Introduction
Forage grasslands are the main source of ruminant feeding in the tropics (Estrada et al., 2015). However, perennial grasses (Poaceae) and legumes (Fabaceae) can be affected by weather and rainfall. Nowadays, much of the literature emphasizes that rainfall is the most critical condition to produce dry matter (DM) (Perera et al. 2019; Norman et al. 2021). Widely evidence suggests that plant fertilization is a key to reaching high-level production (Martínez-Dalmau et al., 2021). Hence, studies argue the needs for better nutrient management and planning to recover the rates after harvesting or grazing (Norton et al., 2013; Teague et al., 2013). Specifically, nitrogen (N) is an essential compound for constructing amino acids, proteins, and chlorophyll (Islam et al., 2022). Moreover, it is responsible for the development of roots and the proper establishment of pastures (Sakiroglu et al., 2020). A possible way to achieve good levels of N in soil is through the biological fixation by specialized microorganisms or abiotic fixation (Hodge, 2005; Prosser, 2005). Because of the scarce N supply on both fixation ways, N inorganic fertilizer input is an important source of N for plants.
Likewise, soils play an important role in water- nutrient retention, carbon (C) reservoirs, and agricultural productivity. However, Oxisols (which represent 23 % of the soils in the intertropical zone) and Ultisols (18 %) are predominant in the island of Puerto Rico and are widely used as pastures for livestock; these soil orders are equivalent to 10.8 % and 19.8 % of the area of Puerto Rico, respectively (Muñoz et al., 2018). Also, 2.1 % of the total land area is used for hay and grazing forages (USDA, 2014) These predominant soil groups are highly weathered soils with low fertility, as well as naturally acidic and highly erodible. Additionally, these soils have a big lack of N. Furthermore, their intensive use also causes deterioration of their physicochemical properties such as compaction, and decreases cation exchange capacity (Beinroth, 2000). This situation justifies the use of N fertilizer, alternative fertilizers, and more productive grasses to improve soil fertility and yield production.
Tropical perennial grasses, such as the Megathyrsus spp. (cv. Mombasa), have a high breeding potential for digestible energy. In Puerto Rico, local guinea grass at 50-d harvest reported crude fiber of 36.9 % (Vicente-Chandler et al., 1964). Likewise, in a Latossolo Vermelho-Amarelo distrófico in Matto Grosso, Brazil, 100 kg of urea-N ha-1 was effective increasing the mean of dry matter yield (DMY) by three consecutive harvests of 3399, 1856, and 1488 kg ha-1, which was significantly different (Olivastro et al., 2018). Thus, rates from 60 to 100 kg N ha-1 every 40-45 days increased CP (> 7 %) and leaf-stem ratio. With a total rate of 180 kg N-urea ha-1, DMY was on average 5704 kg ha-1 when harvested every 40-45 days in the established year (Hare et al., 2015). Mombasa grass is of great relevance for tropical agriculture systems due to its high biomass production and nutritional value (Caldas et al., 2020).
On tropical soils, nutrient release may be limited by microbial flora since their interaction with the decomposition of organic matter (OM) and enables nutrient mineralization and its accessibility to forages (Zhang et al., 2021). For this reason, a microbial catalyst (MC) is of great interest, because an improvement in the microbial population is expected. A rapidly absorbed N fertilizer such as liquid urea (LU) contributes to the nutritional value and vigor of regrowth, in addition to root growth in tropical forages. Afterall, low losses under field conditions of liquid urea fertilizer are expected to mitigate ammonia losses and increase nitrogen use efficiency (NUE) and crop yield.
Urea fertilizer undergoes hydrolysis after its application and reacts under the presence of the urease enzyme. Then, ammonification process releases NH4+ and, by nitrification, produces n03 - ions; finally, after plant uptake, the N source presents losses by volatilization (Sun et al., 2008) Mariano. et al. (2019) found that urea remained on the soil surface four to six days after fertilization, thus reducing ammonia losses. Galindo et al. (2017), 2018) found advantages when 300 kg N ha-1 was used because N fertilization is more efficient and does not cause damage at high doses (1200 kg N ha-1) in the tropics. At low dosages, Castagnara et al. (2011) observed that Mombasa treated with doses ranging from 40 to 160 kg urea-N ha-1 showed acceptable CP, NDF and ADF percentages (8.7 %, 73 % and 42 %, respectively).
N fertilization has been limited or non-existent due to prohibition and high costs of N fertilizers in Puerto Rico. However, little is known so far about the use of LU as N fertilizer or MC and its effects on the yield of cv. Mombasa. For the tropical and sub-tropical area, the use of these nutrient sources would assist in the adoption of a better N fertilizer use management. The LU will be potentially useful in N plant nutrition with positive effects on shoot and root biomass and shoot nutritional value. In this respect, further research has suggested that LU or urea and micronutrients improve shoot biomass production and increase the nutritional value (Barros et al., 2018; Caldas et al.,2020;de Oliveira et al., 2020; Escarela et al., 2017; Galindo et al., 2017). The aim of this study was to compare the effect of a MC and LU on dry matter yield (DMY) and the nutritional value on five-week regrowth of well-established stands of Mombasa from March to October 2019, as well as to determine the biomass of root tissues, the percentage of soil organic carbon (OC) and soil nutrients.
Materials and methods
The study was conducted at the Agricultural Experiment Station of Isabela (AES) (17° 27’ 32’’ N and 67° 02’ 53’’ W; average annual rainfall of 1451 mm) of the University of Puerto Rico, located in Mayagüez, northwest of Puerto Rico. Well-established Mombasa on an Oxisol of Coto series (very fine, kaolinic, isohyperthermic, Typic Hapludox) (Muñoz et al., 2018) was used for this study from March 18 to October 28, 2019. The climate was tropical humid (data provided by AES) (Table 1).
Table 1 Mean monthly rainfaLL (mm), minimum, medium and maximum temperature from March to October, 2019 at the Agricultural Experiment Station Isabela, Puerto Rico
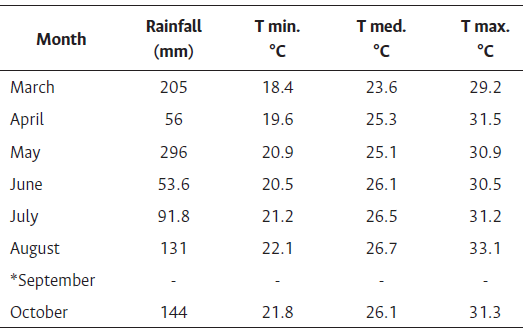
*Data not avaiLabLe. T min.: minimum temperature, T med.: medium temperature, T max.: maximum temperature.
The experimental design was completely randomized. Mombasa crop was established on October 31, 2018, and a standardized cut (10-cm height) was conducted on March 18, 2019, using a flail-chopper. A 40 x 37 m plot area was digenervided into 16 experimental units. Treatments: control (without liquid urea or microbial catalyst), microbial catalyst (MC) (Table S1), liquid urea (Lu) (Table S2) and MC+LU were randomly assigned to each plot in split applications (on March 19 and July 13, 2019). Both applications of LU were of 84 kg N ha-1 yr-1; both applications of MC were of 1.0 L ha-1; while the MC+LU consisted in two doses applied through foliar spraying for each experimental unit. Four of the experimental units were left as control. Treatments were applied on the same day of the standardized cut and after the 3rd harvest. The experimental variables measured were DMY, ADF, NFD and CP in the aboveground biomass and DMY and dry matter percentage (DM %) in root biomass for each treatment after the sixth 5-week harvests, as well as micro and macronutrients, pH, CEC and OM on soil samples.
Table S1 Property specifications of Microbial catalyst
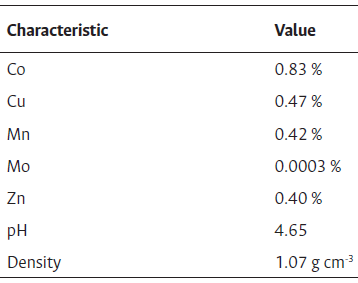
Note: *Information included in the product label.
Aboveground biomass was harvested at 15 cm above the soil in 1 m2 every 5 weeks from the center of each experimental plot (May 6, June 10, July 15, August 19, September 23, and October 29, 2019). The samples were weighed and then placed in a forced air oven at 60 °C for 48 hours. Afterwards, the dried samples were weighed again and the moisture percentage and DMY were obtained from fresh and dry weight, calculated using the equations [1] and [2]. Dried samples were obtained individually using a Thomas-Wiley Laboratory Mill Model 4, passed through a 1-mm screen and stored in a plastic bag for ADF, NDF and N analysis. Ground tissue samples were analyzed at the Dairy One Forage Testing Laboratory in Ithaca, New York, for the calculation of CP (calculated with equation [3]), NDF and ADF, as suggested by Van Soest (1994).
Soil samples were collected from the experimental plot at a depth of 15 cm on two sampling dates, March 19, before treatment, and October 26, 2019, using a grid and a soil corer for the basic soil fertility analysis. The soil samples collected from the last harvest were made in 0.25 m2 and 15-cm depth in each treatment in the center of the plots. Collected soil samples were placed in paper bags and air-dried for five days, then, they were grounded and sieved. Soil nutrients and cation exchange capacity (CEC) were measured by Mehlich III extraction (Sparks, 2007) and filtrated for P, K, Ca, Fe, Mn and Zn in an ICP-OES 7300 DV (Optical Emission Spectrometer). Soil pH was measured in a 1:1 soil, water slurry and CaCl2 solution. Soil OC was analyzed using the dichromate-oxidation Walkley and Black as described by Nelson and Sommers (1982). The results of OC were calculated using the equations [4] and [5].
The data was examined using a two-way ANOVA corresponding to the mathematical model /,, with the fixed factors “treatment” (a) and “harvest” (0), and a one-way ANOVA was made for DMY and soil data analysis using “treatment” as a fixed factor. The Tukey’s test and Least significant difference (LSD) method (level of significance p <0.05) were used as post hoc tests in order to reveal significant differences among treatments. Assumptions of homogeneity and normality of variance were tested using the tests Levene’s and Shapiro-Wilks, respectively. The whole statistical analysis was performed using the SAS system® (SAS, 2021).
NUE was suggested by Dobermann (2005) and calculated in this research to provide a general view of plant performance because the objective of these indexes is to provide evidence about the dose of fertilizer and its economically optimum plant nourishment while minimizing losses.
The estimated weight of root biomass assumes the C potential sequestered on soils. Therefore, roots and crown biomass samples were collected in 0.25 m2 (after final harvests). Roots were transported to the laboratory, washed with water to remove soil, air-dried for one day, and weighed. The root samples were stored in paper bags, dried at 60 °C for 72 hours in a forced air oven and then weighed again.
Results
For DMY, there was a significant interaction between the treatments. The DMY decreased between each period of harvest (1st and 4th harvest) (Table 2). Yield mean values of six accumulated harvests (210 days after planting) showed significant differences. The lowest DMY was observed for the control and MC treatments, with 1091 and 1110 kg ha-1, respectively. These results suggest that MC has no effect on increasing DMY of Mombasa. Dry matter yield doubled on average (>1.0 Mg ha-1) with the application of LU. However, adding MC to the LU did not differ from the sole application of LU, confirming that there was no response of Mombasa to the microbial catalyst treatment MC (Table 2). Accumulated DMY (six 35-d harvests) of LU showed an increase in biomass of over 6 Mg ha-1 in contrast with the control treatment (Table 2).
Table 2 Mean of the effects of the interaction of treatments on dry matter yield (kg ha-1) in 6 35-d harvests of Mombasa at the Agricultural Experiment Station IsabeLa, Puerto Rico
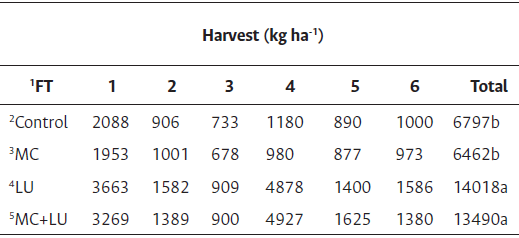
1 Fertilizer treatment (FT), 2Control group, 3Microbial catalyst (MC), 4Liquid urea (LU), 5Microbial catalysts (MC) plus liquid urea (LU). *Means followed by different letters in the same column are significantly different among treatments (p < 0.0001) using a one-way analysis of variance (ANOVA) andTukey's test was used as a post hoc test.
There was no significant interaction between fertilizer treatments at any harvests for CP, but there was a significant main effect for fertilizer treatments (Table 3). Crude protein concentration was similar for the control and MC, averaging 7.4 %. Crude protein percentage was 1.3 % higher in LU treatment compared with MC+LU treatment. This difference is not clearly understood, as a hypothesis was that MC should affect positively N concentrations in plant tissue. The LU applications were approximately 2.3 % higher than the control, indicating a positive effect of LU on N concentrations on tissue.
Table 3 FertiLizer treatment effects and summary of analyses of variance for nitrogen (N) and crude protein (CP) percentages of Mombasa at the Agricultural Experiment Station Isabela, Puerto Rico

1Nitrogen percentage, 2Crude protein percentage, 3Control group, 4Microbial catalyst (MC), 5Liquid urea (LU), 6Microbial catalysts (MC) plus liquid urea (LU),7Fertilizer treatment, 8Harvest, 9Interaction between fertilizer treatments, *harvest. Means followed by different letters in the same row are significantly different among treatments and harvest (p ≤ 0.05) using a two-way analysis of variance (ANOVA) and Tukey’s test was used as a post hoc test.
The apparent recovery efficiency by difference (Er), removal efficiency (RE), CP produced by kg of N applied, partial factor productivity (PFP) and agronomic efficiency (AE) are shown in the supplemental material (Table S3 and S4). The applied doses of DMY and N and the N concentration in tissues were used to calculate these values. This index interpretation was made to provide useful information for growers and livestock farmers to keeping records of inputs, outputs and current status of NUE at a specific place, time and rate. In summary, the PFP index values in this study ranged from 40 to 70 with values > 49. The increase of AE was between 23 and 43 kg in yield per kg of N applied. The Er is better close to 100 %, as shown in the experiments over > 25 to 81 %, and for RE, it is better > 80 %; the values shown in this research were > 69 %. All indexes correspond to well-managed systems. Also, cumulative CP in DMY by kg of N applied was higher in the 4th harvest compared to the 1st one, where the range of CP biomass was 357 to 518 kg CP ha-1. The index values were around 4.38 and 5.3 kg CP produced per kg of N applied (Table S4).
Table S3 Liquid urea and liquid urea plus microbial catalyst effect on N recovery in Mombasa (1st and 4th harvest) and apparent recovery efficiency by difference at the Agricultural Experiment Station Isabela, Puerto Rico
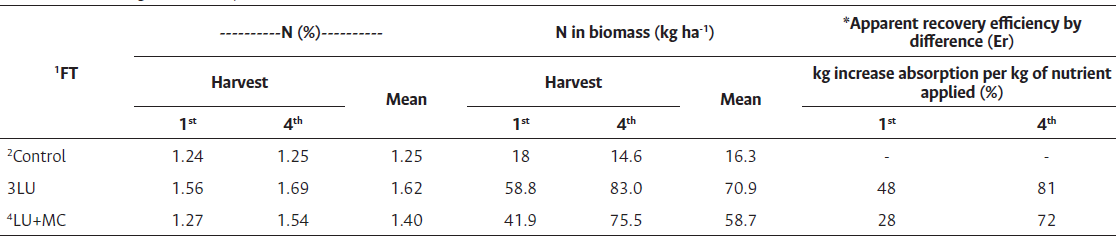
1 Fertilizer treatment (FT), 2Control group, 3Liquid urea (LU), 4Liquid urea (LU) plus microbial catalyst (MC). * Two applications of 84 kg N ha-1 each, and mean per treatment were made, and depending on the index, a control value was used.
Table S4 Removal efficiency (RE) and CP produced by kg of N applied on Mombasa at the Agricultural Experiment Station Isabela, Puerto Rico
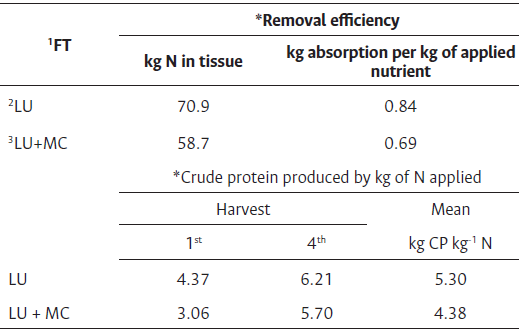
1Fertilizer treatment (FT), 2Liquid urea (LU), 3Liquid urea (LU) plus microbial catalyst (MC). *Mean biomasses of cuts 1st and 4th in kg ha-1.
Table 4 presents the percentage values and analysis of variance for ADF and NDF in tissues of Mombasa on the 1st and 4th harvest. There was no interaction of fertilizer treatment by harvest on ADF, but differences per treatment were found. Mean percentage values for ADF were 39.9 % for MC, while those treated with MC+LU and LU averaged 42.2 % (two percentage points over the MC). An interaction was observed on fertilizer treatment by harvest for NDF; the average for the LU was 74.2 % in contrast to the control (71 %).
Table 4 Fertilizer treatments and summary of analysis of variance for ADF and NDF of Mombasa at the Agricultural Experiment Station Isabela, Puerto Rico

Note:1Acid detergent fiber percentage, 2Neutral detergent fiber percentage (NDF), 3Control group, 4Microbial catalyst (MC), 5Liquid urea (LU), 6Microbial catalysts (MC) plus liquid urea (LU), 7Fertilizer treatment, 8Harvest, 9Interaction between fertilizer treatments. Means followed by different letters in the same row are significantly different among treatments and harvest (p < 0.05) using two-way analysis of variance (ANOVA) and Tukey's test was used as a post hoc test.
There were no significant differences between fertilizer treatments for root biomass (Table 5), which averaged 836 kg DM ha-1 for LU and MC+LU and 560 kg DM ha-1 for the control. The leaf-root ratio (LRR) was 3.7 for the LU treatment and 2.0 for the control. While N had a significant effect on aboveground biomass of Mombasa, it had little or none effect on root biomass.
Table 5 Effects of fertilizer treatments on Mombasa root dry matter yield and leaf root ratio after six 35-d harvests at the Agricultural Experiment Station Isabela, Puerto Rico
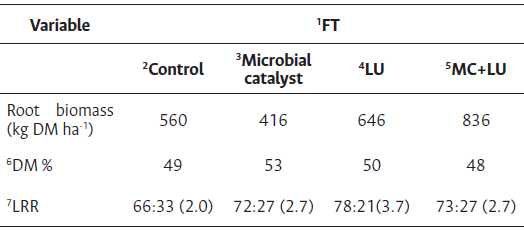
1 Fertilizer treatment (FT), 2Control group, 3Microbial catalyst (MC), 4Liquid urea (LU), 5Microbial catalysts (mC) plus liquid urea (LU), 6dry matter percentage and 7Leaf root ratio. No significant means among treatments were found (p <0.05) based on a one-way analysis of variance (ANOVA) and Tukey's test was used as a post hoc test.
As can be observed in Table 7, the K content was significantly different. The K value was higher in the control group (0.37 meq 100 g-1) than MC and LU with 0.22 and 0.14 meq 100g-1, respectively. As reported in this experiment, N fertilization, specifically N-NH4+, depletes soil K content. Overall, there were no significant effects of treatments on the percentage of OC, OM, P, N, Ca, Mg and CEC. However, there was an increase in N and a decrease in P, as observed in the LU treatment, opposed to the soil conditions before the experiment (Table 6).
Table 6 Soil chemical properties on Mombasa plots (study initiated on March, 2019) at the Agricultural Experiment Station Isabela, Puerto Rico
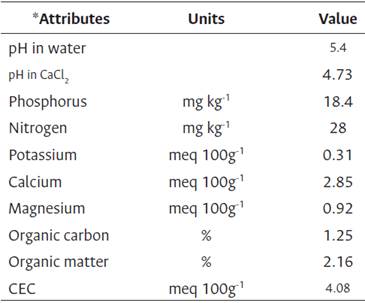
*No calibrated rating
Table 7 Effects of fertiLizer treatments on soiL chemical properties after six 35-d harvests of Mombasa at the AgricuLturaL Experiment Station Isabela, Puerto Rico
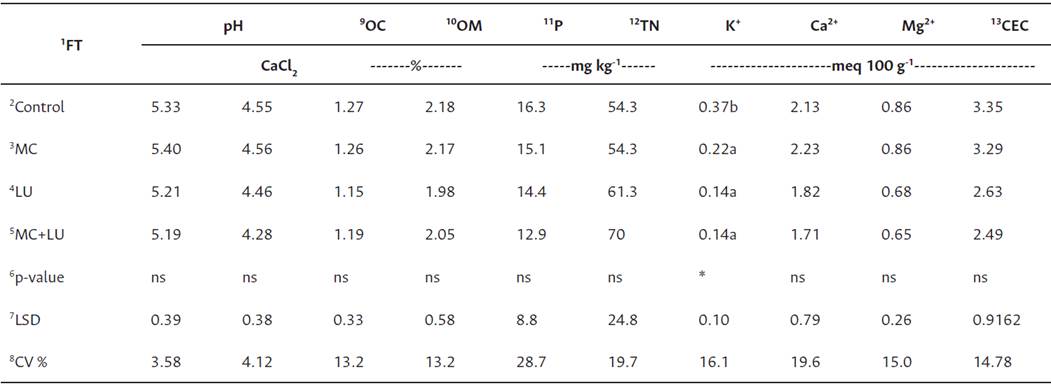
1 Fertilizer treatment (FT), 2Control group, 3Mycrobial catalyst (MC), 4Liquid urea (LU), 5Microbial catalysts (MC) plus liquid urea (LU), 6p-values results significant (p < 0.05) and no significant results (ns) (p > 0.05). Means within a column followed by the same letter are not significantly different among treatments based on a one-way analysis of variance (ANOVA). 7Least Square Difference (LSD) at p < 0.05 was used as a post hoc test. 8C.V. % is the coefficient of variation. 9Organic carbon, 10organic matter, 11phosphorus, 12total nitrogen, 13cation exchange capacity.
Discussion
Several studies have reported the positive effect of N fertilization on forage DMY including Mombasa at 28 to 40-days harvests (Barros et al., 2018; de Oliveira et al., 2020; Escarela et al., 2017; Galindo et al., 2017). The present study showed an increase of 100 %-in the biomass with LU compared to the control after the first 35-d harvest (Table 2). Barros et al. (2018) reported positive effects of the rate of N (100, 200 and 400 kg ha-1) with urea and coated urea. As observed in this study, similar results with 168 kg N ha-1 of LU showed high DMY (Table 2). Regarding the N source, similar performance was found by de Oliveira et al. (2020), when they applied 150 kg N ha-1 as ammonium sulfate. It must be noted that Galindo et al. (2017) studied N-sources such as urea and ammonium nitrate at different doses by cutting it during the dry season, and found no influence of it among sources and doses on biomass production.
The results of cumulative DMY in the present study (Table 2) agree with those reported by Galindo et al. (2017), 2018), where cumulative yields with N fertilization during the dry season reached 10,518 kg DM ha-1 applying 300 kg N ha-1. For example, mean yields were more than 1700 kg DM ha-1, which is adequate for animal consumption (Galindo et al., 2018). Galindo et al. (2017) also observed that guinea grass presented the maximum response with increasing N-rates (range of 300 to 1200 kg N ha-1 yr 1). Caldas et al. (2020) found no significant differences between granular urea and LU with micronutrients on biomass production, however, sole granular urea DMY (3767 kgha-1) was slightly higher than LU (2871 kg ha- 1). Overall, these results were similar to our findings among treatments with LU and LU+MC (Table 2).
An improvement in Mombasa grass CP content using high levels of N input was proven by several studies when harvesting at 24 to 45-d in optimal conditions (Caldas et al., 2020; de Oliveira et al., 2020; Escarela et al., 2017). The mean values of the CP percentage observed in this study at 35-d were 10.2 % for LU and 7.4 % for MC (Table 3). For example, Galindo et al. (2018) reported CP values of 12 to 14 %, more than the control with 10 %, using ammonium nitrate or granular urea, respectively. Caldas et al. (2020) found crude protein content ranged from 12 to 13 % when harvested at 24 to 30-d with similar doses of N. With other grasses such as Marandu grass, Delevatti et al. (2019)forage accumulation rate (FAR reported values close to 16 % CP. It must be noted that high N application rates promote a substantial increase in biomass and can impact the CP level (Escarela et al., 2017). However, our results differed; this study showed < 10 % of CP, corresponding to limited CP requirement by livestock (Abbasi et al., 2018).
In this study, both ADF and NDF values were high due to the mature growth of Mombasa (35-d regrowth) (Table 4). Mean percentage values for ADF were 39.9 % for MC, while those treated with MC+LU and LU averaged 42.2 % (two percentage points over the MC). These values were similar to corresponding values reported by Castagnara et al. (2011) for ADF (42 %) at 42-d harvests. However, lower values of ADF were found by Delevatti et al. (2019)forage accumulation rate (FAR with 27.8 % for 180 kg N ha-1, de Oliveira et al. (2020) reported 35.6 % and Galindo et al. (2018) 34.8 % using 50 kg N ha-1 when Mombasa was harvested at 28-d regrowth. Our NDF values were similar to those reported by Castagnara et al. (2011), that reported 73.5 % of NDF, and de Oliveira et al. (2020) with 70.5 %. However, it is possible to reach lower levels as shown by Galindo et al. (2018) (around 67 % using 50 to 100 kg N ha-1) and Delevatti et al. (2019)forage accumulation rate (FAR (56.4 % using 180 kg N ha-1).
Several authors such as de Oliveira et al. (2020) and Delevatti et al. (2019)of Cenchrus ciliaris and Panicum maximum grown under irrigation at Gode, Somali region. The study was executed using 2 x 3 factorial arrangements in randomized complete block design with three replications. The treatments were three level of fertilizer application (0, 50, 100 kg ha-1 of urea reported that NDF and ADF increased with increasing levels of N. This study contrasts with previous studies, for example, with the low values of NDF at high N doses reported by Caldas et al. (2020) and Galindo et al. (2018). High NDF levels reduce voluntary feed intake and digestibility while high ADF levels reduce forage digestibility. These results could be explained because high N rates promote the growth of new structures and forage that induces lignin synthesis to facilitate a better mechanical self- assembly of tissues and the dilution effect in the cell wall, possibly responsible for the decrease of NDF (de Oliveira et al., 2020). However, this mechanism was not observed in this study. It could be argued that the present results are due to the high capacity of accumulating structural carbohydrates (e. g., Galindo et al., 2018).
Warm-season grass yield has a negative response to N fertilization, indicating that, for these species, any level of N input resulted in the allocation of proportionally less biomass to the roots in favor of the allocation to the shoots. However, in the present study, there was evidence of a large difference when high N was put in with MC, versus the control (Table 5). While N had a significant effect on aboveground biomass of Mombasa, it had little or no effect on root biomass. In this study, there was 100 % more root biomass in fertilizer plots using a high N rate than on unfertilized plots. This experiment considered a possible trend that root mass was higher in the LU treatment, but insufficient evidence was found. Root biomass and rhizodeposition decreased after applying an available N source because plants use C reserves for new regrowth (Zang et al., 2016)especially in the rhizosphere (C excess and N limitation. These processes restrict organic matter stabilizing, for example, urea fertilization depletes soil K content (Table 7). According to Reimer et al. (2020), a higher N input represents mining nutrients from the soil, specially P, K, and Mg. Maltas et al. (2018)carbon (C reported a non-significant but positive effect of N fertilization on OC. The effect observed (Table 7) agrees with previous studies that reported that urea fertilization decreased OC decomposition (Li et al., 2017)but this effect is still very uncertain and depends on living plants. We investigated the effects of mineral N (Nmin, and that the percentage of OC remained constant.
Our results underscore the significance of improving N fertilization, NUE and harvest frequencies and its effects on biomass production and chemical soil properties. Moreover, these results offer a comprehensive view of management practices to take advantage of LU and most productive grass varieties like Mombasa. Further research could be conducted to find an economic way to supply N and promote the adoption of better nutrient management practices.
Conclusion
LU provided an excellent source of N, especially in the NH4+ form required by Mombasa. This research showed the LU application rate and MC as an alternative to enhance Mombasa production in Puerto Rico through synchronized 35-d harvests. The CP concentration with LU suggests that split applications of LU might be profitable in the intensive management of this grass under cutting. Finally, detergent fiber, NDF and ADF were high for 35-d harvests; shorter harvest frequencies could be required to improve the fiber quality of Mombasa.