1. Introduction
Cold seeps are places on the ocean floor with leaks of hydrocarbon-rich fluids, mainly methane, that come from subsurface seafloor sediments (Ortakand et al., 2016) and occur widely worldwide along active and passive continental margins (Sibuet & Olu, 1998). According to Judd & Hovland (2007), methane seeps can be identified by visualizing bacterial beds of grayish colorations, precipitates of authigenic carbonate, and communities of clams of the genus Calyptogena. These emission sites have been gaining importance from both a geological and biological points of view, especially for their potential role in climate change, since methane is a greenhouse gas (Panieri, 2006).
One of the problems investigating methane emissions in the sedimentary record has been the scarcity of well-defined indicators that can be used to establish the chronology of such events. These has led to the search for tools to identify old and recent emissions (Panieri et al., 2009; Panieri, 2006). The lack of data that allowed to evaluate the influence of methane fluxes on geochemistry or on the faunal characteristics of benthic assemblages associated with each filtration (Rathburn et al., 2000), meant that in the last 20 years the contributions to the knowledge of these indicators increased significantly.
One of the tools explored by scientists in the last decade has been the shells of recent calcareous foraminifera and fossils, since they have allowed us to know the history of seeps (Panieri et al., 2017). Studies of foraminifera are helpful indicators of local seafloor and sediment methane emissions (Panieri et al. 2016; Wang et al. 2012). Rathburn et al. (2003) suggested that a better understanding of the ecology of foraminifera and their isotopic composition may improve knowledge of paleo methane emissions and interpretations of climate and paleoceanographic change. It has been seen that this group of organisms can be abundant in seepage habitats, but no endemic species have been found (Bernhard et al., 2001; Rathburn et al., 2000; Sen Gupta et al., 1997). Some species that feed on nutrients provided by endosymbiotic prokaryotes can survive in sulphurous and anoxic environments, although excessive emissions can become toxic to most foraminifera (Panieri, 2005; Rathburn et al., 2003; Bernhard et al., 2001; Sibuet & Olu, 1998). Foraminifera carbonate shells in cold seep areas have also been found to have values of δ 13C very negative compared to sites where there are no seeps because of the influence of methane present around them (Wang et al., 2012; Hill et al., 2004; Rathburn et al., 2000). Foraminifera communities along with geochemical indicators have been analyzed to reconstruct past episodes of gas and methane seeps, which has led to understanding the dynamics of emissions in the present (Zhang et al., 2018; Pan et al., 2017; Sztybor & Rasmussen, 2017; Panieri et al., 2016).
Modern environments, in which methane gas migrates through porous sediments, are geographically widespread and are ideal modern habitats for examining the influence of methane seepage on living benthic foraminifera (Judd, 2003; Sibuet & Olu, 1998). According to Panieri (2006) foraminifera have high densities in the exhaust zones despite the lack of oxygen and sulfur content on the environment. These changes in oxygen concentrations at the sediment-water interface and their morphological characteristics are reflected in the size, wall thickness, porosity, and taxa (genera and species) of presented foraminifera. Some morphological and taxonomic differences have been quantified as a dissolved oxygen index (FBOI) (Kaiho, 1994), which, together, with the change in the abundance, diversity, density, and dominance of this group provides information on oil release events or spills in marine reservoirs (Schwing et al., 2017; Pan et al., 2017; Panieri, 2005).
The results of the studies and the effectiveness of the use of foraminifera as indicators depend on the methodologies used and their limitations. For example, the availability of remotely operated vehicles (ROVs) allows the exact location of sampling to be selected. Similarly, several authors have mentioned the limitations of the use of rose Bengal to distinguish between living and dead specimens (e.g. Gooday et al., 2013; Rathburn et al., 2000). Therefore, the objective of this work is to review the different methodologies used for the study of foraminifera in surface and subsurface sediments of current filtration environments, to understand what they depend on and how they contribute to obtaining specific results. With this information, there is a methodological proposal for the Colombian Caribbean with the available technology.
2. Development and discussion
Fig. 1 shows a summary of the different field and laboratory methodologies used in the study of foraminifera related to hydrocarbon environments, from the identification of sampling sites to the processing of samples in the laboratory. Each of them will be explained later.
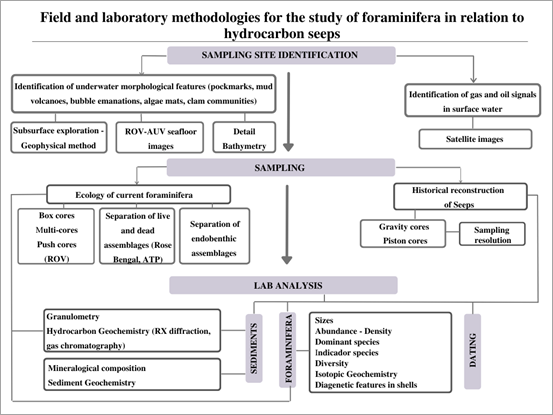
Source: The authors
Figure 1 Conceptual map of field and laboratory methodologies for the study of foraminifera in hydrocarbon filtrations.
2.1. Identification of sampling sites
During the last three decades, the areas of seepage have been indirectly identified by marine geomorphological features such as pockmarks, emanations, mud volcanoes, chimneys, bacterial mats, carbonate calcretes, and other characteristics associated with the flow of fluids on the seafloor (Basso et al., 2020; Judd, 2003; Rathburn et al., 2000). The pockmarks have been one of the most outstanding geomorphological features for sampling in a vast majority of studies (Dessandier et al., 2019, 2020; Fontanier et al., 2014; Hill et al., 2003).
These were discovered in the sixties after the advent of acoustic mapping (King & Maclean, 1970). They are depressions of approximately 5 m in diameter that can be located alone, in groups, or in chains, representing the most recent and active filtration sites (Hovland et al., 2010). These structures have been investigated with geophysical studies (shallow seismic data of high resolution) and chemicals, which in turn have allowed the identification of other associated intra-sedimentary structures, such as pockmarks, fossils, and domes (Judd & Hovland, 2007). Currently, the study of seeps includes high-resolution bathymetries along with seismic and acoustic analyses the recognition of pockmarks on the sea floor, filtration plumes on the water column, or acoustic bleaching, among others (Schroot et al., 2005). These filtrations, together with 3D seismic exploration, allow us to understand the relationships between the geological complexity of the seafloor and the structure of fluid migration pipelines (Nagakubo et al., 2007). Additionally, ROVs have been increasingly used for seafloor imaging, which identifies benthic communities and bubble leaks, as well as for sampling (Dessandier et al., 2019, 2020; Burkett et al., 2018; Panieri et al., 2014; Gieskes et al., 2011; Sen Gupta et al., 2007).
2.2. Sampling
2.2.1. Ecology of current foraminifera
Studies of live and dead assemblages of foraminifera in surface sediments are carried out to identify the influence of methane seeps on their ecology and geochemistry (Gieskes et al., 2011; Hill et al., 2004). The ecology of benthic foraminifera (FB) in cold seeps remains uncertain, mainly because their ability to develop in the active phases of seeps has not yet been identified (Dessandier et al., 2019). Likewise, an impoverishment in δ¹³C has been demonstrated in foraminifera associated with methane filtrations, the calcification process of foraminifera during discharge periods registers a high variability in the isotopic values of live and dead foraminifera (Torres et al., 2003), which makes it necessary to continue investigations of the isotopic composition of current foraminifera at different levels of the sediment.
2.2.1.1. Sampling instruments: Surface samples, short cores (box corer or multi corer) and thrust cores (ROVs or Submersibles)
The use of these instruments depends on the depth of sampling, its associated characteristics, and the type of fauna to be extracted. Most of the exhaust zone studies extract their sediment samples with ROVs (Basso et al., 2020; Burkett et al., 2018; Bernhard et al., 2001). The use of ROVs and submersibles allow the collection of sediment samples present in clam beds of diameters smaller than one meter at different depths (Gieskes et al., 2011). Some ROVs are equipped with colonization cages for the extraction of epibenthic organisms called adhesion cubes (Burkett et al., 2016). Others feature positioning sensors that allow the exact location of box cores and extraction tubes with suction devices for the recovery of sediments from the seafloor (Sen Gupta & Aharon, 1994). Others use camera arms to obtain sediment cores (McGann & Conrad, 2018; Bernhard et al., 2001).
Box cores vary in dimensions according to the objectives of the study and are generally subsampled for microfauna analyses (Basso et al., 2020). They are used to sample the first centimeters of surface sediment since this tool allows the preservation of the sediment/water interface for the analysis of foraminifera and their geochemistry (Machain-Castillo et al., 2019). Another tool like box cores are multi-nucleators, which allow sampling of surface sediments, bottom-pervading waters, and the water-sediment interface (Schwing et al. 2017; Fontanier et al., 2014; Gooday et al. 2013). As for the thrust cores, these are collected using ROVs and have the advantage that they allow to visualize the marine floor and know exactly the sampling place with respect to the filtrations or indicative geo forms (Dessandier et al. 2019, 2020; Lobegeier & Sen Gupta, 2008). For sampling of current foraminifera studies, it is common to use control nuclei to make comparisons between active and non-active emission zones (e.g. Panieri et al., 2014).
2.2.1.2. Separation of living-dead associations
For the identification of living associations, different staining techniques have been implemented, such as rose bengal (rB), black sudan B (SbB) and other cytoplasmic distinction techniques, such as the biochemical method of Adelosine triphosphate (ATP) and the examination of internal structures from the transmission electron microscope (TEM) (Bernhard, 2000). The rB method consists of protoplasm staining to determine the presence of cytoplasm in the shells of foraminifera (Bernhard, 2000). This method is currently implemented in recent cold exhaust studies (Dessandier et al., 2019, 2020; McGann & Conrad, 2018), which conform to the mandatory recommendations of the sampling protocol for FB biomonitoring studies (FOBIMO, Schönfeld et al. 2012). In this protocol it is suggested that for the preservation and staining of the samples, the rB be added to the preservative (2 g / l) before adding the dilution to the sample, considering that the volume of the preservative is at least equal to the volume of the sample, to allow the sediment to stain for a minimum time of fourteen days (Fontanier et al. 2014). The implementation of this method varies in terms of the concentration of the rose Bengal and the staining time of the samples. Studies such as that of Panieri et al. (2014) used 1g/l of rose Bengal and allowed the samples to stain for 48 hours, while others such as Etiope et al., (2014), Hill et al. (2004) and Panieri et al. (2014) used 1g/l for one week.
However, the method of rB has often been questioned due to several factors (Bernhard, 2000), among which it is highlighted that the cytoplasm of FB can remain in the shell of the dead individual even after several weeks (Gooday & Rathburn, 1999; Bernhard, 1989). Thus, studies such as that of Bernhard et al. (2001) and McGann & Conrad. (2018), in addition to using rB staining, they used the ATP method. This allows to observe the internal structure and quantify the biomass of the foraminifera through the photoluminescent reaction between the preservative and the luciferase enzyme (DeLaca, 1986). Despite these questions, authors such as Rathburn et al. (2000) indicate that the rB staining method is one of the most practical to distinguish live specimens in large quantities, in addition to the fact that the data generated are comparable with the results of previous studies since the effects that other staining techniques would have on the isotopic composition of carbon in the shells of stained foraminifera are not yet known.
2.2.1.3. Endobenthic separations
For the study of infaunal foraminifera, the methodology consists of selecting the first 5 cm surface of the sediment and then subsampled at intervals of 1 cm (Dessandier et al., 2020), 0.5 cm (Martin et al., 2010) or at variable intervals (e.g. 0.5 cm to 3 cm and 1 cm to 10 cm, Rathburn et al., 2000). There are cases such as that of Fontanier et al. (2014) in which they divided a first nucleus into intervals of 1cm from the water/sediment interface to 3 cm deep, and an additional core every 0.5 cm from the water-sediment interface to 2 cm and every 1cm between 2 to 5 cm deep. In terms of depth and sampling resolution for biomonitoring studies there are recommendations (Schönfeld et al., 2012) where it is indicated that it is necessary to define a level 0 with the intermediate point of any irregular sediment surface, in such a way that the level 0-1 cm of shallowest sediment extracted is sampled. Additionally, it is recommended that the sample has a volume of 50 cm³ to optimize the extraction of live foraminifera.
2.2.2. Historical reconstruction of seeps
Sediments extracted from the ocean floor serve as a timeline for the reconstruction of events throughout geological history. The study of paleo-filtrations making use of foraminifera in recent times has focused on topics such as: reconstruction of methane emissions on the seafloor (Pan et al., 2017; Schneider et al., 2017; Panieri et al., 2014, 2016; Millo et al., 2005), reconstruction of the history of basins with gas hydrates (Menezes, 2011; Ingram et al., 2010), records of deep-sea variation influenced by methane seeps (Sztybor & Rasmussen, 2017), and paleoenvironmental comparisons between areas with and without gas hydrates (Zhang et al., 2018; Ortakand et al., 2016).
The most used instruments for the development of these investigations have been gravity cores and piston cores since they allow collecting more sediment in the vertical. The extraction sites of these cores are located within the filtration area, at exact emission points or nearby points (control points), depending on the objective of the investigations. For example, Sztybor & Rasmussen (2017) collected more than one gravity and piston core in different years, inside and outside the emission point. For their part, Ingram et al. (2010) collected ten gravity cores around a cold gas hydrate filtration field, with the aim of investigating the sediment of the filtration area and possible disturbances in the vicinity of the field. The sampling of these cores generally varies according to the objectives of the investigations and the lithological changes observed with the naked eye (color, grain size, changes in fauna and the number of sediments recovered) (Ortakand et al., 2016; Wang et al., 2012; Millo et al., 2005). Most of the reviewed articles sampled at 5 cm or 10 cm intervals (Pan et al., 2017; Schneider et al., 2017; Sztybor & Rasmussen, 2017; Ingram et al., 2010; Millo et al., 2005), but others varied the sampling resolution by having areas of interest in the cores. For example, Baas et al. (1998) sampled every 2 cm in the layers related to Heinrich events to observe oxygenation values with FB, and Wang et al. (2012) also chose 2 cm intervals in sections of the cores that showed discoloration changes. In studies such as that of Panieri et al. (2016) the sampling resolution was higher (1 cm) in order to make a detailed reconstruction of paleo-methane emissions.
2.3. Laboratory Analysis
2.3.1. Foraminifera
When studying recent and past foraminifera, the methodological procedure has some variations that are exposed in this chapter. Table 1 provides a summary of the methodologies mostly used in the literature for the analysis of foraminifera in the laboratory.
2.3.1.1. Size of fraction analyzed
For both surface and subsurface samples, the fraction used for foraminifera analysis tends to be variable and largely depends on the number of foraminifera in each fraction. For the study of foraminifera in surface samples, the size of the fraction is chosen depending on the stained foraminifera found (Sen Gupta & Aharon, 1994). Studies such as those of Basso et al. (2020) analyzed foraminifera from the fraction of 250 μm since this fraction presented a greater record of stained live specimens. Studies focused on escapes for surface samples recommend studying foraminifera from the fraction 125 μm, since stained specimens tend to be more abundant (Rathburn et al., 2000). This coincidence of what is recommended in the protocol for biomonitoring benthic communities (Schönfeld et al., 2012), which defines that for infaunal analyses that the study be based on the faunal inventory found in the fraction > 125 μm; However, it is not unknown that for some environments it may be necessary to analyze the >63 μm fraction.
As for the foraminifera of the subsurface samples, the most used fractions have been those of 100 μm, 125 μm, 150 μm, and 250 μm since it is considered that a record of native fauna can be obtained in these fractions (Zhang et al., 2018; Ortakand et al., 2016; Panieri et al., 2016; Wang et al., 2012; Baas et al., 1998). Some studies use larger fractions (300 μm and 500 μm) to select large specimens for stable isotope analysis (Panieri et al., 2016). The 63 μm fraction has also been analyzed in some studies (Ingram et al., 2010; Millo et al., 2005), but it is considered that smaller species belonging to a shelf or slope areas may have been displaced by transport.
Table 1 Summary of laboratory analysis for the study of foraminifera in filtrations.
FB y FP | Size scanned (μm) | Abundance | Density | Dominant species | Indicator species | Diversity | Isotopic geochemistry (δ¹⁸O and δ¹³C) | Diagenetic traits in shells | Dating |
---|---|---|---|---|---|---|---|---|---|
Current | > 63, 150, 125, 250 | # Specimens* gram | # Specimens*cm³ | Calculation of dominances in percentages - Bases for taxonomic classification - Relationship between orders of foraminifera - Cluster analysis | Oxygenation indicator species through the FB oxygenation index (FBOI) | Shannon-Wiener Index/Pielou Equity Index, Simpson Index, Fisher's Alpha Index | Mass spectrometer | Photographs of images with digital camera and Scanning Electron Microscope (SEM) -Electron probe microanalysis (EPMA)-Transmission electron microscopy (TEM) - Secondary ion mass spectrometry (SIMS). | Not Applicable |
From the past | > 63, 100, 125, 150, 250. | Carbon 14. By Spectrometric Mass with Accelerators (AMS) |
Source: The authors. Therefore, the results may have a considerable benthic signal bias (Baas et al., 1998).
2.3.1.2. Abundance and density parameters
Research such as those of Sen Gupta et al. (1997), Rathburn et al. (2000), Bernhard et al. (2001), Torres et al. (2003) and Burkett et al. (2016) study the correlation between benthic foraminifera communities and hydrocarbon emissions in terms of parameters such as abundance, diversity, composition, and dominant species. The calculation of abundance and density is used to facilitate comparisons of the vertical distribution patterns of foraminifera in both surface and subsurface sediment. Most studies in foraminifera report abundance as the number of individuals per gram of sediment (Ind/gr) (Machain-Castillo et al., 2019) and density as the number of individuals per unit volume (Ind/cm³) (Rathburn et al., 2000). Studies such as that of Dessandier et al. (2019) calculate foraminifera densities as the number of individuals per 1 cm of the sediment layer.
2.3.1.3. Dominant species
All studies of foraminifera in seepage environments separate, classify, and count species, to find characteristic associations or indicator species of these environments. According to the standard protocol, at least 300 specimens must be extracted per sample (Fatela & Taborda, 2002). Even so, the extraction of between 200 and 500 foraminifera per sample has been recorded, in both surface and subsurface samples (Machain-Castillo et al., 2019; Schwing et al., 2017; Sztybor & Rasmussen, 2017; Panieri et al., 2014). Usually, 300 foraminifera are searched, and the totals are counted in the samples where it is not possible to obtain this amount (Panieri et al., 2009).
Once the separation and counting of specimens in the chosen fractions have been carried out, the taxonomic classification is performed. The identification of taxa by genus and species has been done following the different classifications of Loeblich & Tappan, catalogs such as Ellis and Messina, the World Register of Marine Species (WORMS, http://www.marinespecies.org/), and/or the Integrated Taphonomic Information System (ITIS, for its acronym in English, http://www.itis.gov/) (Machain-Castillo et al., 2019; Sztybor & Rasmussen, 2017; Panieri et al., 2016; Schönfeld et al., 2012).
Cluster analysis and Q-Mode analysis factor have been the most widely used methods for grouping species into characteristic associations (Machain-Castillo et al., 2019; Ortakand et al., 2016). For example, in Lobegeier & Sen Gupta (2008), associations were obtained from cluster analyses with calcareous species, grouping them from Ward's minimum variance method.
2.3.1.4. Indicator species
Numerous studies have attempted to demonstrate that benthic foraminifera are useful indicators of local methane emissions (Wang et al., 2012), considering them a useful indirect tool when studying these emissions (Panieri et al., 2014). However, direct methane indicator species have not yet been identified, but associations of oxygenation indicator foraminifera have been used (Kaiho, 1994). Oxygen reduction in cold filtration environments is related to the anaerobic oxidation of methane and reduction of gases carried by filtration fluids, which may lead to an increase in the number of hypoxia-tolerant species (Wang et al. 2012).
Kaiho (1994, 1999) developed a benthic foraminifera oxygen index (BOI) that has been widely used in methane seepage studies (Zhang et al., 2018; Pan et al., 2017; Panieri et al., 2014). This index calculates the ratio between the number of oxic, suboxic, and dysoxic foraminifera species (Eq. 1 and 2).
When or > 0,
Table 2 Associations of foraminifera species indicators of oxygenation conditions. Information taken from Kaiho (1994) and modified for this work.
FBOI | Brief morphological description | Indicator species |
---|---|---|
Oxic indicators (3-6 ml/l O2) | Thick-walled epifauna in bottom water with high oxygen content | Cibicides spp., Cibicidoides havanensis, Cibicidoides kullenbergi, Cibicidoides mundulus, Cibicidoides robertsonianus, Cibicidoides wuellerstorfi, Cibicidoides spp., Globocassidulina subglobosa, Laticarinina pauperata, Pyrgo murrhina, Quinqueloculina spp., y Triloculina spp. |
Suboxic indicators (0.3-1.5 ml/l O2) | A. Small specimens of oxic species (<350 μm) | |
B. Epifaunal and infaunal inhabitants in high oxygen bottom water conditions who are commonly inhabitants of epifauna in low oxygen bottom water conditions | Alabamina spp., Astrononion pusillum, Bolivinita quadrilatera, Bulimina striata, Cancris inaequalis, Cassidulina spp., Ceratobulimina pacifica, Dentalina spp., Ehrenbergina pacifica, Eilohedra nipponica, Favocassidulina favus, Fissurina spp., Gyroidina spp., Gyroidinoides spp., Hoeglundina elegans, Lagena spp., Lenticulina spp., Melonis spp., Nonion spp., Oridorsalis spp., Pullenia spp., Rosalina columbiensis, Sphaeroidina bulloides, Stainforthia apertura, Tosaia hanzawai, Trifarina spp., Uvigerina spp. y Valvulineria spp. | |
C. Thin-walled foraminifera between the indicators of group B and dysoxic conditions | Bulimina aculeata, Elphidium excavation y Nonionella spp. | |
Dysoxic indicators (0.1-0.3 ml/l O2) | Thin walled, elongated, flattened foraminifera with better capacity of oxygen intake. Typical infaunal species. | Bolivina decussata, Bolivina interjuncta, Bolivina pacifica, Bolivina pus ilia, Bolivina robusta, Bolivina seminuda, Bolivina spissa, Bolivina spp. Bulimina exilis, Cassidulina tumida, Chilostomella oolina, Chilostomella ovoidea, Dentalina spp., Fursenkoina complanata, Fursenkoina rotundata, Globobulimina affinis , Globobulimina auriculata, Globobulimina pyrula, Gyroidina multilocula, Rutherfordia spp. y Suggrunda eckisi. |
Source: The authors
When O = 0 and SD > 0
O = oxic indicator specimens, S = suboxic indicator specimens and D = dysoxic indicator specimens.
Table 2 shows the associations of foraminifera indicators of oxidic, suboxic and dysoxic conditions established by Kaiho (1994) in his research on dissolved oxygen levels in modern oceans.
2.3.1.5. Diversity parameter
Diversity indices are performed in seepage studies to characterize foraminifera populations in emission environments or normal environments. The most common used indices in the literature include:
Shannon-Wiener index (Eq. 3) that measures the heterogeneity of species in each sample (Shannon, 1948).
Where: s is the number of species per sample and pi is the ratio of individuals of species i to the total number of individuals (i.e. the relative abundance of species i). The higher the value of H', the greater the diversity of species.
Fisher's alpha index (Eq. 4) providing a species richness parameter (Fisher et al., 1943).
Where: S is Wealth, is ∝ Fisher's Alpha and N is the number of individuals in the sample.
Pielou Equity Index (Equitability index, Eq. 5) which measures equity as a function of the number of species (Marrugan, 1988).
Where: H' is the Shannon index and S is the total number of species. The value of this index is from 0 to 1, so that 1 is all equally abundant species and 0 explains the absence of uniformity.
Simpson index (Eq. 6): Measures richness and dominance in a set of species (Somerfield et al., 2008).
Where: 𝑛𝑖 is the number of individuals in the 𝑖th species and N the total number of individuals. The higher the value of S, the lower the dominance of a group of species or a single species.
Diversity indices have been implemented to characterize diversity in specific centimeters or variability of this along the core to make comparisons between intervals before and after the events of hydrocarbon intrusions in the subsurface. For example, Schwing et al. (2017) documented post-2010 spill biota recovery in the Gulf of Mexico (Deepwater Horizon), where density and diversity recovery were likely caused by a predominance of opportunistic taxa that were able to thrive on high organic carbon deposition and oil concentration.
2.3.1.6. Isotopic geochemistry (δ¹⁸O and δ¹³C)
Studies of modern methane seeps have shown that foraminifera may be able to record the highly negative composition of δ¹³C of methane-influenced dissolved inorganic carbon in the bottom and interstitial waters (Hill et al., 2003; Rathburn et al., 2003; Rathburn et al., 2000; Sen Gupta et al., 1997). Thus, estimates of seepage activity and intensity can be obtained by comparing stable isotope data from specimens (Zhang et al., 2018; Rathburn et al., 2000). Isotopic analyses of carbon and oxygen in foraminifera (living and dead) have been performed with an analysis of Spectrometry of Masas (AMS). The selected shells must first undergo cleaning in an ultrasonic bath in methanol for 5 to 10 seconds to remove impurities and authigenic carbonates (Zhang et al., 2018). The fractions and grams selected for this analysis are variable. Some studies selected the most dominant taxa of the > fraction 125 μm (McGann & Conrad, 2018) and others chose the 250 μm and 400 μm fractions (Millo et al., 2005). As for the number of shells, most studies have chosen between 5 and 10 mg of foraminifera. The number of individuals depends on the size, and chosen species (Pan et al., 2017; Panieri et al., 2016; Ingram et al., 2010). All studies measure isotopes in benthic foraminifera, and some also measure them in planktonic foraminifera. Species are chosen for being abundant and common in the study areas.
2.3.1.7. Diagenetic alteration in shells
Low δ¹³C values in foraminifera associated with seeps may be due to primary biomineralization environments or secondary overgrowths of authigenic carbonate in shells (Panieri et al., 2017). For this reason, most of the studies have attempted to identify carbonate overgrowth in foraminifera shells to avoid inadequate measurement of isotopic concentrations. As well as the study of the diagenetic alteration in the shells of benthic foraminifera allows for refining the reconstructions of methane filtrations (Schneider et al., 2017).
The most commonly used methods of observing the wall in the literature have been: a) the binocular magnifying glass in order to observe traces of carbonate incrustation in specimens (Sen Gupta & Aharon, 1994), b) the use of images taken by scanning electron microscopy (SEM) (Bernhard et al., 2001) or images taken with a digital camera coupled to the stereomicroscope (Schneider et al., 2017), c) Electron Probe Microanalysis (EPMA), d)Transmission Electron M Hydroscopy (TEM) and e ) Secondary Ion Mass Spectrometry (SIMS) (Panieri et al., 2017). Although some methods are still under investigation, visual analyses of shells are insufficient, since interior structures and features at the micrometer scale can be overlooked, so Schneider et al., (2017) recommend performing conservation analysis and diagenetic alteration in shells before geochemical analysis, especially where authigenic carbonate precipitation occurs.
2.3.1.8. Dating
The most used method to date calcareous foraminifera in filtration areas or surrounding areas has been Carbon 14 dating through the Accelerator Mass Spectrometry (AMS) technique. Planktonic foraminifera have been selected more frequently for analyses (Pan et al., 2017; Menezes, 2011; Baas et al., 1998). The amount of foraminifera ranges from 10 to 2000 specimens (in some cases weight between 1.3 and 10 mg is indicated). Some use a single species (Baas et al., 1998) but others, due to low abundance, have chosen to mix species (Panieri et al., 2016). When planktonic foraminifera are scarce, dating can combine planktonic and benthic (Zhang et al., 2018), or benthic of one or more species (Panieri, 2014, Wang et al., 2012). Some studies, in addition to foraminifera, use wood fragments, radiolarian associations, and tephra clasts (Ortakand et al. 2016). Others complement radioisotope dating with biostratigraphy of foraminifera and nanofossils (Ingram et al., 2010).
2.3.2.1. Granulometry
In the studies, after sampling, most of the collected samples underwent freeze-drying, weight measurements, and immersed in sodium hexametaphosphate solution to disintegrate the particles (Zhang et al., 2018; Schwing et al., 2017). Subsequently, the sediment samples were wet sieved in the fraction of 63 μm to obtain the remaining sediment (sand size) and then dry. This procedure allows to calculate the percentage of sludge of the samples, which is an indicator of the size of the grain. Subsequently, the sand-sized particles are separated in the granulometric intervals according to the Wenworth classes, to recover the shells of the foraminifera in the selected sizes. We found no studies detailing sediment granulometric parameters.
2.3.2.2. Geochemistry of hydrocarbons
Gas chromatography is one of the most widely used methods for establishing the nature of hydrocarbons and geochemical analysis (Panieri, 2005). This method allows to measure the presence of polycyclic aromatic hydrocarbons (HcsAP), volatile and semivolatile Hcs, monoaromatic components and phenols in sediments based on EPA gas protocol methods and Mass spectrometry (GC/MS) (Schwing, et al., 2017; Etiope et al., 2014). Other studies performed this same analysis for non-condensable organic components (CH4 and C2-C8 hydrocarbons) (Panieri, 2006).
2.3.2.3. Mineralogical composition
The most widely used method for mineralogical analysis in the literature is X-ray diffraction, where minerals such as quartz, feldspar, pyrite, calcite and clay can be identified by diffractograms (Menezes, 2011). In Fontanier et al. (2014) this same method was used to characterize the nature of crystalline phases, their structure, degree of crystallinity, amount of amorphous content, microstructure and size of mineralogical crystals, and organic matter.
3.3.2.4 Sediment geochemistry
Changes in dissolved oxygen concentration at the sediment-water interface and related factors, such as organic carbon content in surface sediments, appear to play an important role in controlling the composition of benthic foraminifera assemblages and morphotypic characteristics, including shell size, wall thickness, and morphology (Machain-Castillo et al., 2019; Kaiho, 1994, 1999). To determine Total Organic Carbon (TOC) in sediments, Pan et al. (2017) used a CHN-O elemental analyzer to determine carbon, hydrogen and nitrogen content, in addition to total sulfur (TS) measurements under an elemental sulfur/nitrogen/carbon analyzer. When there is no elemental analyzer, treatments with HCl and calcination in the oven are done (Menezes, 2011).
Some studies used Energy Dispersive X-ray Fluorescence (EDX) analyses (Millo et al., 2005) to determine qualitative estimates of trace metal contents. Other studies chose quantitative plasma mass spectrometry analyses inductively coupled (ICP-MS) as it is a highly sensitive method capable of determining almost all elements present (Pan et al., 2017). In this case Barium, Bromine, Titanium and Aluminum, in order to identify the biochemical processes, present in the sediment to track paleoproductivity (Ba), organic matter content (Br), availability of terrestrial clastic material (Ti) and decrease or increase of terrigenous elements.
3. Appropriation of a methodology for the Colombian Caribbean
The occurrence of methane hydrates in the Colombian Caribbean has been recorded from seismic reflections made by the Institute of Marine Sciences of the University of Texas (Shipley et al., 1979). From this finding, the theoretical calculation of the heat flow gradient was made (López & Ojeda, 2006). The most significant geomorphological features for the region are mounds representing the surface expression of submarine volcanoes associated with methane seeps, present in the NW of the Colombian Caribbean, identified from bathymetric models of the ocean floor (Ojeda et al., 2004). The leaks have been in several sectors of the continental shelf, which extends 70 km from the Gulf of Morrosquillo, whose thick Pleistocene cover experiences mud diapirism (Vernette et al., 1992). In turn, the morphology of the internal shelf of the Gulf of Morrosquillo presents a concave surface whose bathymetric records have indicated the presence of recent activity due to mud volcanoes and diapirism (Ojeda et al., 2007), indicating that the sector is influenced by gas and oil seeps (García-González, 1991).
Despite the recognition of the leaks in the platform in front of the Gulf of Morrosquillo, no studies have been done focused on benthic foraminifera. There are only studies with macrofauna extracted from 300-940m depth at methane seepage sites, west of the Magdalena and Sinú deltas (Gracia et al., 2012). That is why, within the framework of the project "Methane Seep Hunting: A Multi-scale and Multi Method Approach", a methodology is planned for the study of foraminifera associated with cold seeps in this Caribbean region. Based on this bibliographic review and with the available technological scope, the following protocol was established:(1) Identification of sampling sites from satellite, bathymetric, geophysical and sea floor imagery analyses with ROVs, selecting stations associated with typical seepage geoforms and control stations. (2) Collection of box and piston cores (3) In the box cores collect two subcores of diameters close to 8 cm. One for analysis of the surface 5 cm, using an extruder, and staining with rB for identification of living associations. Another for the determination of sedimentation rates with 210Pb and a detailed sampling of the surface 50 cm (every 1 cm). (4) In piston cores, use of gas chromatography for hydrocarbon characterization and X-ray diffraction analysis for sediment mineralogical composition. (5) In living and fossil foraminifera populations, calculate abundance, density, diversity indices, and isotopes of carbon and oxygen. (6) In sediments, make the measurement of granulometry, TOC and nitrogen, and dating of foraminifera shells with 14C. (7) Definition of the size of the fraction for the counting and classification of foraminifera according to their ontogenic development in the samples.
According to the methodological review at the global level, there are two limitations at the local level, one in relation to the collection of the core, and the other to laboratory analyses. As for the collection of the cores, there are no specialized ROVs that allow collecting thrust cores or defining exactly the place of the socket of the box or piston cores. Although the nucleators will be launched on top of the place of the geoforms, the exact place where they fall relative to them, it will not be possible to control. Laboratory limitation consists of technology for identifying diagenetic traits in foraminifera shells. Autogenous carbonate overgrowths will be identified with available stereoscopes, and their effect on chemical analyses would be attempted to be reduced with ultrasonic washes on ethanol.
4. Conclusions
The methodologies used for the study of foraminifera in current seeps depend on the type of filtration; of the local study area; the availability of tools and equipment for research, and the purpose of analyses for the extracted sediments.
The FOBIMO biomonitoring protocol is the reference method in the most recent filtration studies to perform the handling and treatment of surface samples.
Calculations of diversity and abundance, identification of diagenetic traits in shells, and geochemical analyses of foraminifera are the most widely used methods for both surface and subsurface samples. These methods allow to characterize the ecology of foraminifera, to have reliable signals of δ¹⁸O and δ¹³C in their shells and to identify the isotopic behavior of these in the filtration sites and control points.
Geochemistry of hydrocarbons and sediments, mineralogical composition, and AMS dating are analyses that are performed preferentially on subsurface samples.
In the Colombian Caribbean, the occurrence of seeps has been demonstrated, but there are no studies of the associated foraminifera. From this review, it was possible to establish a protocol to investigate them and identify the main limitations they have.