Introduction
Echinochloa crusgalli L. var. mitis (barnyard grass) is one of the main weeds occurring in rice fields, distributed worldwide. Barnyard grass presents serious problems of herbicides resistance in several places in the world like Asia and the United States (Zhang et al., 2017). The continuous use of herbicides with the same mechanism of action, associated to the absence of crop rotation and high populations of barnyard grass, has allowed the evolution of resistance of this weed to several products. In some cases, multiple resistance has been characterized (Molin et al., 2016). Propagation of resistant biotypes can be accelerated by pollen-mediated gene flow, transferring resistance alleles to previously sensitive populations (Yu et al., 2010).
In irrigated rice crops in southern Brazil, imazapyr and imazapic are widely use as herbicides and the lack of herbicide rotation in treated areas has favoured the selection of resistant barnyard grass, which was confirmed in a previous study with E. crusgalli var. mitis biotypes from Pelotas, Rio Grande and Arroio Grande in Rio Grande do Sul state, Brazil (Bonow et al., 2018).
Resistant weeds can survive herbicide application via a variety of mechanisms, divided into two categories: target-site resistance (TSR) and non-target-site resistance (NTSR) (Délye et al., 2013). TSR mechanisms include increased expression of the target protein resulting from regulatory mutations causing target protein overproduction that compensates for the herbicide inhibitory action, and or structural changes to the herbicide binding site that modify the structure and electrochemical properties of the target protein. NTSR mechanisms include the reduction in herbicide penetration due to alterations in cuticle properties and plant growth characteristics; sequestration and altered translocation of the herbicide away from the target protein; enhanced degradation (metabolism) of the herbicide and enhanced neutralization of cytotoxic molecules generated by herbicide action (Délye et al., 2013).
Weed biotypes with resistance to ALS inhibitors have mainly alterations in the herbicide target enzyme resulting from mutations in the corresponding gene or, more rarely, increased herbicide metabolism in the plant (Yu and Powles., 2014). Nowadays, with advances in the NTSR resistance studies, it is believed that some resistant species may in addition to TSR, have metabolism as a mechanism causing resistance (Délye et al., 2013). Given the complexity with polygenic inheritance, studies involving NTSR are still in progress.
The resistance of weeds caused by metabolization is an important mechanism, since it allows a plant to become resistant to herbicides of different mechanisms of action and even to products never used before to control the species in an area (Powles and Yu, 2010). It occurs from increased rates of metabolic detoxification of the herbicide. This ‘protection’ is performed by certain enzymes and other plant defense proteins, mainly of cytoplasmic location, with a wide range of specificity (Hatzios and Penner, 1982).
Herbicide metabolism in plants occurs in three phases, the first being the detoxification performed by enzymes called cytochrome P450 mono- oxygenates (cyt-P450), which alter the structure of the herbicides by reduction, hydrolysis, hydroxylation or dealkylation of the molecule; this makes the herbicide molecule more reactive (Powles and Yu, 2010). In the second phase of metabolization, the conjugation of the altered molecules with glucose, glutathione, amino acids, proteins or other organic compounds, through enzymes such as glycosyltransferases and glutathione S-transferases (GSTs), form stable, polar (S- glutathione) and less toxic compounds (Yuan et al., 2007; Ogliari et al., 2009). In the third and last stage, transport of these conjugated molecules to the extracellular space or the vacuole can involve ABC transporters (Yuan et al., 2007).
Inhibition of enzymes that act on the cyt-P450 complex by the application of malathion and amitrole insecticides has served as an indirect way of investigating whether metabolism of the herbicide is involved as a mechanism of resistance. The inhibitor 1-aminobenzotriazole (ABT) confers metabolic resistance of Bromus tectorum to chlortoluron herbicide via cyt-P450 (Gorinova et al., 2014). Echinochloa phyllopogon biotypes were also identified with metabolic resistance to penoxsulam after previous application of malathion (Yasuor et al., 2009).
The hypothesis of this research is that barnyard grass biotypes resistant the herbicide mixture of imazapyr and imazapic by enhanced metabolism. Knowledge of a mechanism of low-level resistance in barnyard grass to this herbicide mixture is necessary, in order to establish management strategies in irrigated rice crops.
The objective of this study was to verify if such mechanism is involved in the resistance of Echinochloa crusgalli var. mitis biotypes to ALS inhibitor herbicides.
Material and methods
A study was conducted by duplicate in a greenhouse belonging to Embrapa Clima Temperado, at the Terras Baixas experimental station, Capáo do Leáo-RS (31°80’S e 52°40W, 13 m.a.s.n.l) during the period from December 2014 to March 2015. The experimental was completely randomized design (CRD), with four replications. The climate of the region, according to the climatic classification of Wilhelm Koppen (1930) is Cfa (C: hot mesothermal climate, with average cold months between 3 and 18 °C; f: average monthly rainfall of not less than 60 mm - always humid; and a: temperature of the hottest month above 22 °C), with average temperature of 26 °C during the conduction of the experiment.
Seeds of E. crusgalli var. mitis biotypes were collected from plants in rice fields in southern Brazil and then cleaned, dried (13% humidity) and stored at room temperature until the test was initiated. Seeds were sown in trays and transplanted to plastics pots, with a volumetric capacity of 0.5 l. Plants with 3 to 4 expanded leaves were sprayed with imazapyr + imazapic (73.5 + 24.5 g a.e. ha-1; grams of acid equivalent per hectare), aiming to screen for herbicide resistance (Table 1) (Bonow et al., 2018. The plants that survived the applied dose were cultivated in plastic buckets for one generation in a greenhouse until the production and collection of seeds, avoiding outcrosses by isolating the plants in separate greenhouses. After harvesting the seeds, they were subjected to dormancy (Brasil, 2009).
Table 1 Location and characterization of Echinochloa crusgalli (barnyardgrass) var. mitis in response to the application of imazapyr+imazapic (73.5+24.5 g a.e. ha-1), visually assessed at 28 days after application. Capao do Leao/RS, Brazil.
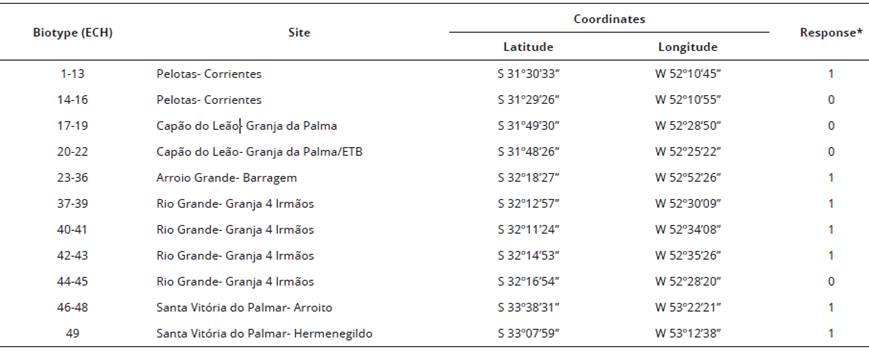
*Biotypes selected according to the response (visual) 0 = susceptible and 1 = resistant that exhibited the following responses to imazapyr+imazapic (73.5+24.5 g a.e. ha-1): ECH1 (Biotype 1) = 10% control., ECH14 (Biotype 14) = 98% control; ECH27 (Biotype 27) = 10% control; ECH38 (Biotype 38) = 5% control and ECH 44 (Biotype 44) = 90% control.
In the same year, seeds harvested of each biotype were sowed in plastic trays and the seedlings transplanted to plastic containers of 0.7 L containing in soil classified as an Albaqualfs (Soil Survey Staff, 2014), or Planossolo Háplico Eutrófico Distrófrico (Santos et al., 2013). Experimental units were composed of one plant per pot. Soil pH in water was 5.0; cation exchange capacity, CEC pH7 = 7.1 cmolc dm-3; organic matter = 1,8%; clay = 14%; texture = 4 (Santos et al., 2013); Ca = 4.1 cmolc dm-3; Mg = 1.1 cmolc dm-3; Al = 1.6 cmolc dm-3; P = 6.5 mg dm-3 and K = 0.15 cmolc dm-3. Fertility was corrected according to recommendations for irrigated rice (Sosbai, 2018). The resistance factor (RF) of the biotypes was determined in a parallel experiment in the 28 days after treatment (DAT) (Bonow et al., 2018), where the dose causing 50% of plant mortality of the resistant biotype (C50) was divided by the dose that causes 50% of mortality to susceptible plants. However, it was not possible to determine RF for ECH38, as it did not reach such control level. The RF was 15.2 or 10.2 for ECH1 and 27.9 or 18.6 for ECH27, in division with the susceptible biotypes ECH14 and ECH44, respectively.
Treatments for metabolization experiment were arranged in a factorial scheme; factor A corresponded to susceptible (S) biotypes (ECH14 from Pelotas and ECH44 from Rio Grande) and resistant (R) biotypes (ECH1 from Pelotas, eCh27 from Arroio Grande and ECH38 from Rio Grande) to imazapyr + imazapic; factor B consisted in application or not of the insecticide malathion or piperonyl butoxide (PBO), as inhibitors of cyt-P450 monooxygenases. Treatments were applied 20 days after sowing (DAS) when plants were in a three to four-leaf stage. Sixty minutes before the application of the herbicides (73.5 + 24.5 g a.e. ha-1), malathion (1000 g a.i. ha-1; grams of active ingredient per hectare) and PBO (525 g a.i. ha-1) were sprayed to plants (Yu et al., 2009). Spraying was carried out in an open environment and after 24 hours they returned to the greenhouse to resemble field conditions, using a backpack sprayer delivering 140 l. ha-1 at 210 kPa.
Visual evaluations were done 14 and 28 DAT, and shoot dry mass was determined after collecting aerial part of the plants, drying in a forced air circulation oven at 60 °C and weighed 28 DAT. The control assessment was performed individually at each plant, using a percentage scale with severity score of phytotoxicity symptoms every 5%, where 0% (representing no effect of herbicides on plants) to 100% (plants total death).
Data obtained was analysed for normality and homoscedasticity, with posterior analysis of variance (P<0.05). Duncan test (P<0.05) was carried out using Assistance Assistat, version 7.7 en.
Results
The significant interaction (P<0.05) among studied factors for visual evaluation at 14 and 28 DAT (Table 2) and also for shoot dry mass (Table 3) was verified. At 14 DAT, it was observed that the control for the resistant biotype ECH1 was the same whether herbicides were applied with or without the inhibitors (Table 2). Also, inhibitors did not enhance control of ECH38. Herbicide control of ECH27 with addition of malathion and PBO was increased by 96% and 73%, respectively.
Table 2 Control (%) at 14 and 28 days after application (DAA) of resistant (ECH1, ECH27 and ECH38) and susceptible (ECH14 and ECH44) Echinochloa crusgalli (barnyardgrass) var. mitis biotypes to the imazapyr+imazapic (73.5+24.5 g a.e. ha-1), applied alone or preceded by the application of malathion (1000 g a.i. ha-1) or PBO (525 g a.i. ha-1. Capao do Leao/RS, Brazil.
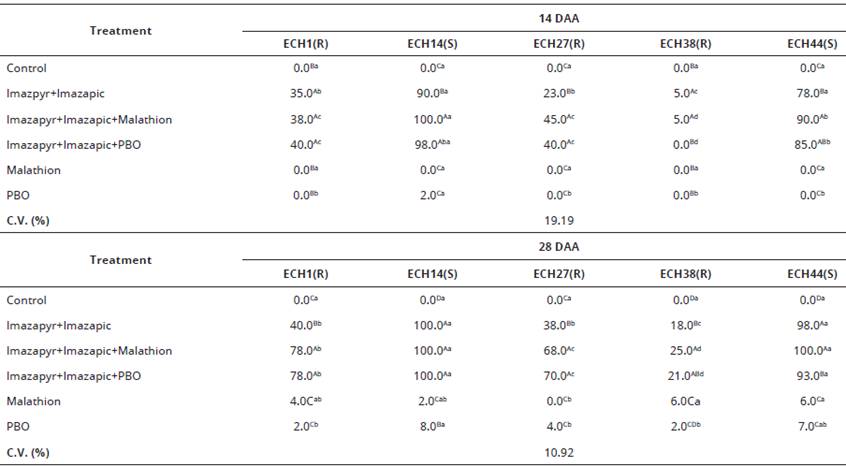
*1) Averages followed by the same capital letter in the column and lowercase in the row do not differ significantly by the Duncan test (P<0,05).
Table 3 Shoot dry mass 28 days after application (DAA) of resistant (ECH1, ECH27 and ECH38) and susceptible (ECH14 and ECH44) Echinochloa crusgalli (barnyardgrass) var. mitis biotypes to the imazapyr+imazapic (73.5+24.5 g a.e. ha-1), applied alone or preceded by the application of malathion (1000 g a.i. ha-1) or PBO (525 g a.i. ha-1. Capao do Leao/RS, Brazil.
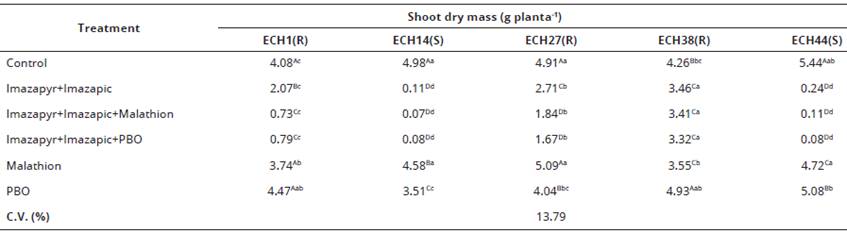
*1) Averages followed by the same capital letter in the column and lowercase in the row do not differ significantly by the Duncan test (P<0,05).
At 28 DAT, the results have further evidenced the action of inhibitors on plants (Table 2). Control treatment ECH1 was controlled by 78% with a previous application of either inhibitor, and was higher than the result obtained with herbicide applied alone (40%). Similar results were observed for ECH27 whose control was enhanced by 68% and 70% for malathion and PBO, respectively and in contrast to the application of imazapyr+imazapic alone (38%). Susceptible biotypes (ECH14 and ECH44) were, as expected, controlled by almost 100% in all treatments. For the resistant biotype ECH38, application of the herbicide associated with the inhibitors did not provide satisfactory control, reaching a maximum control level of 25% 28 DAT. Visual assessment of control 28 DAT with malathion, exhibited a slight but significant enhancement compared to the herbicides alone (Table 2).
Shoot dry mass results (Table 3) presented significant dry matter reduction when the inhibitors preceded herbicide application compared to application of the herbicides alone on the resistant ECH1 and ECH27 biotypes.
Discussion
Resistant biotypes ECH1 and ECH27 presented a significant increase in the control by imazapyr + imazapic as observed when using the inhibitors malathion and PBO, possibly related to the blockage of cyt-P450 activity, which is essential in the metabolism of several substances (Zhu et al., 2008). For Echinochloa phyllopogon, several genes related to multiple resistance involving the action of cyt-P450 such as CYP7IC35, CYP8IA12, and CYP89B16, have been identified, which are highly expressed in resistant plants (Iwakami et al., 2018).
Resistant ECH1 initially did no exhibit increased control at 14 DAT from inhibitors action. It is possible that response may vary over time among plant subjected to the application of the inhibitors for certain herbicidal molecules (Yasuor et al., 2010), however, at 28 DAT, increased control was observed. For resistant biotype ECH38, application of herbicide and inhibitors did not presented satisfactory control, reaching a maximum control level of 25% at 28 DAT (Table 2), which indicates that there is the possibility of another resistance mechanism present at this point, since for E. crusgalli the resistance to imazethapyr is partially reversed with the application of PBO or malathion at a dose of 1000 g a.i. ha-1 for both (Dalazen et al., 2016). This dose was not applied in the study in question for PBO, but Dalazen et al. (2018) found that the gradual increase in the dose of the inhibitor does not increase control response for plants resistant to ALS by metabolization in barnyard grass.
According to Matzenbacher et al. (2015) resistance to imazethapyr in barnyard grass populations is associated with ALS mutations suggesting a change in the site of action as a resistance mechanism. This, together with previous identification of metabolization of herbicides by barnyard grass, indicates high complexity of herbicide resistance in this species. Other studies have found that such complexity significantly restricts the number of active ingredients that can be used effectively for control of this weed (Muthukumar et al., 2017; Dalazen et al., 2018). Eberhardt et al., (2016) evaluated the response of E. crusgalli to different herbicides in the state of Santa Catarina that had difíiculties to be controlled with registered herbicides. The biotype showed cross resistance to the herbicide imazapyr + imazapic and penoxsulan, both inhibitors of the ALS enzyme. However, it was also resistant to ACCase-inhibiting herbicides (cyhalofop-butyl (360 g a.i. ha-1) and quinclorac (500 g a.i. ha-1), thus characterizing multiple resistance.
In summary, two (ECH1 and ECH27) of the three biotypes resistant to the herbicides imazapyr + imazapic have shown resistance possibly due to enhanced metabolism. Similar results were found for E. phyllopogon, where the application of malathion followed by application of bispyribac-sodium to resistant plants, resulted in a decrease in shoot dry mass of up to 31% beyond the obtained without malathion, suggesting enhanced metabolic herbicide degradation contributed to this weed specie resistance to herbicides (Fischer et al., 2000).
Resistance to herbicides caused by NTSR, mainly by the increase of metabolization of herbicides belonging to the class of imidazolinones is highly problematic due to the possibility of multiple resistance involving herbicides with other mechanisms of action. According to Bonow et al. (2018) resistant barnyard grass biotypes can be controlled with ACCase inhibitor herbicides such as cyhalofop-butyl and profoxydim in irrigated rice; or by glyphosate in the case of rotation with soybean genetically modified for resistance to that herbicide (RR) and clethodim. However, ECH27 and ECh38 exhibit multiple resistance to quinclorac (Bonow et al., 2018), and no further control with this herbicide is possible in irrigated rice. Therefore, NTSR is a new challenge for the management of weeds such as barnyard grass, because it may involve resistance to other herbicides registered for irrigated rice.
The observed results reinforce the importance of the practice of the integrated management aiming at the sustainability of rice cropping. The sole dependence on chemical tools to control unwanted weeds has already proven not
viable (Abbas et al., 2017). Producers need to be aware that using herbicides with alternative mechanisms of action may be helpful, but repeated herbicide use may select for resistance in the future. It is essential to implement crop rotation enabling the use of different herbicides, practices to reduce seedbanks, and avoid new seed immigration in the field. This can be challenging, but prevention has always been and will be a cheaper and more effective way of managing and delaying the evolution of herbicide resistance (Abbas et al., 2017).
Conclusions
Biotypes of E. crusgalli var. mitis biotypes from Pelotas (ECH1) and Arroio Grande (ECH27) have evolved resistance to imazapyr + imazapic due to enhanced metabolization of the herbicides as suggested by the partial reversion of resistance using known P450 inhibitors. Resistance of the ECH38 biotype from Rio Grande may presumably involve a different resistance mechanism.