Introduction
Carrot is an olericulture species belonging to the Apiaceae family and a widely appreciated food at global level. The production success of vegetables such as carrots depends on the establishment of their seedlings in the field that directly affects germination capacity and seed vigor. One of the factors that affect seed germination is temperature. Pereira et al. (2007) reported a decreased germination capacity of 16 carrot seed cultivars at 35 °C, which are normally cultivated in the summer. The germination capacity was reduced 70 % to 80 % at 20 °C and <10 % at 35 °C. An uneven emergence of carrot plants can lead to developmental delays, problems in weed control, and crop unevenness at different phenological stages, including maturation.
Another factor that can compromise seed germination is the presence of excess salts in the soil. According to Lima and Torres (2009), the tolerance of plants to excess salts can be determined by evaluating the germination percentage of seeds after exposing them to salinity that negatively affects plant growth and development. Dias et al. (2015) reported that salt stress negatively affects the germination of carrot seeds.
Biostimulants that are applied to seeds are generally chemicals; however, new natural and eco- friendly alternatives are needed for the sustainability of production systems. Agricultural biostimulants are a potential alternative to synthetic chemicals as they make the environment sustainable and resilient (Van Oosten et al., 2017). Among various natural biostimulants, seaweed extracts are potential and reliable candidates.
According to Khan et al. (2009), seaweed extracts can increase the crop performance because the bioactive compounds present in these extracts improve plant tolerance against abiotic stresses (Bulgari et al., 2019) such as water deficit, soil salinization, and exposure to sub-optimal growth temperatures (Du Jardin, 2015).
Jayaraj et al. (2008) reported that algae extracts minimize leaf diseases caused by Alternaria radicina in carrots. According to Peñuela et al. (2018), red algae are the source of polysaccharides in the carrageenan group, exhibiting an eliciting action and provoking defense responses in plants, possibly because of their high sulfate content (Mercier et al., 2001). However, few studies have been performed pertaining to the effects of algae-based biostimulants, especially red algae of the genus Solieria, on the physiology of seeds. This study aimed to determine the effect of a treatment with an algae-based biostimulant made from Solieria filiformis on the physiology and stress tolerance of carrot seeds.
Materials and methods
The study was performed at the Seed and Plant Pathology Laboratories at the Universidade Federal da Fronteira Sul, campus Chapecó, Brazil. Carrot seed cultivars (cv.) Nantes and Brasília Irecê were used. The extract used as biostimulant was a commercial product from the red alga S. filiformis containing 7.5 % of Mn, 13 % of S, and bioactive compounds, and with a density of 1.3 g/cm3. Bioactive compounds were identified by high-performance liquid chromatography (HPLC) using an HPLC equipment (HP model 1100), Lichrospher RP18 column (5 µm) equipped with a 210-nm UV detector, and a quaternary pump system. The reverse-phase analysis was performed using solvent A (Milli-Q water with 1 % phosphoric acid) and solvent B (acetonitrile). The gradient pumping system of the mobile phase used was 90 % of solvent A from 0 to 5 min; 60 % of solvent A from 5 to 40 min; and 90 % of solvent A from 45 to 50 min. The standard flow was maintained at 0.5 mL min−1, according to Bravo et al. (2006).
The samples were filtered through 0.45 µm- nylon membranes. The bioactive compounds were identified according to their order of elution and by comparing their retention time with that of their pure standards. They were then quantified using the external standardization method by comparing the area (mAU*s) of the compound peak with the standard curve plotted using each standard (gallic acid, epigallocatechin, catechin, epicatechin, epigallocatechin gallate, rutin, ferulic acid, naringin, hesperidin, myricetin, resveratrol, quercetin, apigenin, and canferol). The result was expressed in terms of µg/mL of extract.
In all tests, the experimental design used was completely randomized, and the experiments were performed separately for each cultivar.
Seed treatment
Biostimulant doses were set according to Jayarat et al. (2008), at the following concentrations: 0, 1, 2, and 4 mL L−1. After treatment, seeds were dried at natural room temperature for 24 h. Seed germination and seedling growth were evaluated at different temperatures (a) and at different salt concentrations (b), as described below.
a) Seed germination and seedling growth at different temperatures
Germination test
Four repetitions of 50 seeds were distributed on a germitest paper moistened with water (2.5 times the weight of the paper) and kept in a gerbox type plastic box (germination chambers) at 20 °C (ideal) and 30 °C (stress). Seed germination and seedling growth were evaluated at 7 and 14 days after sowing according to the criteria established in the Brazilian rules for seed analysis (Brasil, 2009). The results were expressed as percentages (%).
Germination speed index
The germination count was made daily to obtain appropriate results. Seeds with primary root protrusion were considered, according to Nakagawa (1999).
Seedling root and shoot length
The seedling root and shoot length was determined according to Nakagawa (1999) at the end of the germination test (14 days after germination). 20 normal seedlings per repetition were used; the measurements were performed using a graduated ruler and expressed in centimetres (cm).
Seedling dry mass
After determining the length, seedlings were separated into roots and shoots, placed in paper bags, dried in an oven with forced air circulation at 65 °C for 72 h (Nakagawa, 1999), and the dry mass was determined by weighing them on a precision balance. The value per seedling was obtained by dividing the mass by 20 (number of seedlings used), and then, it was converted into milligrams.
b) Seed germination and seedling growth at different salt concentrations
The seed germination test in the presence of a saline solution was performed according to the method used by Bolton and Simon (2019). Seeds were distributed on a germitest paper moistened with a saline solution (150 mMol of NaCl) or distilled water (control), and placed in a gerbox. Germination, germination speed index, and seedling growth were evaluated as described in section a.
Statistical analysis
An analysis of variance (ANOVA) was used to perform the statistical analysis, in a factorial scheme 2 x 4 (temperature x doses or salinity x doses). Data normality was previously tested by the Shapiro-Wilk test. The results were analyzed by ANOVA and compared by Tukey’s test (for cultivar, temperature or salinity) and regression (for doses); as no significant differences were observed for doses in the regression analysis, these results were compared by Tukey’s test (p<0,05) using the SISVAR® statistical program (Ferreira, 2019).
Results and discussion
Extract characterization
dentification of the molecular characteristics of the extract can be seen in Table 2. The HPLC analysis showed the presence of 63.79 µg L−1 of gallic acid in the extract (Table 1). Gallic acid has been reported in extracts obtained from other species of red algae such as S. robusta (Petchidurai et al., 2019), Gracilaria birdiae and G. cornea (Souza et al., 2011), and Amphiroa anceps, G. cordicata, and Kappaphycus alvarezii (Tanna et al., 2019). Gallic acid is a strong antioxidant and a phenolic compound widely distributed in plants (Niemetz and Gross, 2005; Locatelli et al., 2009) and algae (Fernando et al., 2016).
Table 1 Identification of possible compounds and quantification of phenolic compounds present in the red algae-based biostimulant (Solieria filiformis)

Table 2 Identification by direct infusion present in the red algae-based biostimulant (Solieria filiformis)
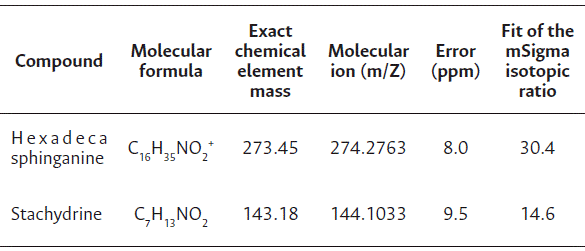
Germination and seedling growth at different temperatures
For the germination and germination speed index of cv. Brasília Irecê, a difference was observed between temperatures and among the extract doses only at 30 °C. A reduction in germination was observed for all doses at this temperature, reaching values of 0 % and 2 % at 1 and 2 mL L−1 of biostimulant, respectively (Table 3).
A drastic reduction in the root length of cv. Brasília Irecê was observed at 1 and 2 mL L−1 of biostimulant at 30 °C, and 4 mL L−1 of biostimulant, compared to control. However, the differences were small at 20 °C, and the highest root length was observed at a dose of 4 mL L−1. No significance was observed between the dose and root length in the regression analysis; nevertheless, an increase of more than 100 % in root length was observed in Tukey’s test at 20 °C and 4 mL L−1 of biostimulant, showing a positive response to the seed treatment (Table 3).
The stimulating effect of algae-based biostimulants on root growth has been reported in tomato (Oancea et al., 2013), corn (Ertani et al., 2018), cauliflower (Rengassamy et al., 2016), and carrot (Alam et al., 2014). Gallic acid present in the extract (Table 1) might have induced seedling growth by participating in the metabolic pathways. Zeid et al. (2019) reported that gallic acid promotes the germination and development of Vigna unguiculata seedlings by increasing the activity of hydrolytic enzymes that mobilize seed reserves.
Shoot length at 30 °C was reduced from 5 cm (control) to 0 and 0.3 cm at 1 and 2 mL L−1 of biostimulant, respectively, indicating no positive effect of the biostimulant used. However, at 20 °C, all doses stimulated more growth than that of the control treatment. As dry mass accumulates in carrot shoot seedlings, cv. Brasília Irecê showed high growth at 4 mL L−1 at 20 °C (Table 3).
Table 3 Treatment of carrot seedlings cv. Brasília Irecê with biostimulant based on red algae (Solieria filiformis) at 20 °C and 30 °C
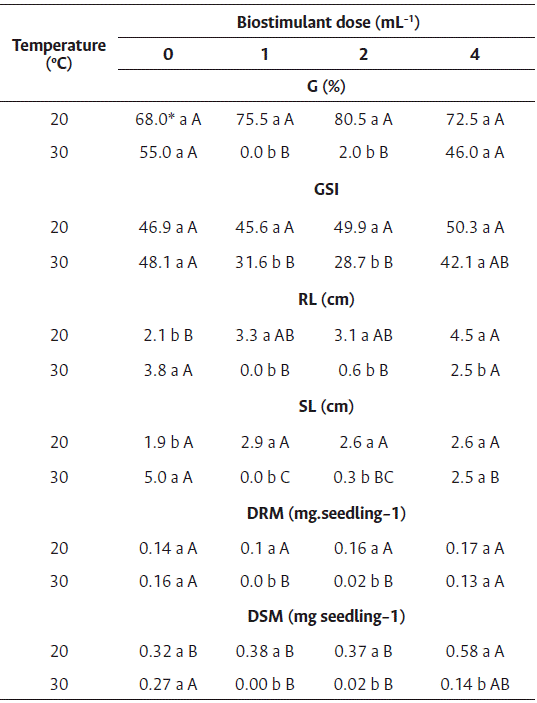
* Averages followed by the same lowercase letter in the column and uppercase in the row did not differ by Tukey’s test (p<0.05).
G: Germination, GSI: germination speed index, RL: root length, SL: shoot length, RDM: root dry mass, SDM: shoot dry mass.
Du Jardin (2015) reported that algal biostimulant improves seed germination and plant growth and development because of its effects on hormones. Cytokinins, auxins, abscisic acid, gibberellins, and other classes of hormone-like compounds such as sterols and polyamines are present in seaweed extracts (Craigie, 2011).
For the germination and germination speed index of cv. Nantes, a difference was observed between temperatures and among the doses of biostimulant only at 30 °C (Table 4). A reduction in germination and in the germination speed index was observed for all doses at this temperature.
High temperatures impair the germination of carrot seeds; Pereira et al. (2007) reported a negative affectation of carrot germination at a temperature of 36 °C. Nonogaki et al. (2010) reported that temperatures higher than optimum affect metabolic events in carrot seeds that occur during phases I and II, preventing them to enter phase III (root protrusion) due to thermoinhibition.
The gene expression studies in Arabidopsis thermoinhibited seeds revealed that high temperatures stimulate the biosynthesis of abscisic acid and repress the biosynthesis of gibberellins (Toh et al., 2008). Seed germination is regulated by a balance between gibberellins and abscisic acid, which act antagonistically (Shu et al., 2016; Heden, 2019).
For the root length of cv. Nantes, differences were observed between temperatures, but not among the doses of biostimulant (Table 4). Similar to these results, Silva et al. (2018) demonstrated that the Ascophyllum nodosum extract has inhibitory effects on the growth of Celosia cristata.
However, for the shoot length of carrot seedlings, differences were observed between temperatures and among the doses of biostimulant at 30 °C. The seeds treated with 1 and 2 mL L−1 of biostimulant at 30 °C showed higher shoot length than those treated with 4 mL L−1, although the difference was statistically insignificant compared to the control treatment (Table 4).
Table 4 Treatment of carrot seedlings cv. Nantes with biostimulant based on red algae (Solieria filiformis) at 20 °C and 30 °C
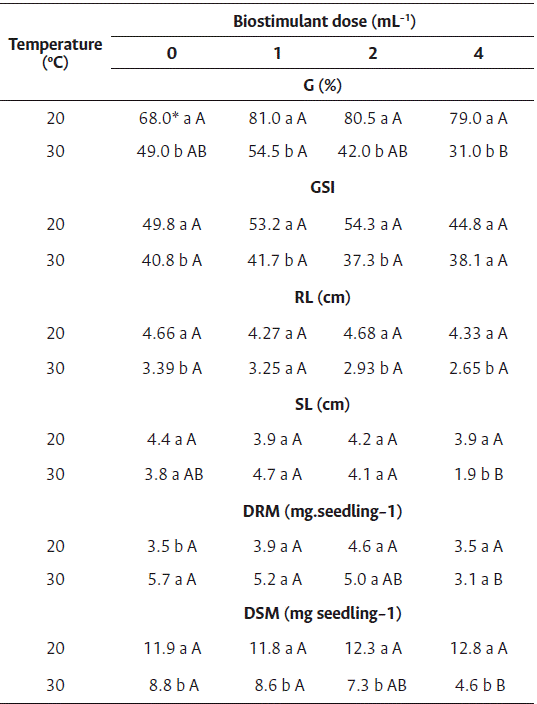
* Averages followed by the same lowercase letter in the column and uppercase in the row did not differ by Tukey’s test (p<0.05).
G: Germination, GSI: germination speed index, RL: root length, SL: shoot length, RDM: root dry mass, SDM: shoot dry mass.
The root and shoot dry mass (Table 4) showed differences between temperatures and among the doses of biostimulant only at 30 °C; however, positive effects of the biostimulant were not observed.
Kauffman et al. (2007) reported that biostimulants can promote plant growth when applied in small amounts; however, their optimum levels for seed treatment are unknown for most cultivated species.
Germination and seedling growth at different salt concentrations
Seeds of cv. Brasília Irecê subjected to saline stress did not germinate, regardless of the dose of biostimulant, indicating that the condition of salinity is harmful for germination. In contrast, in control without salinity, germination was observed in the range of 46 % to 66 %, depending on the dose of biostimulant, and low germination values were observed in the two highest doses of biostimulant (Table 5). For the germination speed index (Table 5), differences between the saline treatments were observed. Seeds subject to saline stress showed lower germination rates, and no differences were observed among the doses of biostimulant.
Table 5 Treatment of carrot seedlings cv. Brasília Irecê with biostimulant based on red algae (Solieria filiformis) subject to salinity stress
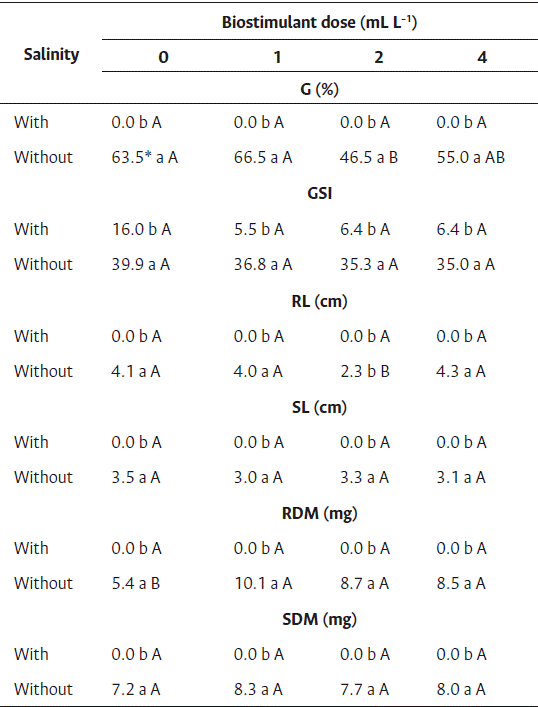
* Averages followed by the same lowercase letter in the column and uppercase in the row did not differ by Tukey’s test (p<0.05).
G: Germination, GSI: germination speed index, RL: root length, SL: shoot length, RDM: root dry mass, SDM: shoot dry mass.
Regarding the root and shoot length and dry mass, seeds subject to saline stress showed all values equal to zero because there was no adequate seedling development (Table 5). Saline stress exerts a negative effect on the chlorophyll content of plants, and thereby plants produce less fresh mass (Shyiab et al., 2019). Nasri et al. (2015) reported less root development in carrot seedlings because of the toxic effects of high sodium chloride concentrations, as well as unbalanced absorption of nutrients by the seedlings. No dose of biostimulant promoted the growth of seedling roots under saline conditions (Table 5). In the control group (without salinity), growth reduction was observed at 2 mL L−1 of biostimulant.
High dry root mass values were observed for seeds treated with biostimulant (Table 3), ranging from 8.55 to 10.10 mg at 1, 2, and 4 mL L−1 of biostimulant, and 5.37 mg for control. In addition to improved tolerance to environmental stresses such as water scarcity in the soil, salinity, and high temperatures, algae-based biostimulants improve root development and mass accumulation (Mukherjee and Patel, 2020).
Similar results as those obtained for the effect of salinity on seed germination of cv. Brasília Irecê were obtained for cv. Nantes (Table 6). The seeds subject to saline stress did not germinate, whereas those in the control group (without salinity) germinated.
Table 6 Seed treatment of carrot seedlings cv. Nantes with biostimulant based on red algae (Solieria filiformis) subject to salinity stress
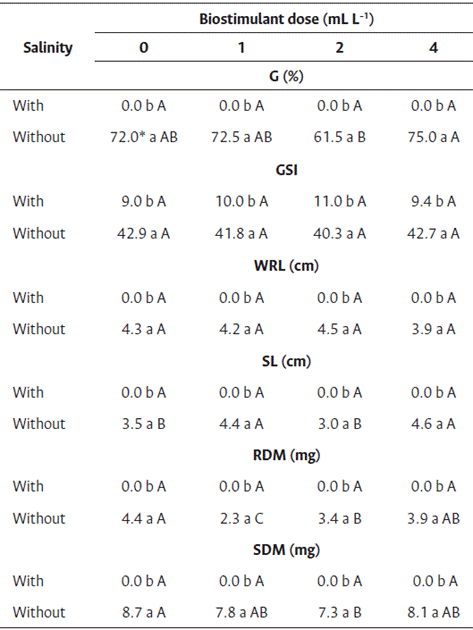
* Averages followed by the same lowercase letter in the column and uppercase in the row did not differ by Tukey’s test (p<0.05).
G: Germination, GSI: germination speed index, RL: root length, SL: shoot length, RDM: root dry mass, SDM: shoot dry mass.
The differences in germination were observed between the doses of biostimulant only for seeds that were not exposed to salinity, although none of the doses increased the germination. The loss of germination caused by the saline environment can be overcome, and the germination capacity can be recovered by providing NaCl-free conditions (Shiyab and Simon, 2017).
Differences were observed between the germination speed indices at different NaCl concentrations (Table 6), and low germination rates were observed in the seeds subject to saline stress, although no differences were observed between the germination speed indices at different doses of biostimulant. Similar to root and shoot length and dry mass, the germination was affected by salt stress.
For root length and shoot dry mass of seeds in the control treatment (without salinity), differences were observed among the different doses of biostimulant. However, the increase in seed root length and shoot dry mass was less compared with those without treatment with biostimulant (Table 4). Thus, the biostimulant did not increase the salinity tolerance in carrot seeds. Further studies with other dose ranges are needed to identify the effect of seaweed biostimulants on salinity tolerance of carrot seeds.