INTRODUCTION
The Eastern Plains of Colombia include 17 million ha ofwell-drained neotropical savannas, 3.5 million of which have low slopes and can be cultivated, while 6.4 million are hilly, dissecting landscapes resting over soils of variable, but generally superficial soils, except in small valleys that are occasionally sown with introduced pastures (Sánchez and Cochrane 1985). Although imperfectly understood (Rippstein et al. 2001; Parr et al. 2014), these extensive rangeland savannas (i.e. Llanos) are a strong element in Colombian culture, historical and socio-economic discourse, whilst having a significant heritage, ecological and geological value for native, colonial, and other multiethnic inhabitants (Navas Ríos 1999; Pulido et al. 2007).
However, despite ongoing intensification of agriculture, cultural, fisheries, forestry, mining, recreational and ecotourism industries (Castiblanco et al. 2015), the beef industry on neotropical savannas and sown pastures have historically captured the main land use (Van Ausdal 2009; Rausch 2013; Huertas-Ramírez and Huertas-Herrera 2015). A similar picture, but at a broader scale is evident within the biophysical, socio-economic, human (indigenous and non-indigenous peoples) and cultural subsystems of the remote beef breeding systems on the fragile northern Australia rangelands [native grasslands, shrublands, woodlands and tropical woodlands savannas (Tothill and Gillies 1992; Marshall and Smajgl 2013; Marshall et al. 2014; Russell-Smith and Sangha 2018)].
In this context, and in order to meet farm and non-farm livelihoods' aspirations and maintain social and communal structures, it is desirable to preserve the identity of extensive breeding beef herds. This requires to deal not only with nutritional and reproductive efficiencies, but to adopt best practices regarding the growth and commercialization of weaners, stockers and yearlings (Kleinhesterkamp and Habich 1985), which in turn drives a wide year-to-year variation in performance and outputs of the pastoral system (Mejía et al. 2004).
In parallel, sustainable production and/or sustainable intensification of the sensitive-resource-dependent beef industry on savannas constitutes a major challenge, given inter-dependencies and inter-relationships amongst but not limited to (i) increasing land transformation (Smith et al. 2010; Australian Government 2015); (ii) vulnerability to climate uncertainty (Marshall et al. 2014; Ramos-Montaño and García-Conde 2016); social resilience and adaptative capacity of primary producers and rural communities to climate
change (Marshall 2010; Marshall and Smajgl 2013); and the required implementation of an integrated extensive mitigation pastoral framework (Ramírez-Restrepo and Charmley 2015) to assist nutritional security and positive farm financial returns in the context of a growing global food-quality demand [(CONPES) 2014; Thompson and Martin 2014; Norton 2017].
In this scenario, the potential animal production of the well-drained savanna environment is well known to pastoralists in Colombia (Thomas et al. 1990; Lascano 1991), however, the environmental impacts of extensive beef system practices have been little studied (Tapasco et al. 2015). In this regard, it is important to note that attempts at quantifying these impacts still remains controversial because constructed lines of evidence have largely relied on surmised parameters from the international literature with limited applicability and/or inputs from local field research (Etter et al. 2011; Lerner et al. 2017).
As suggested above, reaching valuable global markets increasingly require quality products supported by documented, low carbon foot printing (McAulifee et al. 2018). This is consistent with Godde et al. (2019) and Tedeschi et al (2002, 2019), who argued that in the face of climate variability it is critical to use modelling approaches to capture short and long, simple and complex environmental livestock representations of earlier or current real-life farming systems in tropical Australian rangelands or elsewhere. Therefore, baseline data and scenarios for various environmental burdens from savanna-beef based production systems in Colombia are desirable to integrate local deep knowledge-sharing and data-driven modelling. Interestingly, more is known about some ecological impacts of sown pastures (Fisher et al. 1994; Ramírez-Restrepo and Vera 2019) in this extensive neotropical savanna environment, than from grazed native savannas per se (Ramírez-Restrepo et al. 2019).
The objective of this study was to quantify cow-calfbeefmethane (CH4) emissions and derived intensity and efficiency indices over two consecutive reproductive cycles (RCs) in relation to weaning and yearlings' age, using a mathematical flux matrix of individual live weight (LW) data from a five-year experiment at the Carimagua Research Centre (CRC) located in the Llanos of Colombia.
MATERIALS AND METHODS
Site conditions details
Carimagua Research Centre situated in the Meta Department is located on a well-drained sandy loam or clay loam Oxisols with overall bulk density, soil pH, available phosphorus, soil organic matter and aluminum saturation values of 1.4 g/ cm3, 4.74, 2.48 mg/kg, 3.07% and 80%, respectively (Rao 1998; Fisher et al. 1994; Rao et al. 2001). Mean rainfall precipitation and ambient temperature during the December to March period (27.4 oC and 169 mm/m2) and the wet season (26.2 oC and 2,790 mm/m2) over the 1984-1987 period studies were recorded at CRC meteorological site (4o36'44.6" N latitude, 74o08'42.2" West longitude; Vera and Ramírez-Restrepo 2017).
Studies used for modelling
Liveweight data comes from projects designed by R. R. Vera Infanzón and implemented by the two authors in the context of the International Center for Tropical Agriculture (CIAT)'s former Tropical Pastures Program, and financed by its core budget. It covers two full and consecutive RCs and replicated twice in consecutive years sourced from neotropical savanna grazing trials conducted ethically by Colombian registered Doctors ofVeterinary Medicine (Vera and Ramírez-Restrepo 2017). The experiments involved Brahman (Bos indicus) and crossbred Brahman x San Martinero (native; Bos taurus) cow-calf pairs subject to conventional weaning (CW; 304 ± 2.29 days) and early weaning (EW 114 ± 2.20 days) herd management practices.
The original research also served a practical purpose of identifying in EW herds, the feeding value of Andropogon gayanus pasture in association with forage legumes Pueraria phaseoloides and Centrosema acutifolium over 4.79 months (30 days) and 4.85 months until CW calves were weaned on savanna in 1984 (Replicate 1) and 1985 (Replicate 2), respectively. What was also of interest was to know the savanna impact on production when stockers (weaned calves; 10.1 ± 1.71 months) were monitored during additional 14.46 months to become yearlings (24.0 ± 0.05 months). This particular combination of pure and crossed bred cattle, forages, environmental and management conditions portrays current local extractive and extensive farming regimes more extensively reported elsewhere (Vera and Hoyos 2018).
Modelling approach
Reproductive, productive, LW fluctuations and SRs values were subject to a mental conceptualization and enclosed mathematical representation (Tedeschi 2019) of CH4 emissions and energy (MJ) gas losses computed on an Excel* spreadsheet mechanistic model as described in detail by Ramírez-Restrepo and Vera (2019). Briefly, relevant measures included LW-derived ad libitum (2.1% of total LW; Fisher et al. 1987) dry matter intake (DMI; Eq. 1) during conception, gestation, calving, weaning and first dry period. In parallel, the LW-derived CH4 emission system after 56 days of age (Huws et al. 2018) identified the allocation impact of daily (g) emissions (Eq. 2) associated with key environmental performance indicators in terms of reproductive parameters, and intensity and carbon dioxide (CO2) equivalents (CO2-eq) efficiency indices. Fecal CH4 and nitrous oxide (N2O) emissions from cattle dung and urine as well as CH4 and N2O from soil are outside of the present calculations (Ramírez-Restrepo et al. 2019).
These environmental estimates used the 100-year horizon global warming potential of 34 for CH4 (Mueller and Mueller 2017), while bulls' data is not included in the flux matrix.
Predictive regression equations:
Eq 1. Dry matter intake (Ramírez-Restrepo and Vera 2019).
Eq. 2. Methane g/d (Ramírez-Restrepo and Vera 2019).
In case of future needs, fuelled by extreme changes, sensitive parameters in the model can be modified to simulate herds on the basis of the new structure. Nevertheless, it is essential to ensure relevant input data from extensive tropical conditions to achieve reliable key environmental and productive performance herd indicators.
Statistical analysis
The variance associated with treatment means was analyzed in SAS (2016) using the GLIMMIX procedure for measurements of LW, DMI and all derived CH emissions. Independent effects of replicates (RPs, i.e. 1 and 2; beginning in years 1984 and 1985, respectively), weaning practice (i.e. CW and EW), RCs (i.e. 1 and 2), the interactions between weaning practice and RC; and between RP, weaning practice and RC were fitted in a linear model using the random effect of cow.
The analysis was complemented using the MIXED procedure to assess the linear fitted effects of RP, weaning practice and the RP by weaning practice interaction on the post-weaning conception (dry) periods. Results are presented as least squares means ± standard errors of the means (LSM ± SEM), unless otherwise noted. Significant differences were calculated at the 0.05 probability level and specific p-values are shown when available.
RESULTS
In cows, DMI (kg/animal/d) was affected by the interaction (p < 0.05) between RC and weaning practices (Table 1). However, the interaction among RC x weaning practice and RP was dominant (p < 0.001) at the first conception and over the dry empty period (Table 1). Overall, during the two RCs, DMI in the CW and EW treatments was similar during conception (6.89 ± 0.114 vs 6.90 ± 0.107), gestation (7.11 ± 0.081 vs 7.04 ± 0.077), calving (7.66 ± 0.066 vs 7.54 ± 0.063), lactation (7.08 ± 0.069 vs 7.03 ± 0.065) and weaning (6.49 ± 0.090 vs 6.52 ± 0.084). The common grazing on savannas led to variation in calves' DMI between RPs (p < 0.01) due to weaning treatments and across all interactions (p < 0.0001). Averaged, DMI was larger (p < 0.0001) in CW calves (4.37 ± 0.037) compared to the EW (3.38 ± 0.035) calves.
TABLE 1 Calculated dry matter intake (kg/animal/d)‡ in cow-calf pairs of commercial Brahman (Bos indicus) and Brahman crossbred mixed hers subject to conventional weaning (CW) or early weaning (EW) savanna farming practices in two temporal replicates. Two consecutive conception-weaning periods were monitored in each replicate of each treatment.
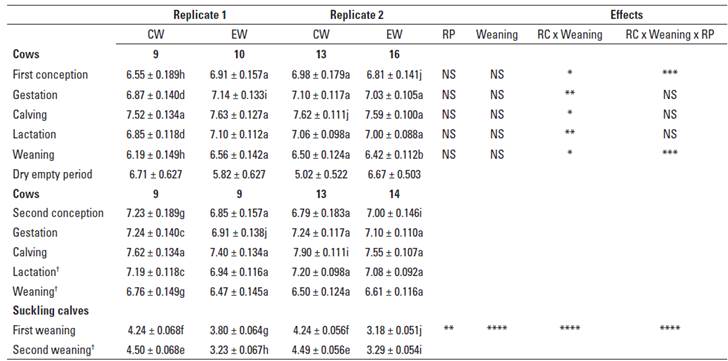
‡ Adapted from Ramírez-Restrepo and Vera (2019). †Modelled data. RC: Reproductive cycle. Values between similar parameters bearing different letters in the same column and RP are significantly different (ab: p < 0.05; cd: p < 0.01; ef: p < 0.001; gh: p < 0.0001; ij: p ≤ 0.10). Comparisons between RPs, weaning management and RC interactions in each row for each parameter are declared at *p < 0.05, **p < 0.01, ***p < .001, ****p < 0.0001, p ≤ 0.10.
NS: Not significant.
At conception, averaged differences of CH4 emissions between CW (123.05 ± 2.628 g/d) and EW (123.29 ± 2.462 g/d) cows were not significantly different, while overall emissions were lower (p < 0.05) in the first RC (121.38 ± 1.919 g/d) than in the second RC (124.97 ± 1.943 g/d). This resulted in a consistent (p < 0.001) RC x weaning practice x replicate interaction.
Table 2 shows CH4 emissions from cows in terms ofarea, day, animal units and indices of intensity and efficiency during gestations of two consecutive RCs. Differences in emissions between RPs and weaning routines were small, but the significative impact of the RC x weaning i nteraction on all parameters was of particular interest, and was particularly large in the case of the efficiency of emissions when the final LW (FLW) of the cows was considered. Estimates of total CH4. Emissions (kg) during the gestation period indicate that irrespective of the RC, CW and EW cows were equal emitters (37.86 ± 0.506 vs 37.47 ± 0.476), while values grouped in the first (37.38 ± 0.383) and second (37.94 ± 0.390) RCs were also similar.
TABLE 2 Calculated total methane (CH4) emissions and derived intensity and efficiency indices from commercial Brahman (Bos indicus) and Brahman crossbred cows during two consecutive reproductive cycles (RC) on neotropical savannas under conventional (CW) or early weaning (EW) farming practices.
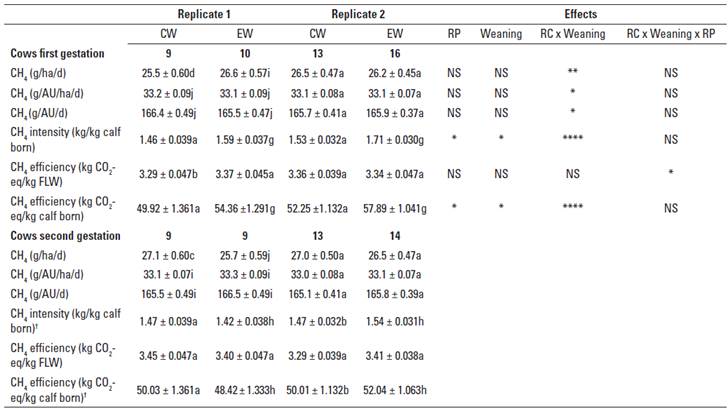
† Modelled data. AU: Animal unit equal to 450 kg. CO2-eq: Carbon dioxide equivalent. FLW: Final liveweight during the phase. RP: Replicate.
Values between similar parameters bearing different letters in the same column and RP are significantly different (ab: p < 0.05; cd: p < 0.01; ef: p < 0.001; gh: p < 0.0001; ij: p ≤ 0.10).
Comparisons between RPs, weaning management and RC interactions in each row for each parameter are declared at *p < 0.05, **p < 0.01, ***p < 0.001, ****p < 0.0001, p ≤ 0.10. NS: Not significant.
Cow CH4 emission profiles over the two lactation periods are presented in Table 3. The results show the model forecasted consistent and significative effects of the interaction between RC and weaning practices in terms ofeither daily emissions (p < 0.01) or indices' expressions (p < 0.0001). However, predicted intensity and efficiency indices considering cows' FLW over the lactations were significantly (p < 0.0001) affected by all of the considered effects. Averaged over the lactations, there was a large and practically important difference (p < 0.0001) in total CH4 emissions between CW cows (38.67 ± 0.456 kg) and EW (14.40 ± 0.435 kg) cows, while values between the first (26.74 ± 0.412 kg) and second (26.33 ± 0.424 kg) lactation were similar.
TABLE 3 Estimated methane (CH4) emissions and derived efficiency and intensity emission indices of two continuous lactations in multiparous cows grazing savannas subject to conventional weaning (CW) or early weaning (EW) farming routines in two temporal replicates.
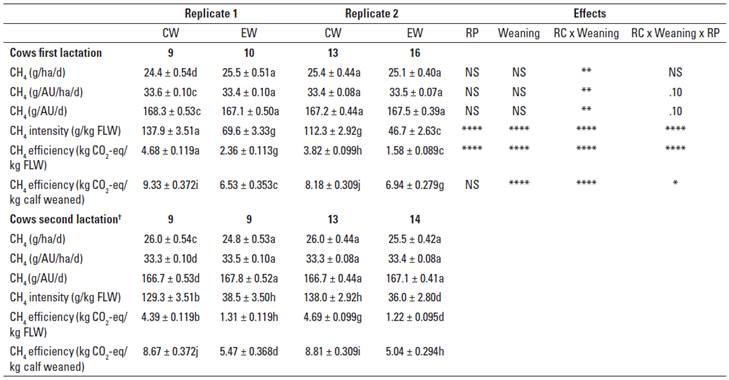
† Modelled data. AU: Animal unit equal to 450 kg. CO2-eq: Carbon dioxide equivalent. FLW: Final liveweight over the period. RC: Reproductive cycle. RP: Replicate.
Values between similar parameters bearing different letters in the same column and RP are significantly different (ab: p < 0.05; cd: p < 0.01; ef: p < 0.001; gh: p < 0.0001; ij: p ≤ 0.10).
Comparisons between RPs, weaning management and RC interactions in each row for each parameter are declared at *p < 0.05, **p < 0.01, ***p < 0.001, ****p < 0.0001, p ≤ 0.10. NS: Not significant.
Results from birth to yearling age indicate that all parameters of CH4 emissions were affected by the interaction of RC and weaning treatments, but again the inclusion of the RP effect in the interaction was dominant (p < 0.0001; Table 4). In parallel, the complete environmental impact was higher p < 0.001) in CW (43.11 ± 0.498 kg CH4) than in EW (40.27 ± 0.472 kg CH4) yearlings. Similarly, relative to the first RC (39.59 ± 0.402 kg), CH4 emissions were higher in the second RC (43.79 ± 0.410 kg).
TABLE 4 Effect of conventional weaning (CW) or early weaning (EW) savanna farming practices on derived methane (CH4) emissions and environmental indices in commercial beef calves form birth up to 24 months of age.
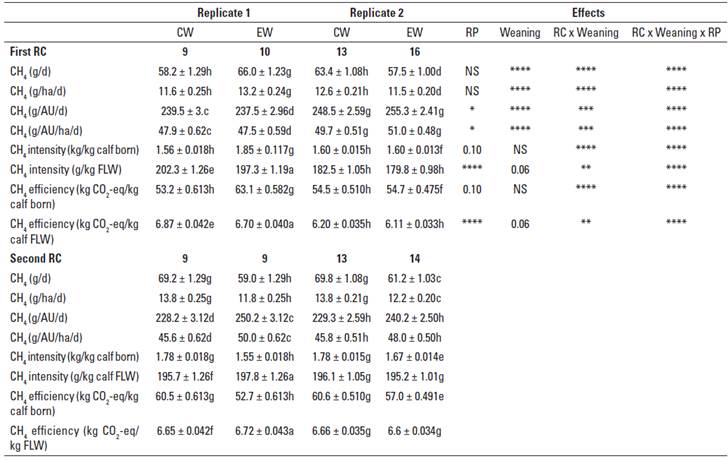
CO2-eq: Carbon dioxide equivalent. FLW: Final liveweight. RC: Reproductive cycle. RP: Replicate.
Values between similar parameters bearing different letters in the same column and RP are significantly different (ab: p < 0.05; cd: p < 0.01; ef: p < 0.001; gh: p < .0001; ij: p ≤ 0.10).
Comparisons between RPs, weaning management and RC interactions in each row foreach parameter are declared at *p < 0.05, **p < 0.01, ***p < 0.001, ****p < 0.0001, p ≤ 0.10.
NS: Not significant.
DISCUSSION
The retrospective analysis of this LW-derived CH4 flux research was designed to determine the impact of extensive cow-calfherd performance on enteric CH4 emissions and potentially contribute to abatement opportunities by considering alternative herd farming practices. The most significant finding was the interaction between RCs and farming weaning management practices as a critical factor to dynamically associate extensive cow-calf beef production and environmental knowledge. This outcome is in line with Donoghue et al. (2016) that indicated that in beef cattle multiple CH4 emission measurements on each animal may be required in the mid to long-term to accurately record CH4 traits such as DMI, daily CH4 (g) and CH4 yield (g/kg DMI) emissions.
Confirming the CH4 emission findings of Ku-Vera et al. (2018) and reflecting on the scale of our virtual representation, our mathematical model offers reliable and detailed carbon footprints to promote the combination of tactical farming decisions. This suggests what management practices to pursue, what stock classes to maintain, when to wean, what to sell and when to sell in order to be more efficiently and sustainably productive. In this sense, as suggested by Tedeschi (2019), the integration of further mental conceptualizations, field research, and virtual domains using previously accumulated local bodies of data and scientific knowledge allows complementary lines of inquiries. Thus, the detailed local systemic knowledge of the extensive beef environmental impact should support a potential improvement in the accuracy of the Colombian greenhouse gases (GHG) inventory (IDEAM 2016) regarding the Colombian Llanos in relation to animals' dynamic physiological conditions. To the best knowledge of the authors, the Colombian GHG livestock inventory relies on a standard Intergovernmental Panel on Climate Change [IPCC (2006)] Tier 1 approach that does not consider CH4 emission factors measured from native or adapted cattle to the local low tropics, but are derived from international temperate scenarios (56 kg CH4/head per year).
Collectively, our observations are in agreement with the cluster and metaanalysis study of Cottle and Eckard (2018) which noted that for GHG inventory reporting, country-beef specific estimates of daily and yield CH4 emissions are required from data generated in each country rather than that derived from the standard IPCC GHG account method. As noted by Cottle and Eckard (2018) a number of reasons may contribute to discrepancies between world and local accounting approaches including, but not limited to, the physio-metabolic interactional framework adopted in this paper.
Those discrepancies plus significative differences between temperate and tropical diets in the metabolizable energy intake and digestible energy intake ratio throughout the year are probably indicative of more accurate CH4 measurements in our study, while supporting evidence of the fact is also represented by the dynamic LW change in dams and calves to derive precise DMI and enteric CH4 emissions. The main physiological driver of CH4 production is DMI (Jonker et al. 2018), but this effect appears to be masked if the digestive effects of plant secondary compounds are considered (Ramírez-Restrepo and Barry 2005; Durmic et al. 2017; Vandermeulen et al. 2018).
In the context of present-day international trends and the controversies surrounding the environmental impacts of beef production (Henderson et al. 2017), veterinary medicine and animal sciences have an eloquent case for a stronger, more relevant role in efficient production, financial return, sustainability, climate change global discussions and within GHG emission and mitigation research (Ramírez-Restrepo and Charmley 2015). In concert with the latter consideration, CH4 is found in sheep (Ramírez-Restrepo et al. 2010) and cattle (Ramírez-Restrepo et al. 2016b) Jugular blood, but its metabolic and physiological systemic impact is still unknow in ruminants (Tedeschi et al. 2014; Ramírez-Restrepo and Charmley 2015; Ramírez-Restrepo et al. 2016a).
Such discussion is extremely pertinent since after gut fermentation of dietary fiber, luminal intestinal methanogenic archaea flora in methanogenic humans converts hydrogen gas to CH4, it diffuses into systemic circulation and the gas is linked to high body mass index (i.e. obesity; Basseri et al. 2012). Other CH4 nested case-control data indicate that human CH4. is associated with the pathogenesis of organic and functional bowel diseases, including but not limited to diverticulosis, colon-rectal cancer, inflammatory bowel diseases and irritable bowel syndrome (Pimentel et al. 2006; Roccarina et al. 2010; Pimentel et al. 2012; Rezaie et al. 2017). It is therefore possible that intrinsic blood CH4 is required to maintain threshold levels and anti-inflammatory signals, as in the canine immune system (i.e. leukocyte) activation that may be modulated by 2.5% CH4 ventilation (Boros et al. 2012).
Collectively, these metabolic data question whether some of the plausible interventions for lowering beef CH4 emissions in tropical environments (Ramírez-Restrepo and Charmley 2015) compromiso or not animal welfare. Relative to a basal diet, Ramírez-Restrepo et al. (2016b) demonstrated the significative effects of tea (Camellia sinensis L.) seed saponin supplementation on blood serum biochemistry of Brahman steers. This argument is further supported by Ramírez-Restrepo et al. (2014) who found in Belmont Red Composite [Africander (African Sanga) x Brahman x Hereford-Shorthorn (3/4 B. Taurus)] steers therapeutical complications of the digestive, muscular and urinary systems when fermented-Monascus purpureus red rice powder containing a natural lovastatin (monacolin K) was supplemented as an anti-methanogenic compound.
Therefore, it is particularly evident and necessary to combine human and animal clinical experiences to elucidate relevant medical questioning regarding interpretations, similarities, synergisms, and divergences of CH4 metabolism and gaps in knowledge. This should allow funding opportunities, research, teaching, national and international engagement. If this premise is accepted, this connectivity would lead to redefining aspects of the veterinary and animal science professions of the future and their social and global health relevance in the growing field of climate change.
Cows' LWs cycled between low values at weaning and higher LWs at calving, but the cycle was stable and tended to go back to the initial conception LWs in the following RC. This is a repeatable phenomenon observed in extensive breeding herds in northern Australia (Fordyce et al. 2013; Fordyce et al. 2014), and together with current savanna knowledge suggests possible entry points if the intention is to raise production levels. Nevertheless, the significant differences between successive RCs reflect the extreme sensitivity of savanna-based herds to between-years variations in environmental conditions.
The observed LWs at reconception (Table 5) showed moderate variability, as estimated by the confidence limits, and did not differ between CW and EW treatments. These LWs are similar to those reported by Vera (1991; 282-300 kg) for the first conception of heifers grazing Brachiaria humidicola, and are slightly below the LWs at the second to fourth conception recorded by Vera et al. (1993; 325-340 kg) for cows grazing that same pasture. They therefore appear to be the lower limit for viable conceptions carried to term in cattle of the same phenotype as used here, and extrapolating from Vera et al. (1993) they are also dangerously close to the calculated asymptotic LW allowed for on low quality rangelands. These values can be compared with the mature body sizes of well-fed and highly fertile cows grazing well managed B. de-cumbens pastures, that ranged between 388 and 452 kg (Vera et al. 2002). The difference between the savanna-based cows presently reported, and those of the well-fed animals on B. decumbens, and other improved Brachiaria cultivars (Peters et al. 2013) therefore indicate the magnitude of the nutritional challenge faced by extensive breeding herds based exclusively on native savannas.
TABLE 5 Liveweight(LW) and relevantreproductive events in extensive beefbreeding herdsfollowing conventional weaning (CW) and early weaning (EW) practices.
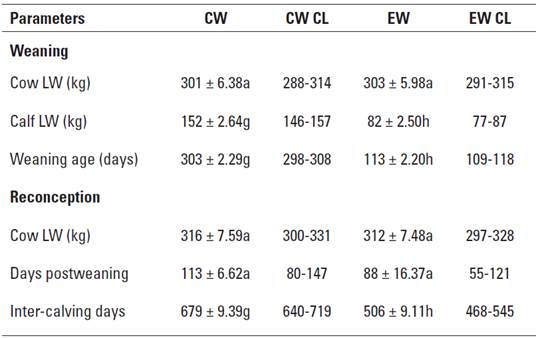
CL: Confidence limits. Values between weaning treatments bearing different letters in the same row are significantly different (ab: p < 0.05; cd: p < 0.01; ef: p < 0.001; gh: p < 0.0001).
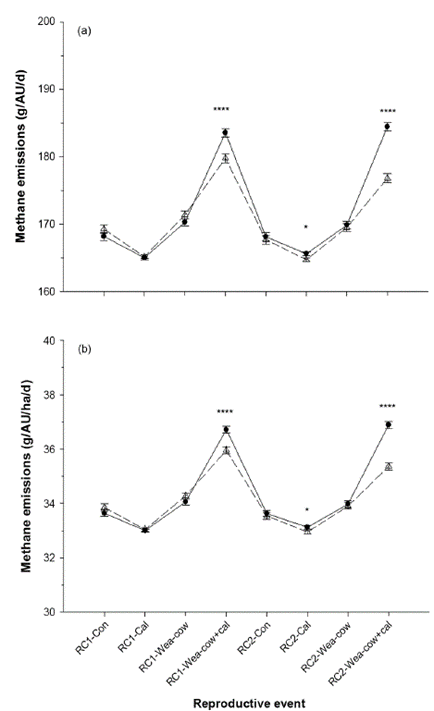
FIGURE 1 Cow and cow plus calf methane emissions in animal units (AU equal to 450 kg live weight) from conventional weaning (∆ short dash line) and early weaning (• solid line) breeding beef herds over two consecutive reproductive cycles (RC1 - RC2) at conception (Con), calving (Cal) and weaning (Wea) days. Bars represent SEM (*p < 0.05, ****p < 0.0001).
Notwithstanding mineral deficiencies (Lebdosoekojo et al. 1980; Depablos et al. 2009), low LWs and energy and protein deficit intakes (Ramírez-Restrepo and Barry 2005; Ramírez-Restrepo et al. 2005), and the effect of lactation and suckling constitute the major constraints to efficient reproductive performance (Osorio-Arce et al. 2002; Delgado et al. and Heamden 2017). These factors probably explain the long inter-calving periods reported in Table 5. The large difference in the length of that period between EW and CW treatments support the contention that under these circumstances, long suckling phases negatively affected the output of breeding herds.
Not surprisingly, long inter-calving periods and variable LWs were associated with the total CH4 output of complete RCs, leading to low environmental efficiencies per ha and per calf born (Tables 2-4), values that are low per ha only because of the low SR supported by the savanna, but are high if a total system approach is pursued as demonstrated by graphical representation of reproductive events over time (Figures 1a and 1b). A potentially important contributor to the emission of GHG is the N outputs of grazing animals, given the large effect of N2O and other nitrogenous compounds (IPCC 2006), but given the low SRs used in the savannas, this effect was not accounted for in the present results.
CONCLUSIONS AND FUTURE DIRECTIONS
As technology, modelling and climate variability influence the way that people live, eat, learn and work, by their very nature, veterinary medicine and animal science fields must dynamically adapt to the challenging needs of livestock production and societal pressure. Thus, based on real-farm situations this paper differentiates annual CH4 estimates (kg/head) between RC1 and RC2 in terms of gestation plus lactation (i.e. breeding; 47.19 ± 0.380 vs 34.75 ± 0.386; p < 0.0001), commercial weaners (14.11 ± 0.163 vs 15.25 ± 0.167; p < 0.0001), stockers-yearlings (25.12 ± 0.233 vs 26.96 ± 0.237; p < 0.0001) and yearlings (20.65 ± 0.196 vs 21.86 ± 0.199;p < 0.0001). Such systems' values argue that our field research-based digital model has portraited the distance between reasonable CH4 emissions from extensive cow-calf extensive beef herds and the Latin American IPCC (2006) default CH4 emission factor. The noteworthy difference emphasizes the need to maintain or carefully move towards a more productive-driven system using our agriculture-animal science practice and ecosystem veterinarian focus. This implies a sound understanding of the ecology of reproductive performance and meat production at the interface of native-introduced pastures, data analytics and environmental farm management. The combined approach should help further address the carbon foot printing of beef herds on the Colombian Llanos and the growing social determinants of sustain-ability and health in the context of farming systems thinking.