Introduction
Brazil is leader in coffee production and export, involving more than half a billion people in the production chain of this bean (Tavares et al., 2018). In 2019, coffee cultivation produced almost 50 million bags, occupying 2.21 million hectares (Souza et al., 2020). The state of Espírito Santo is the second largest national coffee producer and the one that produces the most conilon coffee type (Coffea canephora P.) in the country, despite being considered a state of small territorial extension (Conab, 2018).
In Brazil, a significant part of the conilon coffee plantations is located in the coastal tablelands region in the northern part of the State of Espírito Santo and the southern part of the State of Bahia. In this region, soils with low natural fertility, low water retention capacity, and with the presence of a cohesive horizon are common, such as Oxisols and Ultisols (Santos et al., 2018). These characteristics may limit the agricultural production (Cintra et al., 2008), and increase soil erosion and degradation process (Gomes et al., 2012; Martins et al., 2010). In addition, the occurrence of high temperatures in the summer, with the uneven distribution of rainfall, can accelerate the organic matter oxidation, resulting in a negative influence on the crops longevity.
The use of conventional systems based on monoculture has led to soil quality depletion and natural resources degradation (Souza et al., 2017; Gomes et al., 2012). Coffee plantations, when improperly managed, can lead to negative consequences to the soil, such as compaction, loss of nutrients, and accelerated mineralization of organic matter, reducing the potential of agricultural production (Cerri et al., 2017; Guimarães et al., 2014; Souza et al., 2014).
The implementation of coffee plants intercropped with trees or in agroforestry systems is indicated as a viable option to minimize the soil degradation process, since it promotes improvements in the soil quality through a greater contribution of organic residues, greater root development and nutrient cycling (Méndez et al., 2009; Notaro et al., 2014). Coffee plants intercropped with trees and fruit species also promote greater soil coverage, minimizing the erosion process (Haggar et al., 2011). In regions with tropical climate, intercropping or agroforestry systems are favored by the attenuation of extreme climatic conditions, which can reduce soil temperature and increase its water content (Oliosi et al., 2016; Padovan et al., 2015).
The objective of the study was to evaluate the physical-hydrological and chemical properties and organic carbon stock of the soil in conilon coffee crops intercropped with trees and fruit species and in full sun in the coastal tablelands in the north of the State of Espírito Santo.
Materials and methods
The work was carried out in three areas with commercial plantations of conilon coffee (Coffea canephora Pierre ex A. Froehner) located in the municipality of Sooretama, Espírito Santo, Brazil. The region climate is type Aw according to Köppen- Geiger’s classification, with annual rainfall of 1200 mm and a mean annual temperature of 23.4 ºC.
In area 1 (latitude 19°8’51’’ S, longitude 40º8’55’’ W and altitude of 77 m), the treatments compared were conilon coffee intercropped with rubber trees (Hevea brasiliensis (Willd. ex Adr. de Juss.) Muell-Arg.) (CR) and in full sun (FS). In area 2 (latitude 19º8’43’’S, longitude 40º8’51’’ W and altitude of 77 m), the treatments compared were conilon coffee intercropped with papaya (Carica papaya L.) (CP) and in full sun (FS). In area 3 (latitude 19º8’54’’ S, longitude 40º9’57’’ W and altitude of 81 m), the treatments compared were conilon coffee intercropped with coconut (Cocos nucifera L.) (CC) and in full sun (FS). In area 3, native vegetation (NV) (Atlantic Forest Biome) in natural regeneration for 30 years was used as reference. The soil of the three areas is the dystrocohesive yellow Ultisol with flat relief (Santos et al., 2018), with sand, silt, and clay contents of 662, 43 and 295 g kg-1 in area 1; 693, 36 and 271 g kg-1 in area 2, and 762, 16 and 222 g kg-1 in area 3, respectively.
The main characteristics of the plantations were described in Table 1. During the implementation of the six crops, the soil preparation was used only in the planting line of the coffee and tree or fruit species, using the subsoiler at a depth of 0.40 m. Planting was done manually after the correction of soil fertility with the application of limestone, raising the base saturation to 70 %, and adding simple superphosphate in the planting groove, and nitrogen, potassium, and fertilization micronutrients on the cover, as recommended for the region (Prezotti et al., 2007). Irrigation management was based on evapotranspiration of the most demanding crop. The coffee plants were managed with the programmed pruning cycle (Verdin Filho et al., 2014). The spontaneous vegetation between the coffee plant lines was controlled with glyphosate herbicide.
Soil samples were collected from the treatments under study in March 2017 from plots of 10 x 10 m, at a distance of 30 m. The samples were collected in volumetric cylinders of 0.05 x 0.05 m in the planting line at the center of soil layers of 0.00-0.10, 0.10-0.20, and 0.20-0.40 m to determine soil attributes. The physical properties evaluated were soil bulk density, total porosity, microporosity, and macroporosity (Teixeira et al., 2017). Soil water retention was obtained in Richards’ pressure chambers on porous plates, adapted from Teixeira et al. (2017). The available water capacity of the plants was obtained by the difference between the soil water content in the field capacity (yFC = 10 kPa) and the permanente wilting point (YPWP = 1500 kPa).
The soil penetration resistance was measured with an impact penetrometer (Stolf et al., 2005), in the same places where the volumetric cylinders were sampled. The soil water content (qv) and soil temperature were measured along with the soil sampling in the morning from 7:00 to 9:00 a.m. using a portable digital sensor (Decagon Devices, model GS3, Pullman, WA, USA).
The deformed soil samples were collected to determine the chemical properties (Teixeira et al., 2017), being: pH in water (1:2.5 ratio); phosphorus (P), potassium (K), iron (Fe), zinc (Zn), manganese (Mn) and copper (Cu) (Mehlich-1 extractor); calcium (Ca), magnesium (Mg) and aluminum (Al) (KCL - 1mol L-1 extractor); potential acidity (H+Al) (correlation with pH SMP); and boron (B) (hot water extractor).
The sum of bases (SB), cation exchange capacity at pH 7 (CEC) and the base saturation index (BS) were calculated. Soil organic carbon content (OC) was measured with potassium dichromate in presence of sulfuric acid and heating, followed by titration with ammoniacal ferrous sulfate. Soil organic carbon stock (CS) was obtained by the equation CS = OC*BD*L, where BD and L are the soil bulk density and soil layer, respectively.
The O’Brien and Shapiro-Wilk tests were applied to evaluate the variances of homogeneity (p < 0.05) and normality of residuals (p < 0.05), respectively. The statistical analysis was performed considering a completely randomized design, with four replications, through analysis of variance (F-test, p > 0.05) and comparison of means (Tukey test, p > 0.05), according to Notaro et al. (2014) and Souza et al. (2017).
Results
In area 1, CR presented a soil bulk density (1.43 kg dm- 3) lower than FS (1.55 kg dm-3) on average in soil layers, while in areas 2 and 3 the treatments did not differ statistically in the soil layers (Fig. 1). In area 1, CR presented the highest total porosity (0.44 m3 m-3) in the 0-0.1 m layer, in contrast with FS (0.36 m3 m-3), not differing in the other soil layers. In area 2, the treatments did not differ from each other in the soil layers under study. In area 3, CC and NV presented, on average, the highest total porosity (0.40 and 0.40 m3 m-3), while FS presented the lowest (0.36 m3 m-3).
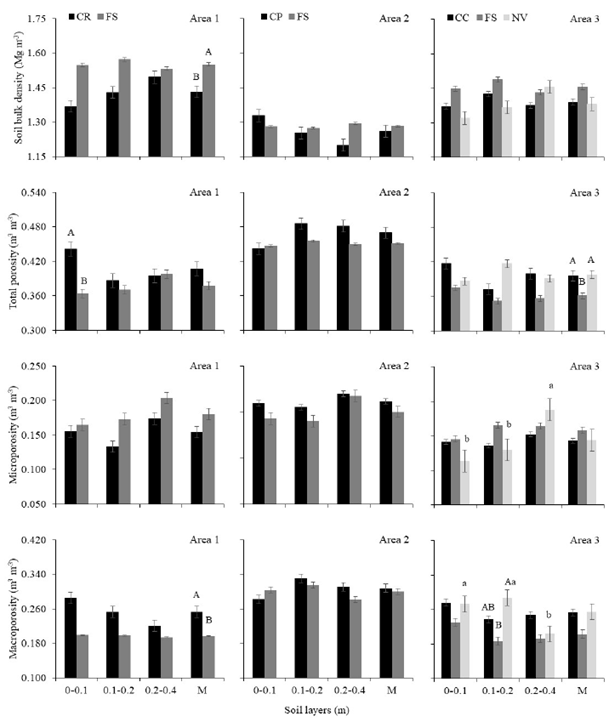
Figure 1 Soil physical properties under conilon coffee intercropped with rubber tree (CR) and in full sun (FS) in area 1; intercropped with papaya (CP) and in full sun (FS) in area 2; and intercropped with coconut (CC), in full sun (FS), and in the native vegetation (NV) in area 3. M = mean of the soil layers. Uppercase letters compare managements and lowercase compare soil layers (Tukey’s test, p > 0.05).
In area 1, CR presented, on average, a greater soil macroporosity (0.25 m3 m-3) in relation to FS (0.20 m3 m-3) (Fig. 1). In area 2, the treatments did not differ statistically. In area 3, the highest soil macroporosity occurred in NV, in the 0.1-0.2 m layer (0.29 m3 m-3), not differing in CC (0.24 m3 m-3). In the other layers, the treatments did not differ. Also, in area 3, a reduction in soil macroporosity occurred in NV in depth (0.08 m3 m-3).
In areas 1, 2 and 3, intercropped coffee and FS did not differ for soil microporosity (Fig. 1). In NV in area 3, there was an increase in soil microporosity in depth (0.07 m3 m-3), which did not occur in the other treatments.
In areas 1, 2 and 3, the conilon coffee treatment did not differ statistically for soil penetration resistance up to 0.4 m depth (Fig. 2). However, in area 3, NV presented the highest values in the layer 0.18 to 0.28 m, ranging from 6.39 to 9.52 MPa.
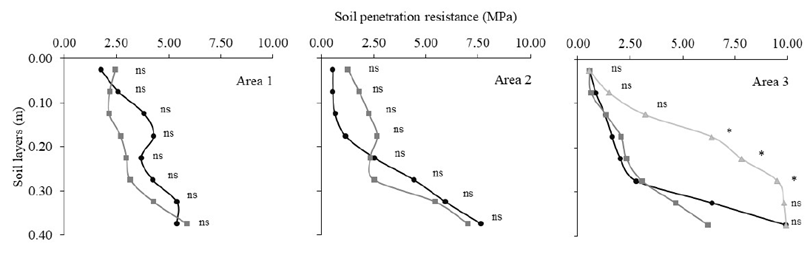
Figure 2 Soil penetration resistance under conilon coffee intercropped with rubber tree ( ) and in full sun ( ) in area 1; intercropped with papaya ( ) and in full sun ( ) in area 2; and intercropped with coconut ( ), in full sun ( ) , and in the native vegetation ( ) in area 3. * = ignificant (Tukey’s test, p > 0.05), ns = not significant.
In area 1, treatments and soil layers presented similar values of available water capacity (AWC). However, in areas 2 and 3, CP and CC presented higher AWC (0.043 and 0.044 m3 m-3) than FS treatments (0.032 and 0.038 m3 m-3). In area 3, NV presented even lower AWC (0.034 m3 m-3) than CC and FS.
In area 1, the treatments did not differ statistically for water content in the soil layers (Fig. 3), however, FS presented higher soil water content in depth (0.11 m3 m-3), which did not occur in CR. In area 2, CP presented, on average, higher soil water content (0.27 m3 m-3) than FS (0.25 m3 m-3) in soil layers. In area 3, coffee treatments did not differ statistically from each other (0.21-0.22 m3 m-3), but presented higher values than NV (0.09 m3 m-3).
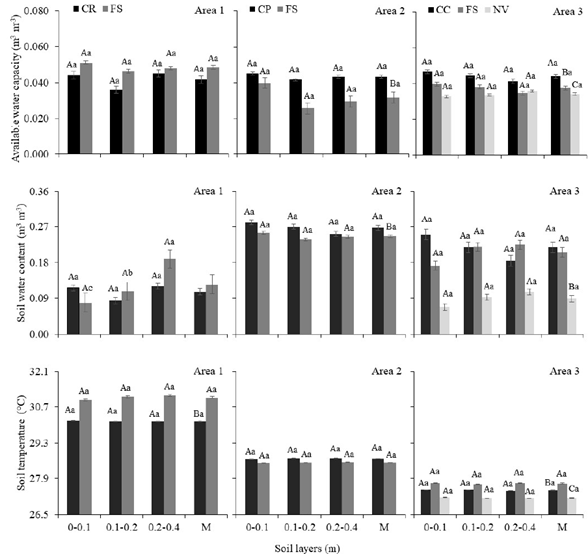
Figure 3 Available water capacity, soil water content and soil temperature under conilon coffee intercropped with rubber tree (CR) and in full sun (FS) in area 1; intercropped with papaya (CP) and in full sun (FS) in area 2; and intercropped with rubber tree (CC), in full sun (FS), and with native vegetation (NV) in area 3. M = mean of the soil layers. Uppercase letters compare managements and lower case compare soil layers (Tukey’s test, p > 0.05).
In areas 1 and 3, FS treatments showed higher soil temperature (31.1 and 27.7 ºC) in relation to the intercropped treatments (30.1 and 27.4 ºC) (Fig. 3). In area 3, NV presented the lowest soil temperature (27.1 ºC). In area 2, the temperature of the treatments did not differ significantly in the soil layers, ranging from 28.5 to 28.7 ºC.
Regarding the analysis of soil chemical properties, in area 1, treatments did not differ statistically from any of the chemical properties analyzed in the soil layers (Fig. 4). In area 2, CP showed higher values of P and Mn in the 0-0.1 m depth layer, higher pH, P, Ca, SB, BS and Mn values and lower Al and H+Al values in the layer of 0.1-0.2 m, and higher pH, P, SB and BS and lower Al and H+Al values than FS in the layer of 0.2-0.4 m. In area 3, CC and NV presented the highest Mn contents in the 0-0.1 m layer (Fig. 4), while FS presented the highest value of P. In the layer of 0.1-0.2 m, FS presented the highest levels of P and Zn, while NV presented the highest value of Mn. In the 0.2-0.4 m layer, CC and NV presented the highest values of P and Mn, respectively.
In areas 1 and 2, conilon coffee intercropped and in full sun did not present statistical differences for the soil organic carbon stock, ranging from 57 to 63 and from 42 to 50 Mg ha-1, respectively (Fig. 5). In area 3, NV presented higher soil organic carbon stock (98 Mg ha-1) in relation to coffee managements (70 and 49 Mg ha-1). CC presented lower reduction in soil organic carbon stock (29 %) than FS (50 %).
Discussion
CR and CC showed evidence of the improvement in the physical-hydrological quality of soil in relation to FS in areas 1 and 3. The root development of rubber and coconut trees contributed to the formation of biopores, which reduced soil bulk density and increased total porosity, especially macropores, which agrees with Souza et al. (2017). Moreover, a greater root development of tree and fruit species also allows them to extract water and nutrients in deeper soil profiles, contributing to a better use of these resources (Cintra et al., 2008; Padovan et al., 2015).
For CP, the greater machine traffic between the planting lines influenced the arrangement of the soil particles, with values of soil bulk density and total porosity similar to FS. This higher machine traffic in CP was necessary because of the greater use of pesticides and herbicides in papaya cultivation than in coffee in FS. The papaya tree presents even less rooting capacity than the other intercropping managements, concentrated on the soil surface and close to the micro-sprinklers (Souza et al., 2016). A lower rooting requires greater control in irrigation management and a localized application of fertilizers.
The soil of the areas under study is dystrocohesive yellow Ultisol. In natural conditions such as native vegetation, it is an acidic soil and present natural compaction in the B horizon (Santos et al., 2018) (Fig. 2), which hinders the root development in agricultural crops (Cintra et al., 2008; Oliosi et al., 2016; Souza et al., 2016). However, in area 3, soil preparation with subsoiler to 0.40 m in the implementation of CC and FS contributed to reduce the soil penetration resistance to 50 % in the 0.2-0.4 m layer and increased the available water capacity in relation to NV. Similar results were observed by Souza et al. (2014 and 2017). This lower soil penetration resistance favors the root development and, consequently, the productive capacity of the agricultural crops (Cintra et al., 2008; Padovan et al., 2015; Souza et al., 2016).
In area 2, the higher soil water content under CP was the result of a lower water demand in relation to FS. The decomposition of the papaya cultural residues resulted in the occurrence of biopores on the surface, which contributed to a greater infiltration and retention of water in soil (Fig. 3). In area 3, the lower soil temperature under CC in relation to FS was a result of greater soil shading (Fig. 3), agreeing with Padovan et al. (2015 and 2018) and Souza et al. (2017). In NV, the higher water demand of the tree species and the absence of irrigation resulted in lower values of soil water content, agreeing with Souza et al. (2017); however, the shading resulted in lower soil temperature.
In areas 1 and 3, CR and CC presented soil water content similar to FS. Nevertheless, Padovan et al. (2015 and 2018) observed a reduction in soil water content under coffee plants in agroforestry systems compared to full sun. Even the improvements in the microclimate of the intercropped coffee (Oliosi et al., 2016), which reduced the soil temperature (Fig. 3), were not sufficient to increase the soil water content, probably due to the higher demand of the crops, agreeing with Souza et al. (2017). In area 1, rubber trees were not yet in the process of tapping, that is, when the latex removal begins, water demand will increase (Mesquita et al., 2006), which will increase the competition with coffee plants, requiring adjustments in the irrigation management. In area 1, FS presented an increase in soil water content in depth, which was related to the lower soil water retention in the surface layer (Fig. 3), resulting in its vertical movement to the subsurface, which may be unavailable to the coffee plant. In intercropped managements, this soil water available in deeper layers could be used by trees species, agreeing with Padovan et al. (2015).
Improvements in soil fertility were observed in area 2 in CP relative to FS. This improvement was related to the increase of pH, P, Ca, BS, and Mn values, the reduction of Al and H+Al contents, and the conservation of high micronutrient levels, according to Haggar et al. (2011) and Notaro et al. (2014). This improvement was caused by the greater fertilizer application in papaya, and the residue used by the coffee plant after the senescence of the papaya plants, which can be advantageous in the productive and economic aspects.
In area 3, the addition of nutrients to the soil in CC and FS resulted in similar contents for most of the soil chemical properties. These contents in NV were similar to those of coffee treatments because of the nutrient cycling that allows the maintenance of soil fertility (Luizão et al., 2006). However, NV presented the lowest values of P due to the low natural levels of this element in Brazilian soils (Broggi et al., 2010). The higher levels of P and Zn in FS are related to lower nutrient demand in relation to CC and to fertilization of coffee plants compared to NV (Fig. 4).
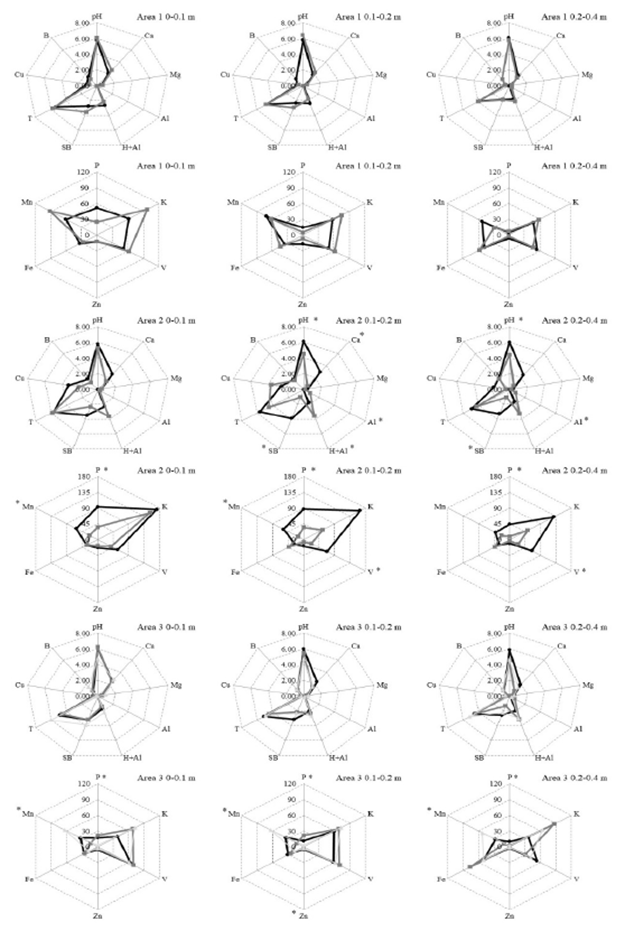
Figure 4 Soil chemical properties under conilon coffee intercropped with rubber tree ( ) and in full sun ( ) in area 1; intercropped with papaya ( ) and in full sun ( ) in area 2; intercropped with coconut ( ) , in full sun ( ) , and in the native vegetation ( ) in area 3. * = significant (Tukey’s test, p > 0.05).
In general, FS areas presented greater chemical limitations in soil for agricultural production (Fig. 4), based on the limits defined by Prezotti et al. (2007). P, K and Cu were the most limiting properties in areas 1 and 3 in the treatments under study, while in area 2 it was Mg in the two treatments and P and K in FS. Such low availability of essential nutrients indicates the need for adjustments of organomineral fertilization, which is essential for the economic viability of agricultural activity.
The anthropic process of agricultural cultivation resulted in the reduction of soil carbon stock in relation to NV in area 3 (Fig. 5). The conversion of native vegetation to agricultural systems results in a decline of the litter and, consequently, the organic carbon in soil (Cerri et al., 2017; Notaro et al., 2014). However, CC minimized soil carbon losses in relation to FS, in agreement with Guimarães et al. (2014) and Souza et al. (2012). The higher soil carbon stock under NV was a result of the greater availability of plant residues and a bigger soil cover, which contributes to the greater stability of organic matter in the soil and its physical protection (Notaro et al., 2014).
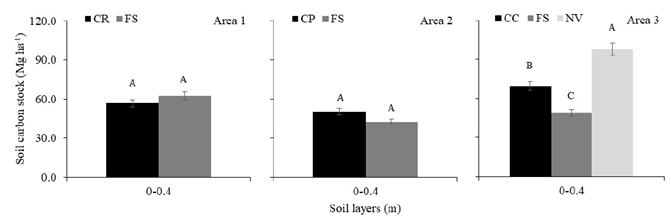
Figure 5 Soil carbon stock under conilon coffee intercropped with rubber tree (CR) and in full sun (FS) in area 1; intercropped with papaya (CP) and in full sun (FS) in area 2; and intercropped with coconut (CC), in full sun (FS), and in the native vegetation (NV) in area 3 in a 0-0.4 m soil layer. Upper case letters compare treatments (Tukey’s test, p > 0.05).
In areas 1 and 2, the treatments with intercropped conilon coffee (CR and CP) did not differ from the treatments in FS. The residues produced by rubber and papaya trees present high nutritional quality with lower lignin contents, which favors its decomposition (Luizão et al., 2006). This reduces the accumulation of organic residues on the soil surface in CR and CP in relation to CC. In addition, FS in areas 1 and 2 showed longer implementation time, which is associated with the annual pruning management (Verdin Filho et al., 2014), as weed breeding preserves soil carbon stocks, agreeing with Cerri et al. (2017).
Conclusion
Conilon coffee plantations intercropped with rubber trees improved the soil physical quality, while the coffee intercropped with coconut trees improved the soil physical-hydrological quality and increased the soil carbon stock in relation to coffee cultivation in full sun in the coastal tablelands region, contributing to soil and water conservation and to the sustainability of the agricultural activity.
Conilon coffee intercropped with papaya improved the soil fertility and available water capacity in relation to the management in full sun, favoring the maintenance of the productive potential of the soil in these agroecosystems.