Introduction
The early diversification of angiosperms has been studied mostly by dispersed pollen and detached leaves (e.g., Doyle and Hickey, 1976; Hughes, 1976; Hickey and Doyle, 1977; Hughes, 1994) and flowers (Friis et al., 2011). There is not a distinctive pattern in the latitudinal distribution of early flowering plants, as Early Cretaceous angiosperms from both middle northern paleolatitudes and the tropics, are similar in diversity and abundance (Friis et al., 2011). Macrobotanical evidence from North America indicates that angiosperms only became ecologically significant at high latitudes by the earliest Late Cretaceous, but mostly restricted to riparian environments (Wing et al., 1993). Estimates based on pollen (diversity and abundance) suggest that angiosperms were already dominant in some environments at mid-paleolatitudes by the Cenomanian (Lupia, 1999). In contrast, the Cretaceous patterns of angiosperm radiation and diversification at low latitudes are not well understood (Mejia-Velasquez et al., 2012; Jaramillo, 2012, 2019), particularly because there are very few studies from tropical latitudes that have assessed the whole floristic composition (Crane and Lidgard, 1989; Mejia-Velasquez et al., 2012).
Floras preserved in amber can be very informative but they are very rare in the geological record. Recently, we found two amber deposits in Ecuador (Hollín Formation) and Colombia (Une Formation) that have the potential to be very useful to unravel the nature of tropical floras during the early Cretaceous. Here, we analyze the palynological content of both deposits to assess their age, determine their broad floristic composition, and interpret if they accumulated under humid or arid conditions.
Methods
Several localities with amber were studied for the Hollín Formation in Ecuador. The first consists of strata from the lower Hollín Formation that crop out at the abandoned Pungarayacu quarry, Oriente Basin (Lat: -0.70700, Long: -77.74100), the second also from the lower Hollín Formation at the Genoveva mine, Oriente Basin, Ecuador (Figure 1A) (Lat: -0.71102, Long: -77.78778; Albian paleolatitude: -6.92, paleolongitude: -53.87, coordinate reconstruction by Muller et al. (2016)). The third set was collected from upper Hollín Formation outcrops along the Misahualli river (Lat: -1.00120, Long: -77.67280). Along the same river, several samples of the lower Napo Formation were also collected (Table SM1).
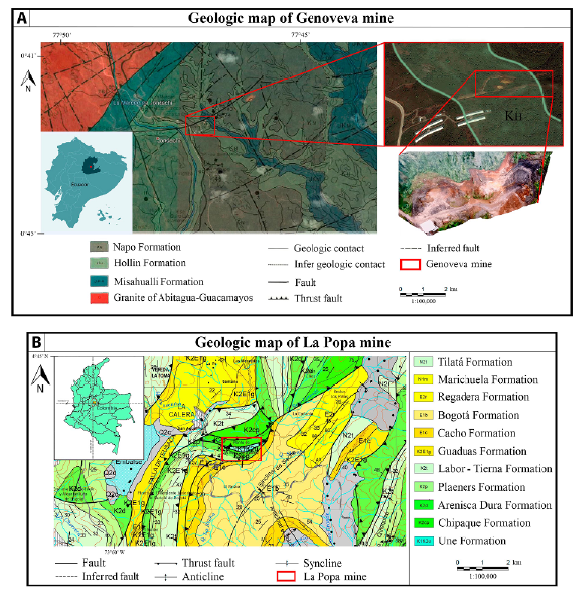
Figure 1 Geologic maps of the study zones. A. Genoveva mine in Ecuador. Map after Eguez et al. (2017). B. La Popa mine in Colombia. Map after Buitrago et al. (2008) and Alcárcel and Gómez (2019).
Samples of the Une and the overlying Chipaque Formations were collected from outcrops within the abandoned La Popa mine (Figure 1B) (Lat: 4.70866, Long: -73.95213, Albian paleolatitude -1.77, paleolongitude -50.29, coordinate reconstruction by Muller et al. (2016). At this site, the Une Formation is seen as lens of sandstone that is rich in amber and it is bounded above and below by the shales of the Chipaque Formation, and the contact seems to be tectonic. Additional samples were collected just above and below the amber-bearing lens in shales of what appears to be the Chipaque Formation. Additional samples were taken elsewhere in the mine from shales of the Chipaque Formation.
Geologic setting
Paleozoic basement, Mesozoic-Cenozoic volcanic rocks, and Cretaceous-Cenozoic sedimentary units are exposed in the Oriente Basin of Ecuador (Vallejo et al., 2002). The Hollín Formation has been widely studied because it is the main oil reservoir of the basin (Dashwood and Abbotts, 1990). It comprises two units, the lower Hollín Formation, known as the Main Hollín Sandstone (Baby et al., 2004), and the Lower Sandstone, known as the Upper Hollín Sandstone or upper Hollín Formation (Jaillard et al., 1997). The Hollín age ranges from middle-late Aptian to middle Albian (White et al., 1995; Jaillard et al., 1997; Vallejo et al., 2002; Ordoñez et al., 2006; Romero et al., 2017). In contrast, Sarmiento-Rojas (2019) suggests an early Aptian-middle Albian age. The lower Hollín Formation is dominated by conglomeratic sandstones that grade to quartz sandstones with cross-bedding deposited in braided river channels (White et al., 1995; Romero et al., 2019). The upper Hollín Formation is characterized by sandstones and mudstones intercalations. These mudstones are rich in organic matter and leaf fragments, and the system deposited in coastal plains to shallow platforms (White et al., 1995; Romero et al., 2019; Sarmiento-Rojas, 2019). The upper boundary of Hollín and the overlying Napo Formation corresponds to a major flooding surface (Romero et al., 2019).
The Napo Formation age ranges from late Albian to early Campanian (Mello et al., 1995; Vallejo et al., 2002; Sarmiento-Rojas, 2019) and consists of organic-rich shales, limestones, and sandstones. It is subdivided into several informal members that can be correlated over large distances in the Oriente Basin of eastern Ecuador (Tschopp, 1953). Two sandstone units (labeled T and U sandstones) constitute the most important hydrocarbon reservoirs, and the organic-rich zones (e.g., Basal Shale Member) are considered the source of almost all hydrocarbons in the basin (Rivadeneira, 1986; Dashwood and Abbotts, 1990; Mello et al., 1995; Vallejo et al., 2002). The Napo Basal member has been dated as late Albian by palynological studies (Vallejo et al., 2002) and has been interpreted as deposited in dysoxic-anoxic, paralicneritic environments (Mello et al., 1995) or in inner shelf environments (Sarmiento-Rojas, 2019).
The Eastern Cordillera of Colombia is composed of Phanerozoic sedimentary rocks, with local exposures of a Proterozoic-lower Paleozoic basement with multiple orogenic episodes (Etayo-Serna et al., 1983; Cediel et al., 2003; Bayona et al., 2008; Horton et al.,, 2010; Saylor et al., 2012). The Une Formation can be several hundred-meters thick, it is widely distributed in the Eastern Cordillera, and is a clastic unit (Zavala et al., 2009). The Une Formation forms strong geomorphological escarpments that contrasts with the underlying Fómeque Formation and the overlying Chipaque Formation, which are both fine-grained and generate valleys (Ulloa et al., 2001; Corredor and Terraza, 2015). The Une Formation is composed by black mudstones, dark grey quartz-siltstones and white quartz-sandstones with dark siltstone intercalations that are found in thin to very thick tabular layers. The siltstones are locally calcareous and fossiliferous, while the mudstones have lenticular bedding with the presence of fossil bivalves and abundant plant remains (Reyes, 1984; Corredor and Terraza, 2015). The formation has been dated as Albian-Cenomanian (Renzoni, 1981; Fabre, 1985; Montoya and Reyes, 2003) and interpreted as accumulated from very shallow marine settings to deltaic deposits (Renzoni, 1962; Ulloa et al., 2001; Sarmiento-Rojas, 2019). The overlying Chipaque Formation is dominated by claystones and mudstones (Renzoni, 1962; Guerrero and Sarmiento, 1996; Corredor and Terraza, 2015). This unit has been dated as late Cenomanian to Santonian (Montoya and Reyes, 2003; Corredor and Terraza, 2015), while Guerrero and Sarmiento (1996) indicated that the lower part of the Chipaque Formation is early Turonian. It accumulated in a shallow marine platform (Ulloa and Rodríguez, 1976; Villamil, 1998; Sarmiento-Rojas, 2019) when the tectono-eustatic base level reached its maximum level during the Mesozoic (Sarmiento-Rojas et al., 2006; Corredor and Terraza, 2015; Sarmiento- Rojas, 2019).
Sampling and processing
Ten samples of dark, fine-grained facies along the 72.5 m thick stratigraphic section (Figure 2) that is exposed at the Genoveva mine (Ecuador). The strata in the mine indicated a variety of environments that include proximal braided rivers, lacustrines, hyperpycnal flows and distal braided rivers (Table 1). Twelve samples were collected in the Pungarayacu quarry (Lat: 0.70700, Long: -77.74100), three along the Misahualli river and two additional sites (Table SM1). Five samples from the lower Napo Formation were collected along the Misahualli river in four localities (Table SM1).
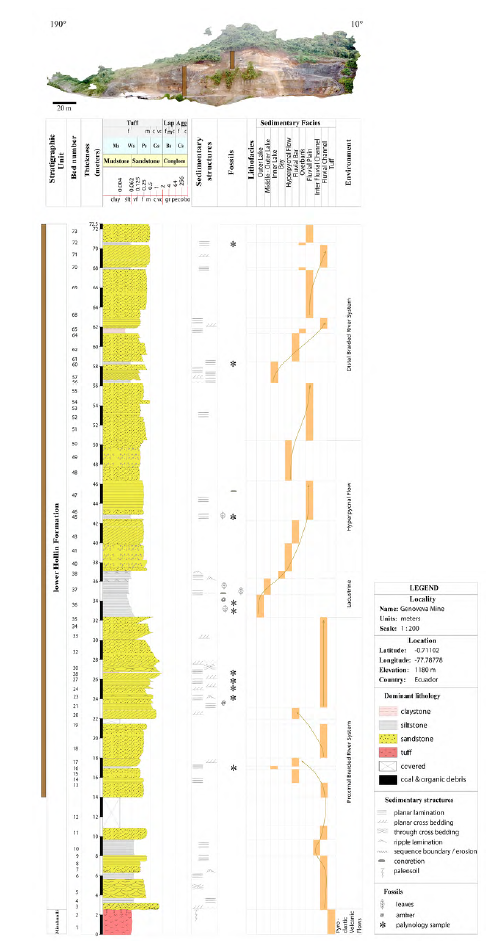
Figure 2 General orthomosaic and stratigraphic column with 1:200 scale of the Genoveva mine. In the orthomosaic, the brown bar shows where the stratigraphic column was created.
Table 1 Samples (top to base) collected from the Genoveva mine, lithology, sedimentary facies and environment.
Stratum base (m) | Stratum top (m) | STRI ID | Lithology | Sedimentary facies | Environment |
---|---|---|---|---|---|
70.4 | 70.7 | 47733 | Dark grey siltstone | Overbank | Distal braided river system |
58.2 | 58.5 | 47732 | Dark grey siltstone | Inner lake | Distal braided river system |
42.5 | 42.9 | 47731 | Grey siltstone | Fluvial plain | Hyperpycnal flow |
33.6 | 33.7 | 47724 | Dark grey siltstone | Outer lake | Lacustrine |
32.6 | 32.7 | 47723 | Dark grey siltstone | Outer lake | Lacustrine |
26.7 | 26.8 | 47725 | Dark grey very fine-grained sandstone | Fluvial channel | Proximal braided river system |
25.8 | 25.9 | 47722 | Dark grey very fine-grained sandstone | Fluvial channel | Proximal braided river system |
24.8 | 24.9 | 47721 | Dark grey very fine-grained sandstone | Fluvial channel | Proximal braided river system |
24 | 24.1 | 47720 | Dark grey very fine-grained sandstone | Fluvial channel | Proximal braided river system |
16.9 | 17 | 47734 | Dark grey siltstone | Inner lake | Proximal braided river system |
At La Popa mine (Colombia), 10 samples were collected from the Une Formation and five samples from the overlying Chipaque Formation (Table SM1). There is a large degree of structural complexity at the mine with several inverse faults and folds, making it difficult to produce a continuous stratigraphic section. The amber deposit is located in a 5 meters thick lens of the Une Formation that is bounded by faults above and below, and embedded in the shales of the Chipaque Formation. It was not possible to determine what was the stratigraphic position of the Une lens within the whole Une Formation that can be up to 600 meters thick.
Samples were prepared following the standard procedure described by Traverse (1988, 2007) at the Laboratorio de Palinología y Materia Orgánica of the Instituto de Investigaciones en Estratigrafía (IIES) at Universidad de Caldas. The sample 47748 from the lower Napo Formation was also processed for calcareous nannoplankton by smear slide (Bown and Young, 1998).
Palynological analysis
The morphological database of Jaramillo and Rueda (2022) which includes all palynological publications from South America was used to identify the palynomorphs. The biostratigraphic zonation of Muller et al. (1987) proposed for northern South America was used for the age determinations. An additional zonation for South America developed for Brazil (Regali et al. 1974) was not used here as it is based on sites from the eastern region of South America while our samples were collected in western South America.
Palynomorphs were grouped into five groups including angiosperm pollen, non-gnetalean gymnosperm pollen, gnetalean pollen, spores (Figures 3-5), and marine palynomorphs. Gnetales were placed in their own category rather than with other gymnosperms because they are an important component of equatorial Cretaceous floras (Herngreen et al., 1996) as well as climate indicators. Humidity was estimated by comparing the total diversity of dry indicators (Classopollis, ephedroid pollen grains, and elater-bearing species) vs humid indicators (fern spores), following previous studies (Herngreen et al., 1996; Mejia-Velasquez et al., 2012, 2018).
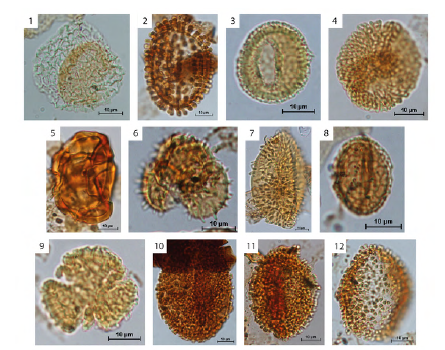
Figure 3 Angiosperm taxa found in the study. 1. Afropollis jardinus (T8-2, 47728; Hollín Formation); 2. Baculamonocolpites sp. (D8-3, 47732; Hollín Formation); 3. Clavatipollenites hughesii (F26-1, 47725; Hollín Formation); 4. Cornetipollis perforatus (P37-1, 47725; Hollín Formation); 5. Cretacaeiporites sp. (T14, 47723; Hollín Formation); 6. Droseridites senonicus (O17-3, 46432; Chipaque Formation); 7. Echimonocolpites sp. (U22, 47725; Hollín Formation); 8. Pennipollis peroreticulatus (D3-4, 47728; Hollín Formation); 9. Retitricolpites operculatus (P17, 47720; Hollín Formation); 10. Stellatopollis barghoornii (J36-4, 47728; Hollín Formation); 11. Stellatopollis densiornatus (V7-3, 46872; Hollín Formation); 12. Stellatopollis dubius (Q30-1, 44889; Hollín Formation).
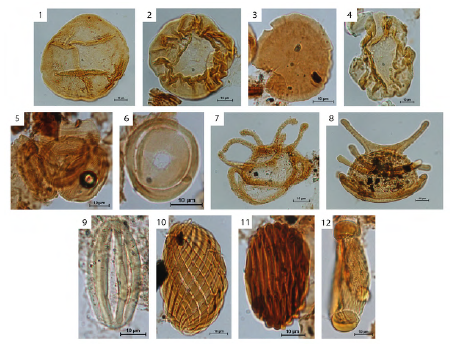
Figure 4 Gymnosperm taxa found in the study. 1-6. Non-gnetalean gymnosperm pollen, 7-12. Gnetalean pollen. 1. Araucariacites australis (G2-1, 44663; Hollín Formation); 2. Callialasporites dampieri (J21, 44663; Hollín Formation); 3. Callialasporites infirmus (K40-4, 47733; Hollín Formation); 4. Callialasporites trilobatus (J10, 44663; Hollín Formation); 5. Classopollis classoides (U3-4, 44884; Hollín Formation); 6. Classopollis meyeriana (M7-2, 47730; Hollín Formation); 7. Elaterosporites klaszii (X16, 44892; Napo Formation); 8. Elaterosporites verrucatus (J42-4, 44892; Napo Formation); 9. Ephedripites ambonoides (Q19, 47748; Napo Formation); 10. Ephedripites barghoornii (N45, 44889; Hollín Formation); 11. Gnetaceaepollenites retangularis (H31-2, 48376; Une Formation); 12. Steevesipollenites cf. multilineatus (S33-4, 47720; Hollín Formation).
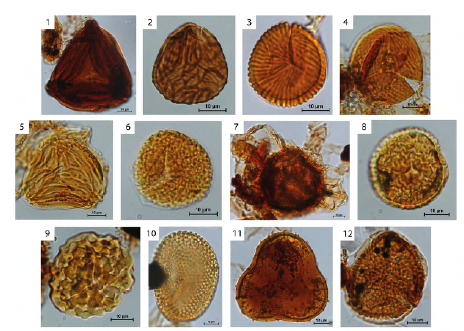
Figure 5 Spore taxa found in the study. 1. Appendicisporites erdtmanii (Q28-1, 44889; Hollín Formation); 2. Camarozonosporites ambigens (W16-1, 44892; Napo Formation); 3. Cicatricosisporites avnimelechi (O10-2, 44661; Hollín Formation); 4. Cicatricosisporites hallei (V30, 44661; Hollín Formation); 5. Cicatricosisporites hughesii (V14-1, 44661; Hollín Formation); 6. Cicatricosisporites kedvesii (E22-1, 44662; Hollín Formation); 7. Crybelosporites pannuceus (J15-4, 44661; Hollín Formation); 8. Hamulatisporis insignis (U20-2, 46427; Une Formation); 9. Ischyosporites variegatus (O41, 46426; Chipaque Formation); 10. Microfoveolatosporis skottsbergii (U12-4, 47725; Hollín Formation); 11. Pilosisporitesparvispinosus (H18-1, 44889; Hollín Formation); 12. Sestrosporites pseudoalveolatus (L41-1, 44883; Hollín Formation).
Results
Palynological assemblages
The assemblage found in the Hollín Formation samples includes Afropollis complex, Araucariacites australis, Callialasporites dampieri, Callialasporites trilobatus, Cicatricosisporites hallei, Classopollis classoides, Cretacaeiporites sp., Crybelosporites pannuceus, Ephedripites complex, Gnetaceaepollenites complex, Ischyosporites variegatus, Pennipollis peroreticulatus, Pilosisporites sp., Retitricolpites operculatus and Steevesipollenites complex (Table SM2).
The assemblage of the Napo Formation samples includes Elaterosporites klaszii, Elaterosporites verrucatus and Ephedripites complex (Table SM2). The calcareous nannoplankton of sample 47748 from lower Napo Formation showed a good to moderate preservation (Figure 6). Assemblage includes Chiastozygus spp., Cyclagelosphaera reinhardtii, Rotelapillus crenulatus, Gartnerago cf. nanum, Gartnerago theta, Manivitella pemmatoidea, Prediscosphaera columnata, Radiolithus planus, Staurolithites halfanii, Watznaueria cf. britannica, Zeugrhabdotus scutula, Zeugrhabdotus diplogrammus and Zeugrhabdotus elegans.
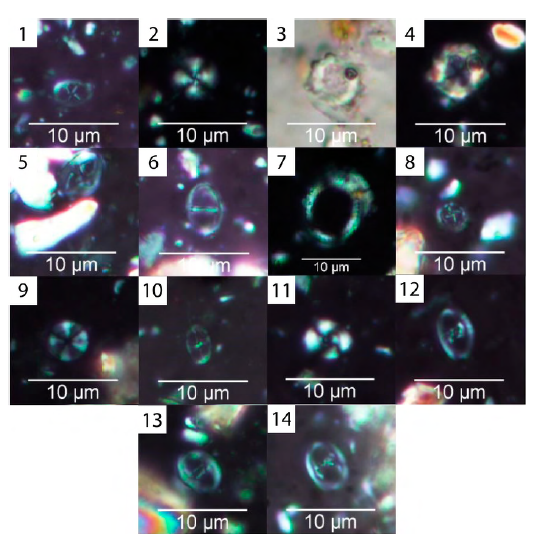
Figure 6 Calcareous nannofossils in sample 47748, lower Napo Formation. 1. Chiastozygus spp.; 2. Cyclagelosphaera reinhardtii; 3-4. Rotelapillus crenulatus; 5. Gartnerago cf. nanum; 6. Gartnerago theta; 7. Manivitella pemmatoidea; 8. Prediscosphaera columnata; 9. Radiolithus planus; 10. Staurolithites halfanii; 11. Watznaueria cf. britannica; 12. Zeugrhabdotus scutula; 13. Zeugrhabdotus diplogrammus; 14. Zeugrhabdotus elegans.
The assemblage found in the Une Formation samples includes Afropollis complex, Araucariacites australis, Callialasporites dampieri, Gnetaceaepollenites complex and Ischyosporites variegatus (Table SM2).
The assemblage of the Chipaque Formation samples includes Callialasporites dampieri, Droseridites senonicus and Ischyosporites variegatus (Table SM2).
Floristic composition and climatic estimation
The Hollín Formation palynoflora is composed by 166 taxa, including 52 angiosperms, 14 non-gnetalean gymnosperm pollen, 22 gnetalean pollen and 78 spores. The Napo Formation palynoflora is composed by 25 taxa, including nine angiosperms, five gnetalean pollen, six spores, and five dinoflagellate species. The Une Formation palynoflora is composed by 35 taxa, including 10 angiosperms, five non-gnetalean gymnosperm pollen, two gnetalean pollen and 18 spores. The Chipaque Formation palynoflora is composed by 19 taxa, including six angiosperms, two non-gnetalean gymnosperm pollen, nine spores and two dinoflagellate species. The palynoflora diversity in the Hollín Formation is dominated by humid indicators compared to dry indicators (75%, 78 vs 26 taxa respectively), while the Une Formation also has a higher diversity of humid indicators compared to dry indicators (90%, 18 vs 2 taxa respectively) (Table SM2).
Discussion
Geological Age
The co-occurrence of Callialasporites dampieri, Callialasporites trilobatus, Afropollis jardinus, Cicatricosisporites hallei, Pennipollis peroreticulatus, Crybelosporites pannuceus and Gnetaceaepollenites barghoornii in the Pungarayacu quarry (Table 2) indicate the assemblage belongs to the Zone 5 of Muller et al. (1987), that is dated as latest Aptian to the earliest Albian (Figure 7). The Genoveva mine seems to be in a younger stratigraphic position and the co-occurrence of Retitricolpites operculatus and Gnetaceaepollenites jansonii (Table 2), indicates zones 6 to 8 of Muller et al. (1987), that are dated as Albian to Cenomanian (Figure 7), but given the Genoveva mine is stratigraphically near the base of the formation and therefore close stratigraphically to the Pungarayacu quarry, it seems reasonable to consider the sequence in the Genoveva mine as Albian. The co-occurrence of Crybelosporites pannuceus, Ephedripites barghoornii, Ephedripites multicostatus and Gnetaceaepollenites retangularis in the Misahualli Hollín river (Table 2) indicates zones 5 to 8 of Muller et al. (1987), that are dated as latest Aptian to Cenomanian (Figure 7), but given the strata are stratigraphically near the top of the formation and the lower Napo Formation is dated as late Albian (see below), it seems reasonable to consider the sequence of the upper Hollín Formation in the Misahualli Hollín river as early Albian. Therefore, the whole Hollín Formation would encompass the late Aptian to the early Albian, supporting ages by previous studies (White et al., 1995; Jaillard et al., 1997; Vallejo et al., 2002; Ordoñez et al., 2006; Romero et al., 2017).
Table 2 Biostratigraphic key taxa found in this study. 1 = if taxon is present.
Geological Formation | Hollín | Hollín | Hollín | Napo | Une | Chipaque | Chipaque | Chipaque |
---|---|---|---|---|---|---|---|---|
Stratigraphic Position | Pungarayacu quarry | Genovena Mine | Misahualli Hollín river | Misahualli river | Lens with amber | below lens with amber | above lens with amber | La Popa Mine |
Taxa Name | ||||||||
Afropollis jardinus | 1 | 1 | ||||||
Callialasporites dampieri | 1 | 1 | 1 | 1 | ||||
Callialasporites trilobatus | 1 | |||||||
Cicatricosisporites hallei | 1 | |||||||
Crybelosporites pannuceus | 1 | 1 | ||||||
Droseridites senonicus | 1 | |||||||
Elaterosporites klaszii | 1 | |||||||
Elaterosporites verrucatus | 1 | |||||||
Ephedripites barghoornii | 1 | |||||||
Ephedripites cf. procerus | 1 | |||||||
Ephedripites multicostatus | 1 | |||||||
Equisetosporites aff. minuticostatus | 1 | |||||||
Equisetosporites ambiguus | 1 | |||||||
Equisetosporites dudarensis | 1 | |||||||
Equisetosporites fragilis | 1 | |||||||
Equisetosporites laticostatus | 1 | |||||||
Gnetaceaepollenites barghoornii | 1 | |||||||
Gnetaceaepollenites jansonii | 1 | |||||||
Gnetaceaepollenites retangularis | 1 | 1 | ||||||
Ischyosporites variegatus | 1 | 1 | ||||||
Pennipollis peroreticulatus | 1 | |||||||
Retitricolpites operculatus | 1 |
The occurrence of Elaterosporites klaszii and Elaterosporites verrucatus in the lower Napo Formation at the Misahualli river (Table 2) indicate the zones 6 to 7 ofMuller et al. (1987), that are dated as Albian to early Cenomanian (Figure 7). The age is further refined by the calcareous nannoplankton assemblage of sample 47748, which according to the concurrence of Gartnerago theta, Gartnerago cf. nanum, and Prediscosphaera columnata, suggest a zonal range between BC27c to UC3a (Burnett et al., 1998) equivalent to late Albian-middle Cenomanian. Therefore, the lower Napo Formation could be considered as late Albian, an age that agrees with previous interpretations (Mello et al., 1995; Vallejo et al., 2002; Sarmiento-Rojas, 2019).
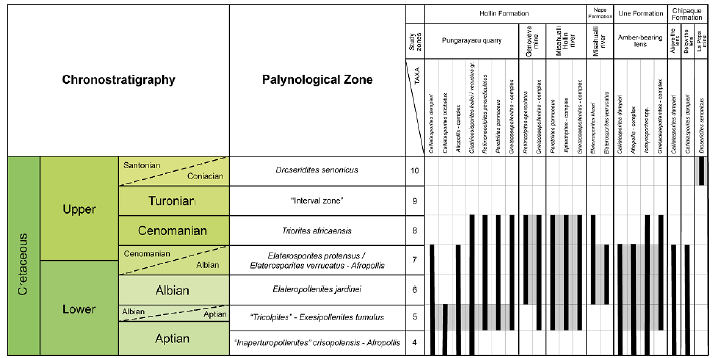
Figure 7 Temporal distribution of the biostratigraphy index taxa found in this study. The gray band represents the estimated age according to Muller et al. (1987).
The co-occurrence of Callialasporites dampieri, Afropollis jardinus, Ischyosporites variegatus, and Gnetaceaepollenites retangularis in the amber-bearing lens of the Une Formation at La Popa mine (Table 2) belongs to the Zones 5 to 7 of Muller et al. (1987), that is dated as latest Aptian to the early Cenomanian (Figure 7). The age of the lens lies within the age span that has been considered for the formation (e.g., Renzoni, 1981; Fabre, 1985; Montoya and Reyes, 2003).
The occurrence of Droseridites senonicus in one sample of the Chipaque Formation at la Popa mine indicate the Zone 10 of Muller et al. (1987) dated as Coniacian-Santonian (Figure 7), age agree with previous interpretation of the Chipaque Formation (Montoya and Reyes, 2003; Corredor and Terraza, 2015). Callialasporites dampieri occurs in two samples of the Chipaque Formation (Table 2) taken immediately above and below the lens containing the amber. This taxon would imply a not-younger than Cenomanian age according to Muller et al. (1987) zonation. However, we have considered both contacts as faults and it could be possible that both samples are within the fault zone and have a mixture of Chipaque and Une palynofloras as it also contains the dinoflagellate Spiniferites. It also could be possible that the shales above and below also belong to the Une Formation, therefore, further stratigraphic work is needed in La Popa mine which has a high degree of structural complexities.
Humidity
The taxonomic composition of the Albian Hollín Formation indicates a flora dominated by humid indicators, similar to the flora found in the samples of the Une Formation. This result agrees with the previous studies from northwestern tropical South American that also show high levels of rainfall (Mejia-Velasquez et al., 2012, 2018). These results contrast with eastern tropical South American, where the well-studied Crato Formation indicates arid climate conditions (Mohr and Rydin, 2002; Batten, 2007; Braz, 2012; Bernardes-de-Oliveira et al., 2014; Ribeiro et al., 2021). Additional evidence suggests a humid climate during the Albian in northwestern South America, such as the isotopic composition of coal layers found in Albian deposits from the Caballos Formation in Colombia that showed mean annual precipitation values slightly higher than the those occurring today in tropical latitudes (Suarez et al., 2010). Furthermore, several Global circulation climate models also predict a precipitation gradient within the tropics in South America, from being wet in the west towards dry in the east (Ufnar et al., 2004; Fluteau et al., 2007; Hay and Floegel, 2012; Kuethe, 2016).
Conclusions
The Hollín Formation was dated as spanning the late Aptian to early Albian while the lower Napo Formation has a late Albian age.
The amber-bearing lens of the Une Formation at La Popa mine was dated as latest Aptian to early Cenomanian, while one sample of the Chipaque Formation was dated as Coniacian-Santonian.
The overall floristic assemblage suggests a humid climate conditions in the northwestern margin of Gondwana.