Sporotrichosis is a subcutaneous granulomatous mycosis with a subacute or chronic evolution course that afflicts humans and mammals worldwide and produces different clinical manifestations. The disorder is produced by dimorphic fungi included in the clinical clade of Sporothrix spp. 1.
In Latin America, sporotrichosis is the most prevalent subcutaneous mycosis. It regularly exhibits changes in its epidemiological patterns. Classically, sporotrichosis is acquired by traumatic inoculation of the fungus through contaminated materials, such as plants, thorns, or soil, penetrating the host’s subcutaneous tissues. Nonetheless, zoonotic transmission by domestic cats has been observed, thus changing the accepted transmission paradigm of this disease 2.
For over a century, Sporothrix schenckii was considered the only species responsible for all cases of sporotrichosis 3,4. However, in 2007, Marimon et al. 5 proposed to split S. schenckii into several molecular siblings by phylogenetic analysis of the calmodulin gene (exons 3-5). Sporothrix is a diverse genus with wide genetic and ecological diversity, reflected in the many different associations between organisms and their hosts or niches 6.
Currently, Sporothrix comprises 53 species divided into clinical and environmental clades. The clinical clade includes four species causing human and animal infections: S. brasiliensis, S. schenckii, S. globosa, and S. luriei. Most Sporothrix species belong to the environmental clade: S. pallida complex, S. stenoceras complex, S. candida complex, S. inflata complex, and S. gossypina complex; and feature lower pathogenic potential toward mammals. Environmental Sporothrix species are usually associated with decaying wood, plant debris, soil, insects, etc. However, some species of this clade have been reported as the causative agent of sporothricosis cases: S. pallida complex (S. chilensis, S. humicola, S. mexicana, S. pallida), and S. stenoceras complex 6,7.
Identification and differentiation of clinical clades species is important due to the variability of their virulence and geographic distribution. Additionally, their sensitivity to antifungals reveals different levels of resistance. These variations are also noticed in the frequency of the species causing the mycosis.
Sporotrichosis is the most common subcutaneous mycosis in Colombia, with a prevalence rate of 0.1 to 0.5% in one report 8 or eight cases per 100,000 patients in another 9. In Colombia, the epidemiology and microbiology information about the causative agents of sporotrichosis is unknown since the related studies were conducted in big cities, such as Medellin and Cali, and were focused merely on the disease epidemiology and clinical manifestations 10,11. In the Medical Mycology Laboratory at the Facultad de Medicina of the Universidad de Antioquia (Medellín), where we collected the present data, S. schenckii was considered the only causative agent of sporotrichosis, but the characteristics of the isolates were not explored. This prompted us to undertake a detailed phenotypical and genotypical characterization of those clinical isolates.
Materials and methods
Isolates
We characterized 42 isolates previously identified as Sporothrix spp. These isolates were preserved according to the following protocol.
Subcultures
The isolates were sub-cultured on Mycosel agar and incubated at room temperature for one month. Once we confirmed the isolates’ growth, some colonies were transferred to tubes containing distilled water for short-term storage at room temperature, and others, mineral oil and skim milk medium for -20°C storage, for further studies.
Phenotypic characterization
Macroscopic morphology. The growth range, pigmentation, and colony texture were assessed in 42 isolates. An inoculum of 5 x 105 conidia/ml in 10 μl was plated on potato dextrose agar and corn meal agar (CMA) per triplicate. The plates were incubated at 25, 30 and 37 °C. The growth diameter was measured in millimeters, longitudinally and transversely, using a ruler every 7, 14, 21, 28, and 40 days. During the same period, we evaluated qualitatively pigments, morphology, and texture of the grown colonies. All isolates were cultured in dark incubators.
Microscopic morphology. We evaluated the conidia produced by the mycelial form using microcultures. After ten days of incubation at 25 °C, we estimated morphology, diameter, and conidiation type in potato dextrose agar and corn meal agar. Ten photographs were taken for each isolate, with a 100fold magnification, using a Leica DM 500™ camera adapted to a Leica ICC 50W™ microscope. We employed the SE Micrometrics Premium 4 ACCU- SCOPE software to measure the area and the perimeter of 100 conidia (100 hyaline and 100 dematiaceous or pigmented) per isolate. This information was compiled in an Excel™ database for further statistical analysis.
Transition from mycelial to yeast form. Two enriched culture media induced the yeast morphology in each isolate: Brain-heart infusion (BHI, BBLTM; ref. 211065. Franklin Lakes, NJ, USA) supplemented with 2% glucose (Sigma, ref. G5400-250G) (BHI-I); and supplemented with 0.1% L-cysteine (Sigma, ref. C-7755. St. Louis, MO, USA), 5% sheep blood, and 2% glucosa (BHI-II). These media were incubated at 37°C with 5% CO2.
The complete transition from mycelium to yeast form was determined by conducting multiple cultures every eight days, until creamy colonies obtention. Confirmation was achieved through microscopic observation of blue lactophenol-stained cotton corresponding to the colonies, revealing oval structures analogous to blastoconidia and hyphae absence.
Carbohydrate assimilation. Carbohydrate assimilation was evaluated in all isolates in the yeast form using API AUX 20 (Biomerieux®, Marcy-l’Etoile- France) after confirmation of the complete transition from mycelium to yeast form, as mentioned. The protocol was modified by adding 200 μl of yeast suspension and reading at 8-10 days after seeding the wells. Turbid wells were considered positive compared to the negative control, lacking a source of carbohydrates. We evaluated the following carbohydrates: glucose, glycine, α-ketoglutarate, arabinose, xylose, adonitol, xylitol, galactose, inositol, sorbitol, methyl D-glucosidase, N-acetylglucosamine, cellobiose, lactose, maltose, sucrose, trehalose, melezitose and raffinose.
Genotypic characterization
DNA was extracted and purified from the yeast phase of all 40 isolates after three days of growth using the protocol recommended by Norgen Biotek Corporation for fungi/yeast genomic DNA (Thorold, ON, Canada). For the remaining two isolates, DNA was extracted from the mycelial phase using the phenol-chloroform and isoamyl alcohol method. The DNA was quantified using the NanoDrop™ One/OneC Microvolume UV-Vis spectrophotometer. A expected protein ratio (260/280) within the range of 1.8-2.0 was needed to ensure the absence of protein contamination.
Partial amplification of the calmodulin gene (CALM1) was performed from genomic DNA using conventional PCR with primers described by O'Donnell et al. 12: CL1 (5’-GAR TWC AAG GAG GCC TTC TC-3 ‘) and CL2A (5’ -TTT TTG CAT CAT GAG TTG GAC-3 ‘). These primers generate an 800 pb amplicon corresponding to exons 3 and 5 of this gene.
Phylogenetic analysis
The products were sent to Macrogen Inc. (Geumcheon-gu, Seoul, South Korea) for sequencing using the ABI 3730XL DNA sequencing technology with the quality criterion of QV20. The obtained sequences were edited using the Geneious, version 11.0.2, software. Partial calmodulin gene sequences from Sporothrix spp. isolates, reported in the GenBank, were also included to improve phylogenetic tree support. The IqTree, version 1.4.4, software was used to determine the evolutionary model and the relationships between the isolates. A maximum likelihood-based phylogenetic tree was built using the Kimura two-parameter models and Jukes Cantor with a 1,000 bootstrap.
Results
Phenotypic characterization
Growth ranges. The isolates’ growth was measured every seven days for a 40 day-period. The isolates showed similar growth ranges in the evaluated culture media (potato dextrose agar and corn meal agar). However, their growth differed at the tested temperatures, with colonies growing more at 25 °C than at 30 °C (figure 1A and 1B). Growth was not observed in none of the 28 isolates at 37 °C, regardless of the culture media used (data not shown). Among the 14 isolates that exhibited growth at 37 °C, the range was between 0.3 and 2.5 mm in potato dextrose agar, and between 0.2 and 1.9 mm in corn meal agar.
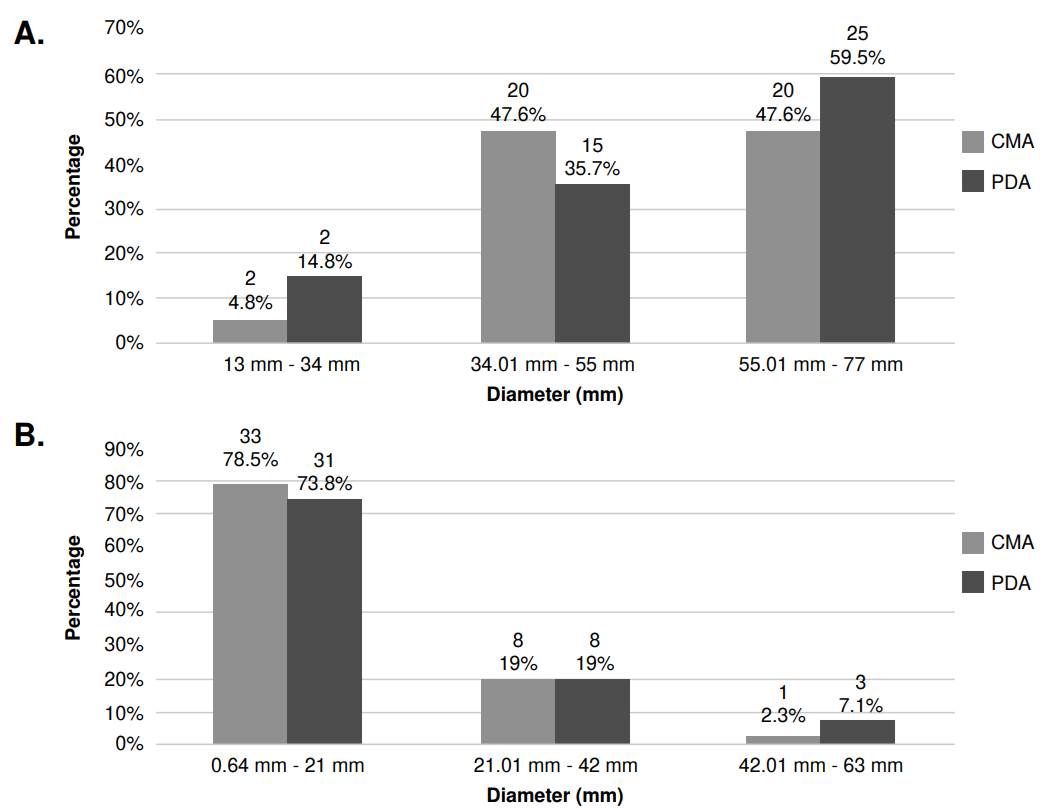
Figure 1 Growth range of growth of Sporothrix spp. clinical isolates in their mycelial phase. A. Comparison of growth diameter in potato dextrose agar (PDA) and corn meal gar (CMA) at 25 °C and after 40 days of observation. The number of isolates (n) are located at the top level of the numbers in percentages for each bar. B. Comparison of PDA and CMA growth diameter at 30°C at 40 days of observation. The number of isolates (n) are located at the top of each bar.
Colony textures of Sporothrix spp. clinical isolates
Macroscopic characteristics among the isolates were better defined in potato dextrose agar at 25 °C, while in the corn meal agar, all had a similar texture, not allowing differentiation among the isolates. Therefore, observations of the colony texture and pigment were made only in potato dextrose agar.
The texture of Sporothrix spp. clinical isolates exhibited a heterogeneous range of colonies. We found the membranous aspect prevailed among 50% of the isolates (n=21), but this trait was also present in 42,85% of others (n=37), sharing other textures such as velvety, central hairy, and cerebriform-like. A single texture, different from the membranous, was less frequent: centralhairy texture colonies corresponded to 2.38% (n=1) and with velvety texture to 2.38% (n=1). The cerebriform aspect shared textures with those previously described in 11.9% (n=5), but it was not observed as a separate texture. Figure 2-I illustrates previously described textures, and table 1 shows the frequencies of the observed different textures.
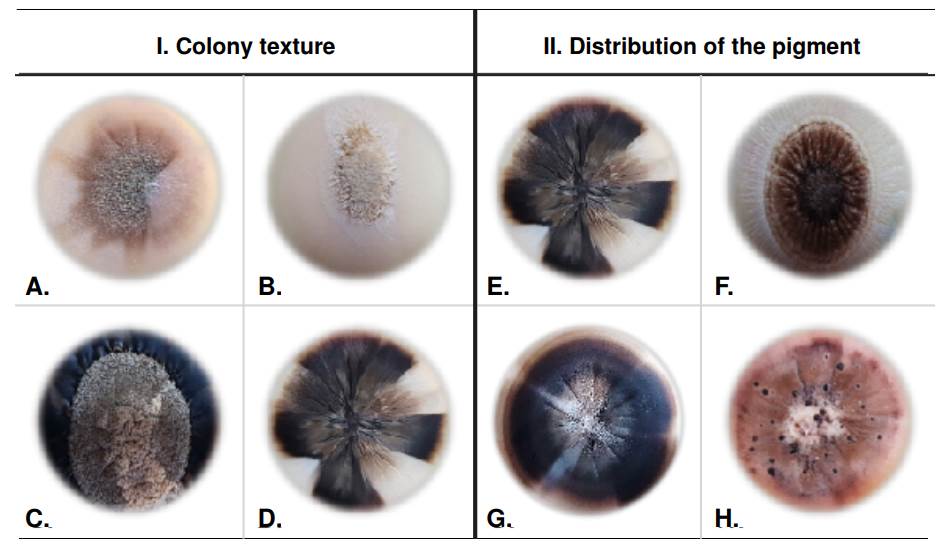
Figure 2 Phenotypic characteristics of Sporothrix spp. clinical isolates colonies. I. Texture of the Sporothrix spp. clinical isolates colonies after 40 days of observation: Velvety (A), central hairy (B), membranous (C), and cerebriform (D). II. Pigment distribution of the Sporothrix spp. clinical isolates colonies after 40 days of observation: Spotted heterogeneous (A), centralized heterogeneous (B), sectorized heterogeneous (C), and dotted heterogeneous (D).
Table 1 Texture of Sporothrix spp. isolates colonies in their mycelial phase after 40 days of culture in potato dextrose agar at 25 °C (N=42)
Texture | % | n |
---|---|---|
Membranous | 50 | 21 |
Membranous and central hairy | 19.05 | 8 |
Membranous and velvety | 9.52 | 4 |
Membranous and cerebriform | 2.38 | 1 |
Membranous, velvety and cerebriform | 2.38 | 1 |
Membranous, central hairy and velvety | 2.38 | 1 |
Membranous, central hairy, cerebriform and velvety | 2.38 | 1 |
Central hairy | 4.76 | 2 |
Velvety | 2.38 | 1 |
Central hairy and velvety | 2.38 | 1 |
Pigments produced by Sporothrix spp. clinical isolates at the mycelial phase
The isolates in their mycelial phase presented various pigment types after 40 days of growth in potato dextrose agar at 25 °C, even within the same isolate. The pigments observed included light types (beige, light brown) and dark types (dark brown, black). The pigments were associated with the size of the colonies: Smaller-sized isolates (22-35 mm) tended to develop dark pigments; medium-size (35.1-58 mm) and biggersize colonies (58.1-76mm) did not show a tendency toward any pigment tone and exhibited pigments ranging from light to dark tonalities.
Distribution of pigment in the mycelial colonies was registered as follows: Homogeneous 33% (n=14), spotted heterogenous 33% (n=14), sectorized heterogenous 26% (n=11), centralized heterogenous 5% (n=2), and dotted heterogenous 3% (n=1). We also studied pigments tonality and distribution in the mycelial colonies. Light pigments exhibited homogenous distribution in 19% (n=8), while dark had a homogenous distribution in 14.2% (n=6) of the isolates. Spotted heterogenous distribution was observed in 11.9% (n=5) of the isolates, and sectorized heterogenous distribution in 2.3% (n=1) of the total.
In some isolates (n=22) we observed a mixture of light and dark pigments and, among this group, sectorized heterogenous distribution in 23.8% (n=10) and spotted heterogenous distribution in 21.42% (n=9) of the isolates. Lower frequencies were obtained for centralized heterogenous and dotted heterogenous distributions, occurring in 4.76% (n=2) and 2.38% (n=1) of the isolates, respectively. Examples of colony texture are shown in figure 2-II.
Sporothrix spp. conidia and their microscopic morphology in clinical isolates
The observed hyaline conidia had an average area of 4.1 μm2 and perimeter of 8.7 pm. The elongated oval shape predominated in this type of hyaline conidia and was present in all isolates (100%). The elongated-oval form was common and identified in 70% of the isolates. The globose and subglobose forms were present in 65% of these conidia (figure 3). Most of the hyaline conidia were organized in a flower shape around a vesicle, a form characteristically described for Sporothrix spp. The conidia were produced in a sympodial on denticulate conidiogenous cells.
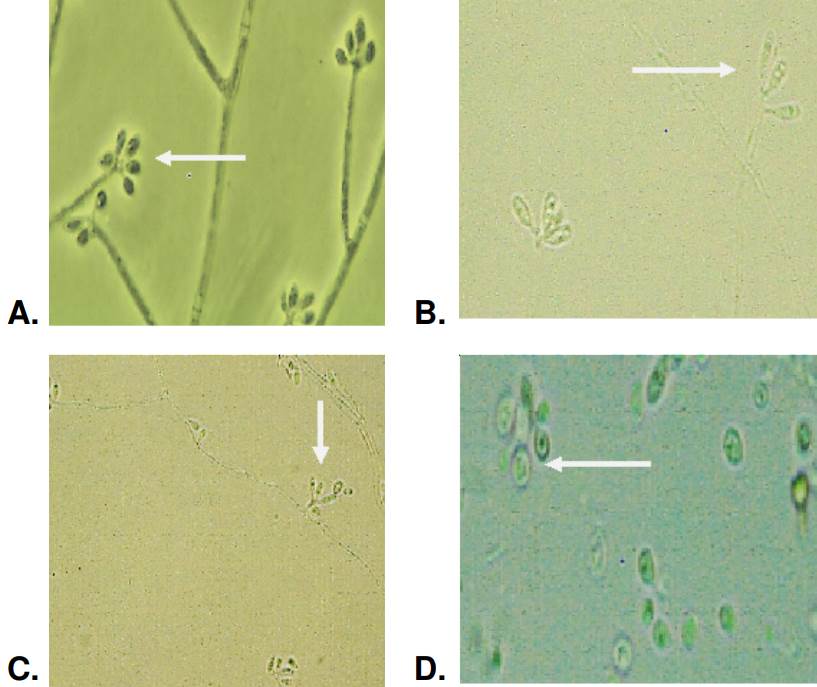
Figure 3 Morphology of hyaline conidia produced by Sporothrix spp. clinical isolates and observed at 100-fold magnification: Oval conidia arranged in flower form (A), oval elongated conidia (B) at 40-fold magnification (C), and globose conidia (D).
It was not possible to obtain sporulation in one isolate, so the morphology, as described below, was evaluated only in 41. The dematiaceous or pigmented conidia had an average area of 5.6 μm2 and a perimeter of 9.6 μm. In these dematiaceous conidia, the globose and subglobose forms predominated in 27 (65%) isolates out of the evaluated 41. Acropleurogenic sporulation was observed in this conidia form. The presence of oval- dematiaceous conidia organized in a flower-like arrangement was identified in 60% (n=25) of the isolates. In 30% (n=13) of the isolates, we found triangular conidia, primarily organized side by side on the hyphae as sessile acropleurogenic conidia (figure 4).
Sporothrix spp. clinical isolates: Patterns of carbohydrate assimilation
The evaluation of carbohydrates assimilation, determined by the API 20 test in the 42 isolates, showed that all isolates were capable of assimilating glucose, glycine, xylose, sorbitol, N-acetylglucosamine, maltose, sucrose and trehalose. However, important differences were found in the assimilation of the carbohydrates raffinose (40%), α-ketoglutarate (38%), and inositol (35%).
Sporothrix spp. clinical isolates: Transition from mycelium to yeast form
The transition from the mycelium to the yeast form was achieved by culturing each isolate four times in fresh culture media, with intervals of seven days. The yeast forms were obtained using two different culture media: BHI-I supplemented with 2% glucose, resulting in the yeast phase for 37 out of 42 isolates (88%), and BHI-II supplemented with 0.1% L-cysteine, 5% blood, and 2% glucose, which allowed all isolates (100%) to change to transit to the yeast form.
Phylogenetic analysis of Sporothrix spp. clinical isolates
Isolate genotyping was performed by partial amplification of the calmodulin gene. The alignment included 40 clinical isolates from the Grupo de Micología Médica, Departamento de Microbiología y Parasitología, Facultad de Medicina, Universidad de Antioquia and 162 sequences from the Genbank database, representing pathogenic Sporothrix species such as S. brasiliensis, S. schenckii, S. globosa, S. mexicana, and S. chilensis, as well as environmental species like S. brunneviolacea, S. pallida S. variecibatus, and S. lignivorus, (used as an outgroup).
Out of the sequenced 42 isolates, 32 were identified as S. schenckii, and eight isolates were identified as S. globose (figure 5). Two isolates were identified as Sarocladium kiliense by BLAST analysis. However, these sequences were excluded from the alignments as they were determined to be laboratory contaminants. All sequences were analyzed by BLAST and showed identification ranging from 99 to 100%. The sequences were deposited in the Genbank.
Phenotypic differentiation by species
The molecular identification of the clinical isolates and the differences observed in their phenotypic characteristics, including growth at different temperatures, colony texture and pigment, and conidia microscopic morphology, allowed for the grouping shown in table 2.
Table 2 Characterization of Sporothrix spp. clinical isolates: Molecular identification and phenotypic findings
Specie | Growth 25°C | Growth 30°C | Growth 37°C | Colonies PDA 25°C | Microscopic morphology | ||
---|---|---|---|---|---|---|---|
PDA (mm) | CMA (mm) | PDA (mm) | CMA (mm) | PDA-CMA (mm) | |||
S. schenkii | 57.4±8.3 | 59.63±8.3 | 18.32±12.3 | 15.3±2.3 | Yes | Membranous | Triangular, oval elongated |
S. globosa | 44.7±11.5 | 41.3±14.5 | 9.1±10 | 7.5±7.9 | No | Velvety | Globoses |
Discussion
The main finding of the present study was the successful specieslevel characterization of 40 Sporothrix spp. clinical isolates obtained from patients with sporotrichosis in the department of Antioquia, Colombia. Partial sequencing of the calmodulin gene revealed 32 isolates identified as S. schenckii, while the remaining eight corresponded to S. globosa.
The S. schenckii isolates demonstrated a heterogeneous group based on all evaluated phenotypic parameters. We observed that mycelial colonies of S. schenckii isolates grown at 25 °C were larger regardless of the culture media used, while at 30 °C, their growth tended to decrease. At 37 °C, the colony size was even smaller in both culture media.
In contrast, S. globosa isolates exhibited small mycelial colonies when grown at 25 °C. At 30 °C, in both culture media, the colonies were much smaller than the S. schenckii isolates in our study. Sporothrix globosa isolates were unable to grow in the nutritional media at 37 °C, consistent with previous phenotypic studies 5 reporting their incapacity to grow at this temperature with the employed culture media. Consequently, the transition from mycelial to yeast form at 37 °C was achieved in 100% of the isolates cultured with supplemented BHI-II medium and in 88% of the cases in non-supplemented BHI-I medium.
These findings suggest that supplemented BHI-II medium, also used for the transition of Histoplasma capsulatum13, could be recommended for studying the yeast form of other dimorphic fungi. In our study, BHI-II medium allowed the yeast form to grow in all tested Sporothrix spp. Clinical isolates. In contrast, in 2010, de Oliveira et al. 14 reported S. globosa growth in potato dextrose agar at 37 °C, suggesting significant phenotypic variability likely associated with genetic variability. This variability could indicate varying expression of S. globosa phenotypic characteristics according to the geographical origin 5,6.
Overall, our study provides valuable insights into the characterization of Sporothrix spp. species at 25 °C, as their growth characteristics had not been previously evaluated at this temperature in other phenotypic studies conducted with this fungus 5.
Our data differ from results obtained in other studies incubating the S. schenckii complex species at 30 °C in potato dextrose agar and corn meal agar 5. In our study, we observed smaller colony sizes at this temperature than those reported by Rodrigues et al. in 2013 15 and Camacho et al. in 2015 16. They described colony size ranges above 50 mm for S. schenckii and greater than 30 mm for S. globosa colonies. In 2007, Marimon et al. 5 reported colony sizes ranging from 33 to 36 mm for S. schenckii and from 18 to 40 mm for S. globosa, also higher than the sizes observed in our study.
In 2002, another study by Mesa-Arango et al. 17 analyzed the growth of Colombian isolates from different geographical origins at temperatures of 35 °C and 37 °C. Although these temperatures were different from those evaluated in our study, the findings were similar. The Colombian isolates exhibited lower thermotolerance with a higher percentage of inhibition at these temperatures compared to isolates from other countries (Mexico and Guatemala), which showed statistically significant differences among them. Based on these observations, we can conclude that the growth of Colombian Sporothrix spp. isolates is affected at temperatures above 30 °C.
In this study, we evaluated qualitatively the production and distribution of pigments in all isolates. In half of the S. globosa isolates (n=4), we detected mixed pigments (light and dark) with heterogeneous distributions, including spotting, centralized, distributed by sectors, and stippled patterns. Conversely, in the remaining S. globosa isolates, the distribution was homogeneous, with dark pigments predominance (n=3) compared to the light pigments (n=1).
Among the isolates identified as S. schenckii, we observed pigment diversity, including light, mixed, and dark pigments with the mentioned distributions, except for the stippling pattern, found exclusively in S. globosa isolates. Previous studies evaluating the phenotypic characteristics of Sporothrix spp. have not described the pigment type produced by these fungi. Chemical methods should be implemented to enhance these findings and to identify and quantify produced pigments by Sporothrix species.
Regarding pigment assessment, our analysis revealed significant diversity in the colony texture among the evaluated clinical isolates with a predominant membranous aspect. In the isolates identified as S. globosa, the dominant texture was velvety-like when grown on potato dextrose agar at 25 °C. Seven of these isolates exhibited this characteristic, along with the cerebriform and central-hairy aspects. Additionally, one of the two isolates that presented a mixture of all four textures, belonged to this species. The isolates identified as S. schenckii had a predominant membranous, followed by combinations of central, velvety, and cerebriform hairy textures. Similarly, in S. globosa one isolate presented all four textures.
This study does not allow us to specify a characteristic aspect for each species, but similarly to other works, it shows that colonies of the Sporothrix species tend to have a predominant membranous texture 18. The variation in macroscopic characteristics of the isolates raises suspicion about the possible existence of another species distinct from S. schenckii. In the case of the clinical isolates analyzed in this study, our concerns were confirmed with the finding and identification of S. globosa. We conducted phenotypic evaluations in potato dextrose agar medium because in corn meal agar all isolates exhibited similar pigment and texture. Cerebriform or centrally hairy colony appearance is not reported because its expression is probably affected by isolates’ multiple in vitro culture passages over the years, causing variability from its original form.
The microscopic morphological findings of this study align with those reported by Marimon et al. in 2007 5 and Camacho et al. in 2015 16. Conidial morphology descriptions in S. schenckii isolates revealed triangularshaped conidia, also known as sessile conidia. All isolates presenting this morphology belong to this species. On the other hand, none of the S. globosa isolates formed this type of conidia. They had predominance of globose (round) and sub-globose conidia.
Another significant difference observed in our study is the presence of elongated-to-oval conidia in 28 isolates of S. schenckii allocated in IIa clade according to the molecular biology, contrasting with Marimon et al. findings: Only two isolates belonging to IIb clade produced that type of conidia 5. Regarding the conidia diameter, we found differences between the two species. In S. schenckii, the average area and perimeter of dematiaceous and hyaline conidia were higher compared to S. globosa conidia from isolates. These findings align with those presented by Marimon et al. in 2007 concerning the area, although they did not report the perimeter 5. This study has enriched the knowledge of the microscopic aspects of prevalent species within the Sporothrix spp. clinical clade, allowing their characterization and differentiation.
Regarding carbohydrate assimilation, the carbohydrates commonly used to differentiate between Sporothrix species are raffinose, sucrose, and adonitol or ribitol 5. Previous studies reported that S. globosa does not assimilate raffinose 5, a finding also observed in our study for S. globosa and several S. schenckii isolates. Therefore, the assimilation of this carbohydrate does not allow for discrimination between the species. We observed differences in the assimilation of aketoglutarate and inositol, but this does not enable the distinction between the two species. The evaluation of carbohydrate assimilation using the Auxonograma API AUX 20 for species of Sporothrix spp. has not been reported before. The reason for evaluating the assimilation of these carbohydrates by clinical isolates of Sporothrix spp. by this method, was the inability to obtain adonitol by any means.
The S. schenckii complex has been divided into species by clades as follows: Clade I, S. brasiliensis; clade II, S. schenckii; clade III, S. globose; clade IV, S. mexicana; and clade V, S. albicans/pallida5. All of our isolates identified as S. schenckii were grouped in clade IIa, a subgroup limited to isolates from South American endemic areas, consistent with reports by Mesa-Arango et al. in 2002 17, Marimon et al. in 2007 5, Zhang et al. in 2015 18, Madrid et al. in 2009 19, and Rodrigues et al. in 2013 15. These reports described the prevalence of S. globosa in Colombian isolates, demonstrating the widespread distribution of this species.
The phylogenetic analysis of this study, based on the partial amplification of the calmodulin gene, resulted in a topology tree like those built by the mentioned authors. This similarity demonstrates the importance of utilizing the CALM1 gene for speciation within the clinical clade of sporotrichosis 15,20,21. The calmodulin gene has been widely used due to its synonymous substitutions in protein-coding regions, indicating a high degree of conservation and preservation of its original function. However, it contains parsimonious informative sites throughout its sequence, allowing for differentiation between species. This characteristic has led to a large number of available sequences of this gene in public databases and facilitated the development of rapid conventional PCR diagnostic tests using specific primers to identify the causative species of this mycosis 22,23.
The primary objective of this investigation was to phenotypically and genotypically characterize clinical isolates, previously identified as S. schenckii and S. globosa, to differentiate between the two species.
The results of this study indicate that the Colombian clinical isolates identified as S. schenckii exhibit a high degree of phenotypic heterogeneity. However, we could not establish a definitive pattern to differentiate between isolates corresponding to S. schenckii and S. globosa. Furthermore, Colombian isolates do not possess a higher genetic variability than isolates of S. schenckii from other regions of the world. Based on the findings of this research, we concluded that phenotypic characteristics assist in defining the genus and identifying major differences among Sporothrix species, but they do not provide a precise species identification. Confirmation of exact species would require the use of molecular biology techniques.