Introduction
One of the most important pests of fruit in modern production is the fruit-fly (Tephritidae). The damage is caused by larvae during their development when feeding on the me-socarp and by female when they make a puncture proof or oviposition (Nascimento and Carvalho 2000). This puncture causes oviposition holes that can cause scarring, deformation in developing fruits and favors the entry of phytopathogens which lowers fruit quality and reduces market value (Nora and Sugiura 2001; Follett et al. 2019). There are probably 1,500 Tephritidae species relating to fruits around the world. Of these, more than 250 species can cause serious damage (Qin et al. 2015), resulting in large economic losses. The most common and important genera are Anastrepha Schiner, 1868, Bactrocera Macquart, 1835, Ceratitis Macleay, 1829, Dacus Fabricius, 1805 and Rhagoletis Loew, 1862 (Qin et al. 2015). Ceratitis capitata (Wiedemann, 1824) (Mediter-ranean-fruit fly) is one of the most important threats to fresh fruits worldwide and has registered as hosting more than 205 kinds of fruits (CABi 2019). In Brazil and other American countries, Anastrepha species are very important (CABi 2019). The South American fruit fly Anastrepha fraterculus (Wiedeman, 1830), is one of the main fruit pests in South America due to its large variety of hosts and a broad geographic distribution (Norrbom 2004; De Sá et al. 2012). According to Rull et al. (2012)A. fraterculus is recognized as a complex of cryptic species composed of several different morphotypes. These morphotypes are related in three natural phenotypic groups that present morphological divergence and endemicity and are described as Mesoamerican-Caribbean lineage, Andean lineage and Brazilian lineage (Hernández-Ortiz et al. 2015). In relation to the Brazilian lineage, Dias et al. (2016) evaluated A. fraterculus populations from different regions and concluded that mitochondrial COI sequencing data, associated with their behavioral and morpho-metric data, may point out divergences and partial pre-zygotic reproductive isolation between populations from the South and Southeast Brazilian. On the other hand, the use of DNA barcode records for different species of Anatrepha is questioned by Barr et al. (2018) since DNA barcoding of the cytochrome oxidase I gene alone cannot diagnose A. fraterculus, Anastrepha obliqua (Macquart, 1835), and Anastrepha suspensa (Loew, 1862). Thus, the authors emphasize the importance of associating these resources to aspects such as the evaluation of the agricultural importance of the specimens included in the database so that they are biologically significant, as well as the proper interpretation of diagnostic measures for the reconstruction of phylogenetic trees or for obtaining diagnostic characters.Among the methods to control fruit fly, there are pesticides, the sterile insect technique (SIT) and biological control especially with parasitoids. For the SIT approach, sterile insects are produced in large numbers, sterilized in the pupal phase, and then released systematically into the field (Enkerlin 2005). Although most programs that integrate the SIT against fruit flies uses bisexual strains (Orozco-Dávila et al.2017), for some species, it is preferable to release only males (Walder 2000), however genetic sexing strains are available for a few species (McInnis et al. 2004). This technique is indicated for area-wide control of this pest (Hernández-Ortiz 2007). However, SIT relies on the sexual competitiveness of sterile males compared to wild males. Different studies address the performance of sterile males, evaluating behaviour such as emission of sexual pheromones, and biological, such as longevity and flight capacity, among others (Abraham et al. 2011; Arredondo et al. 2017; Gallardo-Ortiz et al. 2018). For A. fraterculus in field cage experiments it was observed that irradiated males were also able to modulate the behaviour of females as fertile wild males (Abraham et al. 2013). One of the major constraints of this technique is the separation of males and females in the stage of pupa. It is often difficult to find noticeable phenotypic (visual) differences between pupae of males and females. Even though the release of both sexes sterilized with gamma radiation is a possibility (Bakri et al. 2005), it is not sensible to release radiated females in the field, especially those that can make punctures to developing fruits, because puncture damage may occur. These damages caused by the oviposition puncture, even if the eggs do not develop, are registered for some fruit such as kiwi (Actinidia deliciosa ‘Hayward’) with the record of formation of a crystalline exudate in puncture sites that evolve at harvest to cracks, depressions and beginnings of galleries (Lorscheiter et al. 2012). Also, the oviposition wounds, besides inducing green grapes to drop prematurely, may also serve as a gateway to pathogens and in this way lead to additional production losses (Machota et al. 2016). The SIT is well developed to control C. capitata (Robinson 2002). This is because it is easy to distinguish male and female pupae of C. capitata through the use of genetic sexing strains that promote different colors between the sexes. A similar system was developed from a natural mutation of Bactrocera cucurbitae (Coquillett, 1849) pupae in which females were white (McInnis et al. 2004). Researchers at the Insect Pest Control Laboratory (IPCL - Joint FAO/IAEA) have been developing a genetic sexing strain (GSS) for A. fraterculus, that is based on a pupal color dimorphism (black-brown) (International Atomic Energy Agency 2020). However, there is still a domestication phase of the line before it can be used in mass rearing. For other Tephritidae genus and species, like Rhagoletis spp.and Anastrepha spp. SIT is more difficult to apply since it is currently not possible to distinguish the sexes in the pupa phase by a fast and non-invasive method. Optical image analyses could provide this fast, non-in-vasive and possibly cost-efficient method. Imaging systems based on RGB cameras can obtain phenotypic data within milliseconds, with high resolution and at low costs. However, the spectral range and resolution of these multi-spectral sensors might be a limiting factor. Hyperspectral imaging, in turn, collects and processes information from more than 100 wavebands across the optical electromagnetic spectrum (ultraviolet ~ 100 - 400 nm) to infrared (~ 700 nm to 1 mm) at all pixels of the image (Lillesand et al. 2004). Optical spectroscopy is widely used for qualitative and quantitative analysis. In particular, the absorption of light at specific wavelengths due electronic excitations and vibrations of functional groups and molecules can be related to the chemical characteristics of imaged targets (Goetz et al.1985). In general, spectra from the visible range (V, 400 nm to 750 nm) tend to contain less pronounced features due to the broad and overlapping bands of the absorption. Absorptions in the near-infrared region (NIR, 750 to 2,500 nm) result from the overtones of OH, SO4, and CO3 groups, as combinations of atomic vibrations of H2O and CO2. Mid-infrared spectra (2.5 to 30 μm) are related to the molecular fundamental vibration and these usually exhibit more detailed features (Leroy and Rancoita, 2011). However, due to the higher sensitivity to noise, the near-infrared is less frequently employed. Visible-near-infrared (VNIR) hyperspectral close-range imaging has been used widely in detection and characterization of biotic and abiotic stress factors in agriculture, including the detection of diseases like Fusarium spp. in wheat (Bauriegel et al. 2011) and detection of pests such as the wheat stem sawfl, Cephus cinctus Norton, 1872 (Hym., Cephidae) (Nansen et al. 2009), mites (Reisig and Godfrey 2007; Nansen 2012) and aphids (Reisig and Godfrey 2007). Nansen et al. (2014) showed that three species of parasitoids developing in host eggs (Ephestia kuehniella Zeller, 1879) (Lep., Pyralidae), could be classified based on spectral signatures of reflected light acquired from eggs. Teixeira et al. (2015) identified differences in strains of populations Grapholita molesta (Busck, 1916) (Lep., Tortricidae) collected in different regions of Brazil and Italy and said that the pupal stage was the most useful for the spectral reading. Near infrared spectroscopy has also been used to identify the stage of development of the Anopheles species (Sikulu et al. 2014) and Culicoides sonorensis (Wirth and Jones, 1957) (Dip., Ceratopogonidae) under laboratory conditions, seeking subsidies to speed up the characterization of the control programs for these groups of insect vectors of human diseases (Reeves et al. 2010). Intrapupal development of tse-tse fly was recorded by near infrared photography and video showing that NIR light passes the puparium allowing for the description of the processes until the pharate adult (Moran and Parker 2016).All works cited indicate that multi and hyperspectral imaging can provide relevant data to characterize arthropods. The objective of this research was to use spectral images to discriminate males and females of A. fraterculus in different stages of pupal development. These findings could form a basis for automatic classification of the sexes in lab rearing of A. fraterculus for SIT.
Materials and methods
Tephritidae pupae. Anastrepha fraterculus pupae, a Brazilian-1 morphotype of a laboratory strain from Tucumán, Argentina were obtained from fly breeding in the laboratory of the Insect Pest Control Laboratory (IPCL) from the FAO/IAEA Division of Nuclear Techniques in Food and Agriculture, IAEA Laboratories, Seibersdorf, Vienna, Austria. The pupae were 4.5 - 6.0 mm long and 2 - 2.5 mm in diameter. They were cylindrical, with coloring a dull luteous at first to reddish-yellow or dark brown. Pupae from the IPCL were placed in screened cages kept in controlled conditions (23 °C ± 1 °C; RH 60 % ± 10 % and 12 h photoperiod) until the adults emerged. Insect rearing was conducted in the laboratory of the Leibniz Institute for Agricultural Engineering and Bioeconomy (ATB). The population was kept under standard laboratory conditions, following the guidelines described by Dias et al. (2020). The next generation was used to obtain images from pupae. After the bioassay, adults from this generation were eliminated.The values of degree-days accumulated in the egg-adult period were determined as a function of the daily average air
temperatures by making the daily sum of thermal units (degrees-day) from the base temperature (Haddad et al. 1999), by the following relation:
𝐺𝐷= 𝑦 ∙ (𝑇−𝑇𝑏)
where, GD: thermal units accumulated per day; y: time to complete development (days); T: average daily temperature of the air; Tb 10.7 °C (base temperature for the egg-adult period of A. fraterculus according to Salles 2000). Thus, calculating the development time for an average temperature of 22.5 °C, from egg to the tested pupa phase, pupae were measured with circa 231 degree-days (DD), 288 DD, 345 DD and 403 DD of age for assessing different stages of development (30 pupae of each age).
Image acquisition
The strategy for image acquisition involved groups of five pupae with different development time (DD) placed on a piece of cardboard (3 x 1 cm) (RGB 0-140- 0). Images of the dorsal and ventral view were acquired for posterior analysis. The position and identification of each pupa were replicated for all experiments to allow further comparison (Fig. 1A).
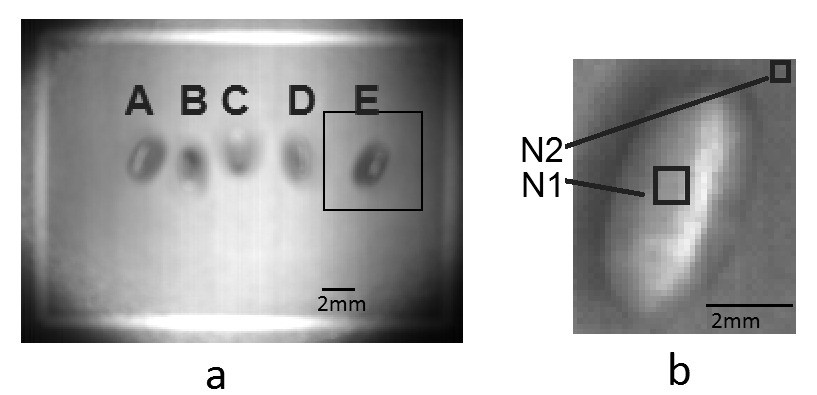
Figure 1 A. Placement and sequence (a-e) for identification of each pupa in the experiments. B. Detail showing normalization strategy by selecting rectangles over the pupa with few pixels out of the glint area (N1) and on the background area (N2).
2mm 2mm A E B A B N2 N1 C D Figure 1. A. Placement and sequence (a-e) for identification of each pupa in the experiments. B. Detail showing normalization strategy by selecting rectangles over the pupa with few pixels out of the glint area (N1) and on the background area (N2).
The hyperspectral measurement system, belonging to ATB image sensing laboratory, consisted of two spectrographs, ImSpector V10E (to VNIR, 200 nm to 750 nm) and ImSpector N17E (to NIR, 750 nm to 1,400 nm), (Specim Inc., Oulu, Finland), a monochrome camera (Pixelfly qe, PCO, Kelheim, Germany), a rotating mirror (adjustable with a microstep motor). A custom software coded in LabView 8.6© (National Instruments 1976) controlled the camera system and pre-processed the data including white-referencing and dark current removal. The system recorded spectra in the wavelength range of 380 - 1,700 nm. The spectral resolution for the 380 - 800 nm range was 2 nm and for 800 - 1,700 nm was 5 nm (Fig. 2).
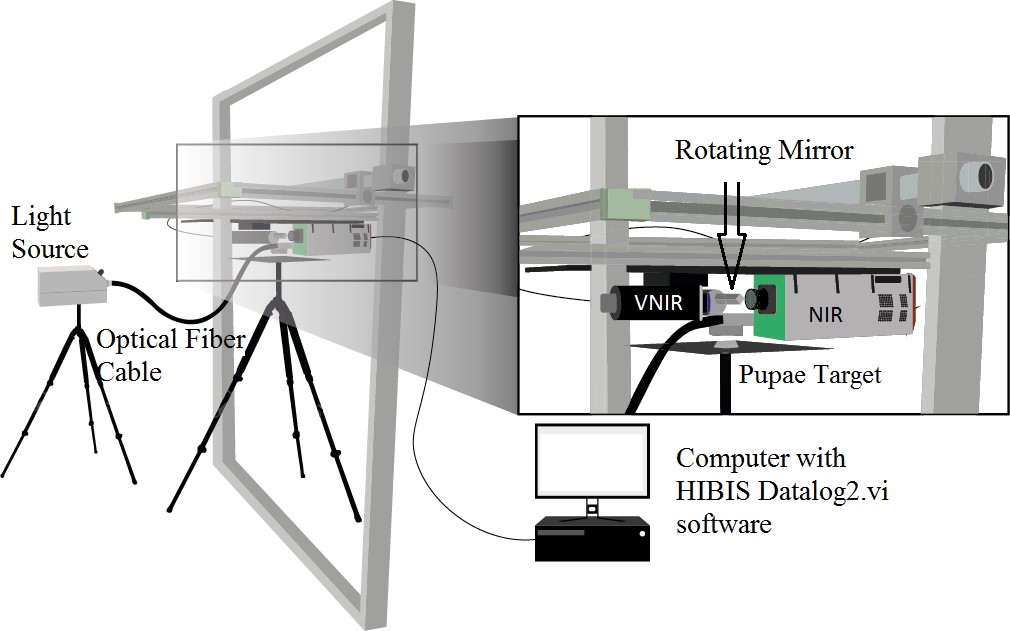
Figure 2 Schematic diagram of the hyperspectral imaging system: Spectrographs, ImSpector V10E (to VNIR, 200 nm to 750 nm) and ImSpector N17E (to NIR, 750 nm to 1,400 nm), Specim Inc., Oulu, Finland), a monochrome camera (Pixelfly qe, PCO, Kelheim, Germany), a rotating mirror (adjustable with a microstep motor).
Raw images were taken row by row. In a consecutive preprocessing step, the data were transformed to create a data cube containing the spatial information in the x-y-direction and the spectral information in z-direction with a band width of 1.2 nm. With each measurement, images for the dark current subtraction and the white referencing balance were recorded for posterior data calibration. To obtain shadow free viewing a ring light with a halogen lamp was used. After image acquisition, the pupae were individualized and placed in 100 mL plastic bottles labeled with the numerical identification and stored in a room at 23 ± 2 °C, 60 ± 10 % RH until the emergence and subsequent confirmation of the sex of the individuals.
Image preprocessing
The images of the groups were segmented, separating each of the pupae that are saved as a new image. A mask was applied to the background allowing the individual acquisition of the spectrum for each pupae. We performed the experiments with at least 30 pupae (15 males and 15 females) selected from different ages always at symmetric positions (e.g. Fig. 1 A a-e, b-d). Even with light controlled by a ring light, the near cylindrical geometry of the pupae favored glint reflection and slightly different illumination over each pupae surface. A lack of uniformity in the illumination caused high intensity at the center of the image, which gradually decreases as it approaches the corners of the selected area (Fig. 1 A). To suppress the radiometric differences within an image and between images (over time) due to different illumination and remaining noise after dark current subtraction, we applied the following procedure:
1. A small zone (about 5 by 5 pixels) from each pupae (N1 in Fig. 1 B) was selected and the measurements for each waveband were averaged. The zone was selected in such a way that light glint was avoided and a uniform region from the pupae’s surface was captured.
2. To obtain reference values for standardization purposes, small background samples close to each pupae were selected for further processing (N2 in Fig. 1 B).
3. Standardization was carried out by dividing the averaged measure over the pupae (N1) by the average of the background (N2) (Fig. 1 B). The resulting value N1/N2 is dimensionless but is theoretically free of any radiometric interference among measures.
The processing was restricted to the range of 400 to 650 nm for Vis and 1,000 to 1,600 nm for NIR since other regions of the spectra were obstructed by noise. Image noise is random variation of brightness or color information, in the images captured and it needs to be filtered before signal processing and image analyses (Trauth 2010). These regions are strongly affected by white noise and makes the signal to noise ratio very poor. So, we selected only region between 400 nm to 650 for visible and 1,000 to 1,600 nm in the NIR region, and the remaining was discarded from the analysis. Also, to avoid illumination discrepancies, comparisons were always made with pupae placed in the same position, or in symmetric position regarding illumination (e.g. Fig. 1 A a-e, b-d). It is important to consider the relation between the signal and the noise at the beginning and at the end of the measurements.
Radiometric analyses
When dissecting the pupae of ca. 403 DD, it was possible to notice some dark bristles on the lower portion of the female beneath the puparuim (Figs. 3A, 3B). This fact combined to the relative transparency of the puparium could indicate that we may find differences between sexes by tracing a spectral profile over the pupae. Thus, we also performed longitudinal measurements along the profile of pupae photos made by RGB camera to reveal radiometric variation along the male and female in an established direction (Fig. 3 C). Spatial standard deviation was calculated for exploratory purposes. It was computed by selecting uniformly distributed wavelength positions.
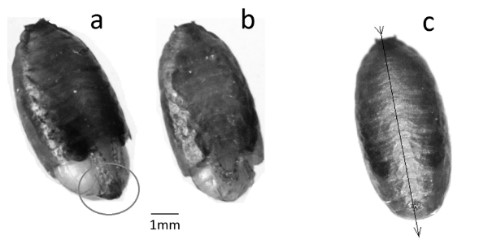
Figure 3 Dissected puparium of 403 DD, showing ventral part of: A. female with concentration of bristles at the end of the abdomen. B. male with abdomen without presence of dark bristles. C. puparium image made by RGB camera in ventral position. The black line and arrows indicate the direction of the profile.
Statistical analyses
After processing the images acquired as described above, the data were extracted and converted into numerical data. The total mean reflectance data for each group (male and female) were contrasted with the variables: VNIR (ventral view); VNIR (dorsal view); NIR (ventral) and NIR (dorsal) in a linear discriminant analysis (LDA). The average spectral variation data for each development time (DD) were plotted in relation to different wavelengths (nm) for VNIR and NIR in ventral view, which was more sensitive to capture the variations. Four bands which presented the greatest discrepancy between genders for the three ages (DD) evaluated, were selected and subjected to the Levene test followed by Welch (Levene 1960; Gastwirth et al. 2009). The data of radiometric variations of the ventral profile of the pupae in the RGB spectrum were adjusted in a linear Fischer’s discriminant regression (Fisher 1936) using the distance in pixels and the digital value of the blue channel to calculate the measure of the intercept for males and females from the top to the base of the pupa. The analyzes were made by the softwares: Bioestat 5.3© (Ayres et al. 2007), Past 4.03© (Hammer et al. 2001) and Matlab® (MATLAB 2010).
Results and discussion
Hyperspectral profile of different ages and sexes of pupae
The linear discriminant analysis (Fig. 4), which separates the groups according to the values of their variables in a linear manner, in this case in two dimensions, shows a tendency for the average data of the spectral profile of the males to be concentrated more to the right, while the female data are concentrated from the center to the left. The variables X1 (Fig. 4), which correspond to the images of the NIR spectrum in ventral view, for both females and males, have the highest estimated values in relation to Fisher’s discriminating functions and contribute more to the discrimination of groups. Thus, it is inferred that the images in the near-infrared spectrum of the ventral view of the pupae are the most suitable to discriminate males and females. Apparently, there is a diagonal separation (northeast direction), but there are outliers, which do not allow a definitive statement. These outliers are data that differ dramatically from others, being points outside the normal curve and may be related to phenotypic characteristics or to other undetermined variables.
The mortality of the 231 DD pupae exposed to the images was very high (about 90 %). As such it was not possible to obtain enough data for a reliable analysis and thus, this stage had to be excluded. Sensitivity to light is probably the factor responsible for this high mortality. In the natural environment, A. fraterculus larvae are protected from direct light since they remain inside the fruits while the pupae are generally buried in the soil (Salles 2000). The lethal effect of specific wavelengths for immature stages of insects has been reported for drosophilids, mosquitoes and flour beetles. Wavelengths from 417 nm to 466 nm had a lethal effect of approximately 60 % to 90 % on the pupe of Drosophila melanogaster Meigen, 1830, at early pupal development (Shibuya et al. 2018). The authors, however, report that this mortality decreases to less than 10 % in the last pupal stage.
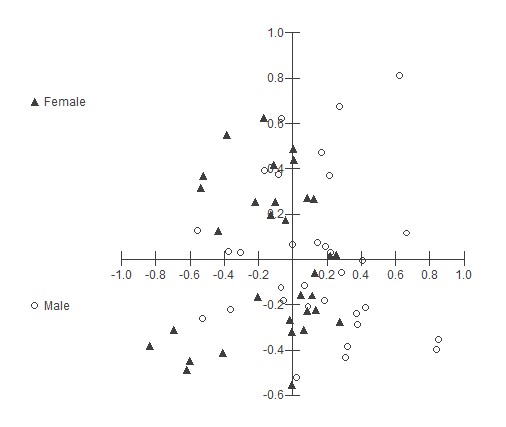
Figure 4 Scatter plot of discriminant analysis. Groups related to pupae of Anastrepha fraterculus males and females; variables referring to the total mean reflectance data obtained by NIR (ventral); NIR (dorsal); VNIR (ventral view) and VNIR (dorsal view) in a discriminant analysis. Fischer’s discriminat regresions were indicated.
Hyperspectral imaging data contained both spatial and spectral information from pupae, each pixel on pupae corresponded to one spectrum. The average spectra of A. fraterculus pupae were obtained by choosing 5 x 5 pixels of each pupae around the center of the ventral and dorsal side of the pupae. The average spectral curves of 288, 345 and 403 DD showed differences, indicating that the pupal hue, and therefore its reflectance, is altered throughout the development (Fig. 5).
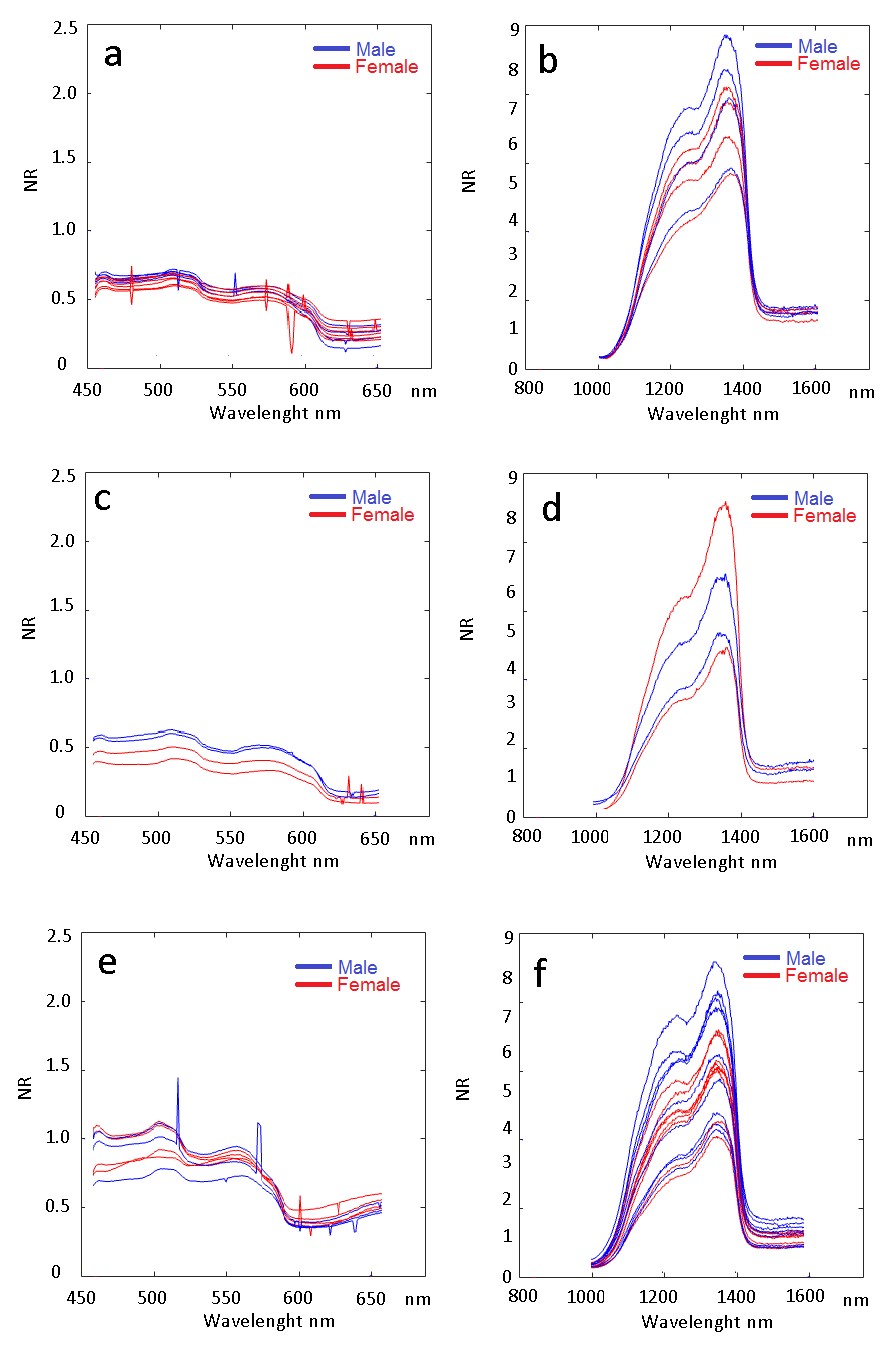
Figure 5 Spectral variation along the analyzed spectrum of pupal stage of Anastrepha fraterculus. Blue lines are referred to male whereas red refers to female pupae. A. VNIR 288 DD. B. NIR 288 DD. C. VNIR 345 DD. D. NIR 345 DD. E. VNIR 403 DD. F. NIR 403 DD.
Comparing the VNIR images, it was observed that at age 288 DD, the entire spectral profile, from 400 to 680 nm (excluding noise), reflectance ranges from 0.12 to 0.70. For the age of 345 DD, it ranged from 0.2 to 0.75, and for 403 DD, 0.42 to 1.23 (Fig. 5). However, in the visible range, age discrimination was not visually noticeable, and it was also not possible to discriminate males from females as there is a lot of variation in the averages resulting from intraspecific differences. The differences between the ages in degree-days, especially identifiable in the VNIR spectrum between the last moments of pupal development are due to the pigmentation processes, especially of the wings and eyes of the flies. This process was described by Fukutomi et al. (2017) for Drosophila guttifera Walker, 1849 as one that discriminates periods of development of pupae with pigmentation of eyes, wings and bristles from melanin precursors such as dopa and dopamine. Considering that the genus Anastrepha also presents colored bands in the wings and eyes with color variation (Zucchi 2000), it is possible that similar processes occur in the development of their pupae. In the NIR spectrum (800 to 1,700 nm), a higher reflectance between 1,150 and 1,380 nm wavelength was observed. In these bands it is possible to observe differentiated variations of reflectance between males and females for each of the evaluated ages (Figs. 5B, 5D, 5F). For males at the onset of pupal development (up to 288 DD) and at the end (403 DD), the reflectance was higher than for females, on average, whereas for females, this was higher in the intermediate period (Fig. 5). These changes in spectral profiles throughout development can probably be related to the morphogenesis of the species. Ma et al. (2015), for example, describe differences in color during the morphogenesis of the pupal phase of Chrysomya rufifacie (Macquart, 1844) (Diptera: Calliphoridae), indicating differences in structures that darken unevenly throughout the pupal period.
It is important to remember that at the end of the larval stage, larval skin transforms to pupal sheath (puparium), initiating the pupal-larval apolysis. After the apolysis is complete, pupal morphology gradually changed with time (Pujol-Luz and Barros-Cordeiro 2012). Puparium pigmentation may also change. Sometimes the change is very visible, as in the case of Sarcophaga dux (Thomson, 1869) (Diptera, Sarcophagidae) in which the puparium goes from creamy white just after apolysis, to dark brown or black before adult emergence (Sinha and Mahato 2016). In the case of A. fraterculus puparium, the difference is barely visible.
The selection of equally distributed regions or bands of the spectral profile of males and females of different ages improved the differentiation between stages of development and sex. The wavelength bands of 1,000 nm, 1,200 nm, 1,400 nm and 1,600 nm were selected to describe the variations in the standard deviation of the spectra (Fig. 6). The intervals were uniformly chosen to represent the different regions of the spectrum. With this analysis it was possible to confirm that the alterations of the profile occurred in males and females during different pupal stages of development.
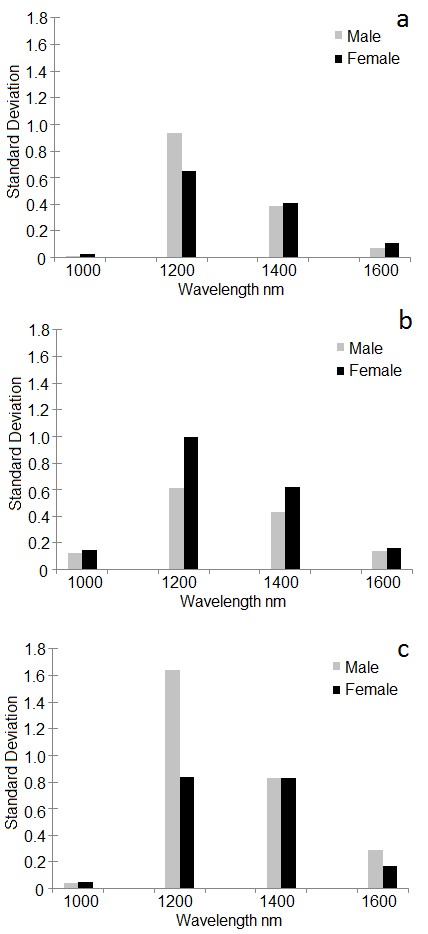
Figure 6 Standard deviation in some regions of NIR of pupal stage of Anastrepha fraterculus. Gray bars refer to male whereas black bars refer to female pupae. A. 288 DD; B. 345 DD. C. 403 DD. * significant differences based on Levene’s test for homogeneity followed by Welch test (F = 4.83, df = 12.93, P = 0.04479).
There were differences in the standard deviation of the reflection values according to the age (degrees of pupal development). The greatest differences between males and females observed at all development times were found at 1,200 nm in ventral view. The deviations between males were higher than for females. This contrast may be due to differences in the pigmentation of the body of pupae of males and females since NIR light passes through the pu-parium, permitting images of the exarate pupae (Moran and Parker 2016).
Consequently, future work can focus on this wavelength range. The difference in reflectance at 1,200 nm at 403 DD of age was the one that discriminated best between the sexes (Fig. 6 C). Thus, images can be made focusing on this range of spectrum to seek a definitive difference. The analyses of standard deviation could be considered an exploratory approach to identify differences on development time and sexual dimorphisms of pupae, that are expressed in the reflectance difference of the body.
RGB data
The difference between the target object, in our case the pupa, and the background was easily observable in this type of analysis (Figs. 7A, 7B). Actually, RGB image analysis has been used in different studies to characterize, classify and quantify insects in traps (Ding and Taylor 2016). Miranda et al. (2014) also used background modeling to detect the presence of insect pests in the captured image.
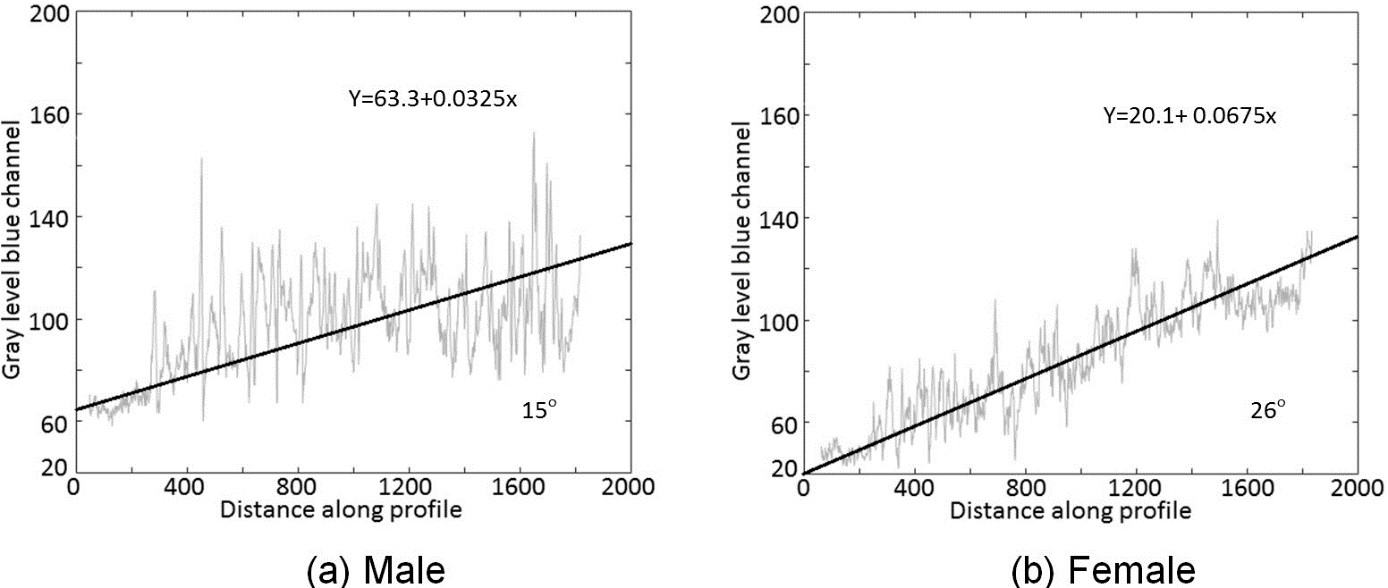
Figure 7 RGB radiometric variations along the pupa in ventral position, in the blue channel of the RGB camera. A. Male. Degree of variation from top to basal part of male pupa in blue channel. B. Female. Degree of variation from top to basal part of female pupa in blue channel.
In the analysis of texture, with a cut of the ventral image with a few hundred pixels it was not possible to identify differences between males and females in our study. Our findings differed from the results of Byers (2006) who used the standard deviation, derived in a similar way, to capture differences between male and female abdomens of Lygus hesperus Knight, 1917 (Heteroptera: Miridae).
Thus, we tried another approach considering that at the end of pupal development the reproductive organs are formed. In this period, it is possible to observe that in the areas near the ovipositor (not yet distended) the bristles depict a darker result due to the dark bristles, even beneath the semitransparent shell (Fig. 3 A). In fact, we observed some differences between sexes by evaluating the spatial RGB reflection profile from the head to the anal area. In an exploratory analysis, we observed that, in general, the spectral radiance increased as we proceed to the anal area. It was observed that spectral radiance of the male pupae was systematically higher than for females (Fig. 7) which may indicate an important parameter to differentiate the sexes.
However, analyzes of these images can be even more detailed, pointing out differences in the sex of the same species. The high spatial resolution of the RGB camera could reveal in detail the radiometric variations along the male and female in an established direction (Fig. 3). By fitting a linear regression model using the distance (in pixels) as the regressor and the blue value (digital number) as the regress and the spatial radiometric variation angle from the top to the basal part of the pupae can be derived. The example of the images (Figs. 7A, 7B), shows, that the angle was greater (26°) for the female than for the male (15°), moreover, the measure of the intercept for male is 63.3 and for female, 20.1. Likewise, the differences in the linear regression trend line angle and measure of the intercept for males and females may be a tool used to discriminate the sexes at other stages. The choice for linear regression was made by prudence, as we have no complete idea of the forcings that influence the color variation along the profile. The difference between the angles of radiometric variations along the profile may also be used to define contrasts between males and females at this stage of development. In general, 403 DD of age is the moment in which pupal sterilization occurs for posterior release of sterile males on fields, as it results in higher numbers of flyers (Walder 2000). To A. fraterculus this is interesting because populations of mass rearing that have their origin in the southern region of Brazil, as in our study, sex may have discriminated before irradiation and later males can be used in releases that cover a wide area, from Buenos Aires, to near São Paulo. This is because populations within this range are sexually compatible and could be managed using a single A. fraterculus mass reared strain (Rull et al. 2012).
Conclusions
In this paper the potential of hyperspectral and photographic images was assessed to provide differentiation between male and female pupae of A. fraterculus. We conclude that hyperespectral images of the pupal infrared spectrum, as well as the study of radiometric variations, can be a viable non-destructive method to detect differences in sex and time of pupal development of A. fraterculus and other species of Tephritidae as a basis for mass production and sterilization. Some slight differences occur in certain spectral channels and at some ages (DD), and further tests can be carried out in order to explore those differences. In particular, the use of RGB cameras along with image processing can be a very cost-efficient approach which should be further evaluated.