. The microparticulation process is based on the heat treatment of whey protein concentrate (WPC), which generates an interaction between proteins that can be observed in the formation of aggregates. They are homogenized, and their sizes range between one and ten μm (Kethireddipalli & Hill, 2015; Masotti et al., 2017; Kew et al., 2020). The methodologies developed by Lebeuf et al. (1998), Sturaro et al. (2015), and Sánchez-Obando et al. (2020) were considered with modifications. First, 20L of WPC standardized to 18% of total solids with 46% of protein on a dry basis (8.28% of protein on a wet basis), obtained by ultrafiltration of sweet whey (Laboratory of Dairy Products, Universidad Nacional de Colombia), was adjusted to pH 4.2 with reactive grade lactic acid (Giroux et al., 2018). Then, the heat treatment, which varied between 76 and 93°C, was conducted on a STEPHAN® Universal Machine UMSK 24E (Schwarzenbek, Germany) with retention times between 3 and 17 min. At the end of this time, a homogenization cycle was applied at 1000 psi (St. Regis, Chicago, USA) according to the conditions proposed by Kelly (2019) and Sánchez-Obando et al. (2020). Finally, the MWP was neutralized with sodium hydroxide (12.5 N) to a pH of 6.7 to incorporate it into the milk to be transformed into cheese.
Analyses on the MWP. The particle size, measured as a volume (D4, 3), was determined using a Mastersizer 2000 light scattering equipment (Malvern Panalytical, UK) by taking the average of five measurements per sample (Kulmyrzaev & Schubert, 2004; Narong & James, 2008). The zeta potential was measured to determine the colloidal stability of the MWP and its tendency to generate phase separation using a Zetasizer light scattering equipment (Malvern Panalytical, UK). It determines the magnitude of the double electrostatic repulsion layer between the particles or colloids of the MWP. The whey sample was injected into the measuring cell and placed into the equipment, which reports the density of colloid loading in mV. Experiments were performed at 25°C (Kulmyrzaev & Schubert, 2004; Narong & James, 2008; Kaewkannetra et al., 2009). The color was determined with a CM-700d spectrophotometer (Konica Minolta Sensing Americas Inc. NJ, USA), and L * (light-dark index), a * (red-green index), and b* (yellow-blue index) were determined according to the CIELab System (International Commission on Illumination).
Preparation of white cheese added with MWP. A 60kg batch of milk was taken to manufacture the control cheese and the one including 5% of the MWP, obtained from each temperature and retention time conditions established in the experimental design. The traditional white cheese and the cheese added with MWP were made based on the methodology of Vásquez et al. (2014) with modifications. After adding 5% MWP to the milk, the mixture was pasteurized at 63°C for 30 min by batches, cooled down to 35°C, and 1% chymosin (Maxiren® DSM, Germany) was added to coagulate for 30 min; after this time, the formed clot was cut and stirred for ten min. Subsequently, the curd was completely strained, salted with 1.8% sodium chloride (w/w), and left in molds for 12 h. The cheese was stored at 4°C ± 2 °C, and its yield was evaluated by the ratio between the weights (kg) of molded and refrigerated curd and the amount of milk used in the production process (Di Cagno et al., 2014; Sturaro et al., 2015).
Microparticulate whey protein retention (MWPR) in white cheese, expressed as a percentage, was calculated based on a mass balance between the protein content of the MWP, the whey resulting from cheese production including MWP, and the obtained cheese (AOAC 920.53 of AOAC- Association of Official Analytical Chemists, 1997). It was determined by Equation 1, which was used by the Dairy Products Class of the University of Santiago de Compostela (USC-Universidad de Santiago de Compostela, 2015).
Equation 1. Microparticulate whey protein retention.
Where MWPR is the recovery of proteins from MWP; W1= weight of whey obtained from the cheese manufacturing process with MWP; PW1= protein content of whey with MWP (% v/v); PWr= protein content in a reference whey (% v/v); M1= weight of milk used; PMW= protein content in the microparticulate; %MWP= percentage of microparticulate added.
Assessment of the inclusion of the optimized microparticulate into the white cheese production. Preparation of white cheese with the addition of MWP: A 30 L batch of milk was taken to make the control cheese and the one including 3 and 5% of standardized MWP; these levels were determined by previous tests. Both were prepared based on the methodology of Vásquez et al. (2014) with modifications, as previously described. Protein retention and cheese yield were evaluated according to equation 1 and equation 2.
Equation 2 . Cheese yield (yield)
Compositional characteristics of white cheese and whey. The moisture content -where 3g of cheese were taken and placed in a forced air oven at 130°C ± 1°C for 1.5h (AOAC 948.12 of AOAC, 1997)- and fat content (AOAC 989.04 of AOAC, 1997) of the obtained cheeses were determined by Babcock’s test; and the protein content (AOAC 991.123 of AOAC, 1997) by the Kjeldahl methodology using the factor %N x 6.38. The protein content in whey from the control cheese and those added with the MWP were analyzed to calculate the retention of added proteins.
White cheese texture profile analysis. The texture profile analysis (TPA) of white cheese made with MWP was performed on a CT3 Texture Analyzer (Brookfield, Middleboro, MA, USA). The sample was taken in a square of 20mm2. For the analysis, a compression percentage of 50% was used at a test speed of 0.5mm/s with two compression cycles; the probe used was TA25/1000 with a load cell of 10000g. Hardness (g), cohesiveness, elasticity (mm), and chewiness (mJ) were determined (Guerrero-Ramos et al., 2015; Guzmán et al., 2015).
Experimental Design. A central composite response surface design obtained through Statgraphic Centurion 16.1 was implemented to evaluate the development of MWP (Statpoint Technologies, INC.). A total of 14 experiments were conducted considering two factors: the temperature of the heat treatment from 76 to 93°C and the retention time of this heat treatment from three min to 17 min with six focal points (84°C and ten min). Seven response variables were evaluated, namely, particle size (D32), color (L*, a* and b*), zeta potential, protein retention of the MWP (in the processed cheese), and cheese yield. An ANOVA was performed for each response variable using an iterative algorithm implemented in Statgraphic Centurion 16.1 (Statpoint Technologies, INC.). Based on this process, a multiple response optimization was performed by maximizing the desirability function of the model and seeking to obtain maximum values in the particle size (D4, 3), the magnitude of the zeta potential to guarantee greater product stability, the retention of the MWP protein in the cheese matrix, and the cheese yield. These variables are of high technological interest. In the experiment, the pH of the WPC was set at 4.2 and the homogenization pressure at 1000 psi as process constants.
One-way variance analysis (ANOVA) was performed with a significance level of 5% to assess the inclusion of the optimized microparticulate into the preparation of white cheese. The inclusion percentage of MWP was considered as a factor: control cheese without the addition of MWP (control), added with 3% MWP (3%), and with 5% MWP (5%). As response variables, cheese yield, percentage of protein retained in the cheese, TPA (hardness, cohesiveness, elasticity, and chewiness), moisture, protein, and fat content were considered in relation to the dry matter of cheese. The least significant difference test was applied when differences were found. This design was analyzed in Statgraphics Centurion 16.1 (Statpoint Technologies, INC).
RESULTS AND DISCUSSION
Experimental factors had no significant effect on color parameters L, a*, b*, and the zeta potential (Table 1). Regarding color, the WPC is a whitish product with characteristics and appearance similar to those of milk; therefore, the WPC did not undergo browning processes during the heat treatment. This is a desirable parameter because it is added directly to the milk before pasteurization; thus, it must not produce strange colors. L* presented values between 61.41 and 78.9, which is mainly a white product and is similar to that reported by Mcdermott et al. (2016) and Milovanovic et al. (2020): L* value of 81.5 and 81, respectively, on average for cow's milk. Thus, it is also desirable for the application of MWP in milk intended for the manufacture of cheeses.
Likewise, the a* parameter was between -2.15 and -1.23, which indicates a slight tendency towards green color. The b* parameter was between 7.73 and 10.27, thus indicating a tendency towards greenish yellow; this is similar to the description made by Prazeres et al. (2012) and Yasmin et al. (2013) and makes it compatible with the color of milk. Additionally, Croissant et al. (2009) and Chung et al. (2014) found WPC with L*, a *, and b* values around 86.4, 0.18, and 15, respectively. Therefore, there are changes generated by the microparticulation process, but within acceptable values to be added to milk.
Table 1 Results for particle size (D (4,3)), Color (L*, a*, b*), zeta potential, protein retention, and cheese yield.
Factors | Response variables | |||||||
---|---|---|---|---|---|---|---|---|
T °C | Time (min) | Particle size (μm) | Color | Potential Z (mV) | MWPR (%)** | Cheese yield % | ||
D (4,3) | L* | a* | b* | |||||
84 | 10 | 5.94 | 70.47 | -1.31 | 8.41 | 5.18 | 74.07 | 17.30 |
90 | 15 | 7.65 | 69.28 | -1.45 | 10.27 | 7.97 | 66.6 | 19.30 |
84 | 10 | 4.97 | 70.7 | -1.3 | 8.74 | 1.83 | 65 | 17.30 |
93 | 10 | 6.74 | 68.65 | -1.4 | 8.99 | 8.74 | 80.8 | 19.00 |
84 | 10 | 5.36 | 70.86 | -1.34 | 8.53 | 6.79 | 72.6 | 17.90 |
84 | 3 | 5.75 | 69.49 | -1.64 | 8.82 | 3.58 | 80.9 | 18.60 |
76 | 10 | 4.22 | 70.14 | -1.68 | 10.02 | 3.74 | 57 | 17.10 |
84 | 10 | 5.09 | 69.36 | -1.65 | 9.26 | 4.1 | 81.5 | 18.60 |
90 | 5 | 6.95 | 68.62 | -1.23 | 8.12 | 3.71 | 78.9 | 19.30 |
78 | 5 | 4.58 | 70.83 | -1.48 | 8.87 | 4.69 | 60.4 | 17.30 |
84 | 17 | 5.49 | 69.7 | -1.38 | 8.41 | 4.6 | 81.7 | 17.80 |
84 | 10 | 4.73 | 68.27 | -1.36 | 7.73 | 2.12 | 76.8 | 18.20 |
84 | 10 | 4.94 | 70.12 | -1.28 | 8.29 | 5.73 | 72.3 | 18.60 |
78 | 15 | 4.31 | 69.83 | -1.51 | 8.75 | 3.28 | 59.6 | 17.90 |
**MWPR= microparticulate whey protein retention
The temperature had a significant effect on particle size (D4, 3), protein retention, and cheese yield. Regarding the particle size, values between 0.19μm and 6.44μm were obtained. Whey proteins tend to form aggregates when WPCs are subjected to heat treatments (Giroux et al., 2018) due to exposure of the hydrophobic and sulfhydryl groups during heating, which leads to interactions and aggregation (Li et al., 2021). The denaturation of whey proteins depends on time and temperature (Guyomarc’h, 2006); it begins at 40°C and rises to 95% when it reaches 85°C (Kilara & Vaghela, 2004). In addition, this process reduces the solubility of proteins by aggregation (Kilara, 2016). According to Wolz et al. (2016), protein denaturation and aggregation are irreversible processes generated by means of disulfide bonds and hydrophobic interactions, which increase the particle size by increasing the level of protein denaturation. That phenomenon was corroborated in this research; Figure 1a shows that the increase in temperature increases the resulting particle size. The values obtained in this parameter agree with the data reported by authors such as Spiegel & Huss (2002) and Ipsen (2017), who stated that an MWP must have sizes between 0.5 to ten μm because they allow them to be trapped more efficiently by the protein matrix generated in the enzymatic coagulation of milk (Giroux et al., 2018). However, there is a clear trend: the higher the temperature, the larger the particle size (Figure 1a).
Moreover, temperature also had a significant effect on protein retention and cheese yield (Figure 1b and Figure 1c), with values between 57 to 81.7% and 14.1 to 15.3%, respectively. Hinrichs (2001) stated that in semi-hard and semi-soft cheeses, the retention of thermally denatured whey proteins could reach 70%; in this work, retention levels of up to 81.7% were observed. Also, a maximum yield of 15.3% was achieved, on average 2.7% higher than that of the control cheese without the addition of MWP, which was 12.6%. These two variables showed higher performance at higher temperatures in the microparticulation process. This phenomenon is directly related to the particle size that also increases based on this factor. Authors such as Giroux et al. (2018) affirm that sizes close to ten μm tend to be trapped more efficiently by the protein matrix formed in milk by enzymatic coagulation, thus having sizes around 6.74 μm at higher temperatures. It improved the protein retention rate and was directly reflected in cheese yield because MWP has a high-water retention capacity since whey proteins are amphiphilic (Adjonu et al., 2014). Therefore, the cheese yields increase with higher protein retention and higher capacity to retain water in the cheese matrix (Perreault et al., 2016; Mayta et al., 2019).
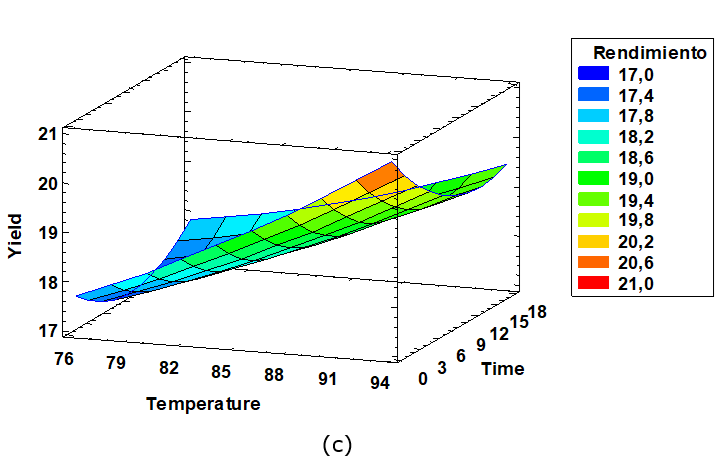
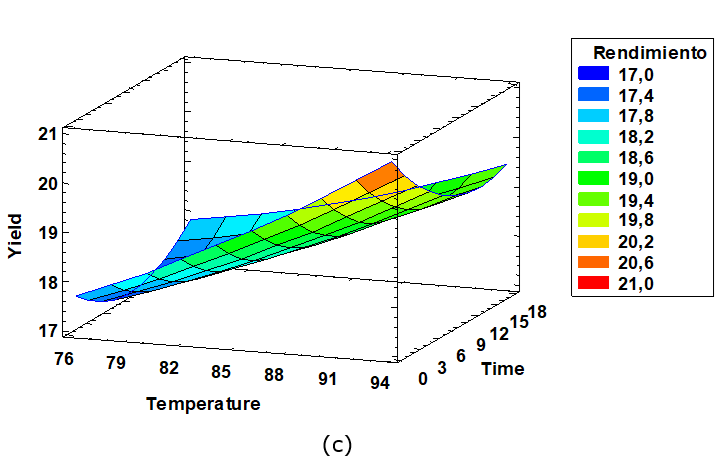
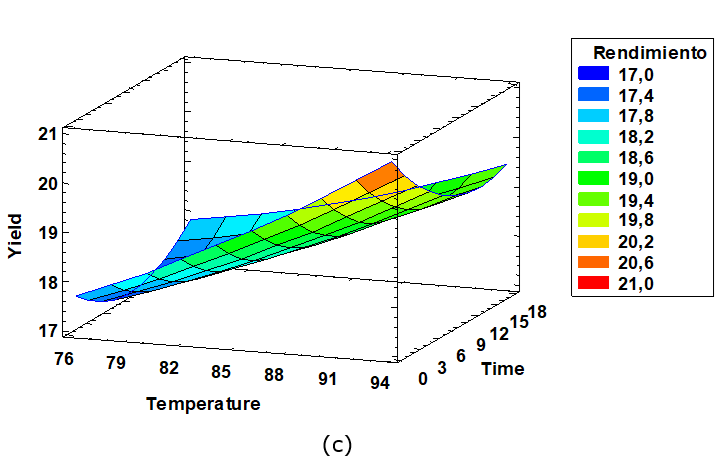
Figure1. Graph of response surfaces for particle size variables D (4, 3) (a), protein retention (%) (b), and cheese yield (%) (c).
The optimization process was performed considering the particle size (D 4, 3), protein retention, cheese yield, and zeta potential as variables. Although the factors had no significant effect on the latter, it is important to maximize it because it contributes to the stability of MWP. An impact of 1, 3, 3, and 1 was assigned to them, respectively, because cheese yield and protein retention are variables of high importance to cheese manufacturers. All of them were optimized based on the desirability function of each possible solution in m possible responses (Equation 2). As a result of the microparticulate analysis of the obtained WPC, the optimal process conditions were 93°C for a retention time of 5.4 min with a desirability of 0.91, to be subsequently subjected to a homogenization process at a pressure of 1000 psi. Coto (2014) worked with different pH values of WPC (4, 5.5, and 7), a temperature of 80°C, and a homogenization pressure of 4000 psi. The author obtained similar particle sizes by adjusting pH to 4, although it showed that the higher the temperature, the bigger the size of the protein aggregates, thus supporting the results. Once these conditions were defined, the optimal MWP was obtained and included in the preparation of white cheese. The optimal values of the response variables were 7.21 µm, 5.6 mV, 81.7%, and 19.7% for particle size, zeta potential, protein retention, and cheese yield, respectively.
Equation 3. Equation of the desirability function
Inclusion of the MWP developed in the production of white cheese. The data on yield, protein retention, and texture variables obtained from the experiment can be seen in Table 2. The inclusion of MWP had a significant effect (P<0.05) on all whey protein retention (MWPR) variables: cheese yield, hardness, cohesiveness, elasticity, and chewiness. The inclusion of 3% presented greater MWPR when retaining an average of 58.95% with respect to the 5% that only retained 49.2%. Therefore, the addition of 3% of MWP is more efficient. These types of products are used as fat substitutes in food processing (Kelly, 2019), particularly in milk. The microparticulate protein is retained in the protein matrix by the same mechanism that fat globules use during coagulation, resulting in mouthfeels similar to whole milk products (Hinrichs 2001; Masotti et al., 2017). It can also be released to whey when the curd is cut, resulting in some protein losses. The protein retention of the MWP leads to a cheese yield increase from 12.6% in the control cheese to 14.5 and 15.1% in the 3 and 5% treatments, respectively. These proteins increase the cheese yield not only because of protein retention but also because they have a high-water retention capacity (Hinrichs, 2001; Masotti et al., 2017; Kelly, 2019). This phenomenon is corroborated by the moisture content of the cheeses (Table 3); those added with MWP had greater moisture with respect to the control cheese by 4.11 and 5.1% for the 3 and 5% treatments, respectively. Stankey et al. (2017) applied MWP in the production of cheddar cheese and increased the moisture content and yield with respect to the control cheese. Di Cagno et al. (2014) documented the same phenomenon in its application to caciotta cheese.
Table 2 Results for protein retention, yield, and TPA of the cheeses added with MWP and the control cheese*.
Parameter | Control | 3% | 5% |
---|---|---|---|
MWPR** % | 0.00a | 58.95 ± 4.97b | 49.21 ± 5.9c |
Yield % | 12.6± 0.0017a | 14.5± 0.0027b | 15.1± 0.002c |
Hardness (N) | 20.52± 1.55a | 16.94± 0.67b | 13.91± 0.66c |
Cohesiveness | 0.66± 0.011a | 0.56± 0.007b | 0.51± 0.015c |
Elasticity (mm) | 8.78± 0.046a | 8.38± 0.07b | 8.44± 0.1b |
Chewiness (mJ) | 153.11± 4.28a | 81.53± 2.2b | 61.92± 0.85c |
*Values with different letter superscripts in a row represent statistically significant differences (P<0.05). If the superscript letter is the same, there are no significant differences (P>0.05). ** MWPR= microparticulate whey protein retention
Table 3 Moisture, protein on a dry basis, and fat on a dry basis from processed cheeses*.
Parameter | Control | 3% MWP | 5% MWP |
---|---|---|---|
Moisture | 58.09± 1.7a | 62.11± 1.0b | 63.10± 1.2b |
MG/ES** | 44.8± 0.018a | 42.3± 0.01b | 39.1± 0.01c |
P/ES*** | 39.5± 0.01a | 40.7± 0.01a | 40.5± 0.009a |
*Values with different letter superscripts in a row represent statistically significant differences (P<0.05). If the superscript letter is the same, there are no significant differences (P>0.05). ** MG/ES=fat in dry extract. *** P/ES= total protein in dry extract.
The cheese hardness decreased from 20.52 N to 13.91 N with the inclusion of 5% MWP. This behavior is due to the increase in cheese moisture due to whey protein water retention capacity. Di Cagno et al. (2014), Stankey et al. (2017), and Masotti et al. (2017) have reported a reduction in hardness by including MWP in the production of cheeses because these materials are used as inert fillers in the para-caseinate matrices. Thus, it could interrupt the continuity of the protein matrix and create weak points, which would reduce the hardness of the product (Stankey et al., 2017). This behavior explains the reduction in the cheese cohesion and elasticity because the weaknesses in the matrix can make it more fracturable and with less cohesion between components. Compositional variables are shown in Table 3. Moisture was significantly higher in the cheeses added with MWP due to the high-water retention capacity of whey proteins. Ismail et al. (2011) included whey proteins in the production of mozzarella cheese and obtained similar results. Adding whey proteins increased the cheese moisture and, consequently, increased the cheese yield. On a dry basis, the fat showed significant differences (P<0.05) between the control cheese and the cheeses added with MWP. The addition of proteins with particle sizes between one μm and ten μm allow them to be trapped by the pores of the para-casein network formed during milk coagulation (Kelly, 2019). This is due to the fat globule retention system, which entraps them in the spaces between the coagulated caseins (Sturaro et al., 2015). Therefore, the protein retention in these pores, or the generation of larger pores, leads to a competition between the fat and the MWP by occupying these spaces and reducing the retention of fat globules in the cheeses added with 3 and 5% MWP with respect to the control cheese, which was not added with the microparticulate. This behavior was reported by Stankey et al. (2017), who added MWP to the preparation of cheddar cheese, and by Johnson et al. (2001) to reduced-fat cheddar cheese. The opposite occurred with respect to the protein content on a dry basis. There were no significant differences (P>0.05) between the control cheese and the treatments (3 and 5%), even though, the cheeses added with MWP had higher moisture content. They had 40.7 and 40.5% of protein on a dry basis, compared to 39.5% in the control cheese. This indicates that there was effective retention of whey proteins, corroborated by the retention data of the added protein (Table 1), which were 58.95 and 49.21% for the 3 and 5% treatments, respectively.
CONCLUSIONS
The optimal conditions to obtain the microparticulate whey protein (MWP) were a temperature of 93°C with a retention time of 5.4 min, with optimal values of 7.21µm for particle size, 5.61mV for zeta potential, 81.7% for protein retention, and 19.7% yield. The goal of the mechanical homogenization treatment was to generate high protein denaturalization and aggregation to achieve the desired particle sizes (one μm-ten μm). The addition of MWP to milk used in white cheese production had interesting effects on variables such as cheese yield, increasing it by 2.7% more than control cheese. However, when evaluating the texture characteristics, the differences with respect to the control were much more remarkable for the cheese with 5% MWP. Regarding protein recovery, it was more efficient in cheeses with 3% MWP. Based on the results, it can be suggested that adding 3% of this MWP to milk can generate important improvements in the cheese yield and retention of whey proteins and, therefore, in its nutritional quality. The MWP development allows an adequate valorization of the ingredients present in whey since microparticulates have been gaining ground in the development of low-fat foods due to their application as a fat substitute and as an alternative to recover whey solids, thus mitigating their environmental impact.